Chapter 14 Lipids, lipoproteins and cardiovascular disease
Introduction
The major lipids present in the plasma are fatty acids, triglycerides, cholesterol and phospholipids. Other lipid-soluble substances, present in much smaller amounts but of considerable physiological importance, include steroid hormones and fat-soluble vitamins; these are discussed in Chapters 8 and 20, respectively.
Triglycerides, cholesterol and phospholipids
Because they are not water soluble, lipids are transported in the plasma in association with proteins. Albumin is the principal carrier of free fatty acids (FFAs); the other lipids circulate in complexes known as lipoproteins. These consist of a non-polar core of triglyceride and cholesteryl esters surrounded by a surface layer of phospholipids, cholesterol and proteins known as apolipoproteins (Fig. 14.1). The latter are important both structurally and in the metabolism of lipoproteins (Fig. 14.2).
Classification of lipoproteins
Lipoproteins are classified on the basis of their densities as demonstrated by their ultracentrifugal separation. Density increases from chylomicrons (CM, of lowest density) through lipoproteins of very low density (VLDL), intermediate density (IDL) and low density (LDL), to high density lipoproteins (HDL). HDL can be separated, on the basis of density, into two metabolically distinct subtypes: HDL2 (density 1.064–1.125) and HDL3 (density 1.126–1.21). Distinct subtypes of LDL (LDL-I, II and III, in increasing order of density) are also recognized. IDL are normally present in the bloodstream in only small amounts but can accumulate in pathological disturbances of lipoprotein metabolism. This classification is illustrated in Figure 14.3 and the approximate lipid and apolipoprotein content in Figure 14.4. However, it is important to appreciate that the composition of the circulating lipoproteins is not static. They are in a dynamic state with continuous exchange of components between the various types. Their principal functions are summarized in Figure 14.3 and discussed in greater detail in the next section.
Lipoprotein metabolism
Chylomicrons
Chylomicrons (Fig. 14.5) are formed from dietary fat (principally triglycerides, but also cholesterol) in enterocytes; they enter the lymphatics and reach the systemic circulation via the thoracic duct. Chylomicrons are the major transport form of exogenous (dietary) fat. Triglycerides constitute about 90% of the lipid. Triglycerides are removed from chylomicrons by the action of the enzyme lipoprotein lipase (LPL), located on the luminal surface of the capillary endothelium of adipose tissue, skeletal and cardiac muscle and lactating breast, with the result that free fatty acids are delivered to these tissues to be used either as energy substrates or, after re-esterification to triglyceride, for energy storage. LPL is activated by apo C-II.
Very low density lipoproteins
VLDLs (Fig. 14.6) are formed from triglycerides synthesized in the liver either de novo or by re-esterification of free fatty acids. VLDL also contain some cholesterol, apo B, apo C and apo E; the apo E and some of the apo C is transferred from circulating HDL.
Low density lipoproteins
LDLs are the principal carriers of cholesterol, mainly in the form of cholesteryl esters. LDL are formed from VLDL via IDL (Fig. 14.6). LDL can pass through the junctions between capillary endothelial cells and attach to LDL receptors on cell membranes that recognize apo B-100. This is followed by internalization and lysosomal degradation with release of free cholesterol (Fig. 14.7). Cholesterol can also be synthesized in these tissues, but the rate-limiting enzyme, HMG-CoA reductase (hydroxymethylglutaryl CoA reductase), is inhibited by cholesterol, with the result that, in the average adult, cholesterol synthesis in peripheral cells probably does not occur. Free cholesterol also stimulates its own esterification to cholesteryl ester by stimulating the enzyme acyl CoA: cholesterol acyl transferase (ACAT).
High density lipoproteins
HDLs (Fig. 14.8) are synthesized primarily in the liver and, to a lesser extent, in small intestinal cells, as a precursor (‘nascent HDL’) comprising phospholipid, cholesterol, apo E and apo A. Uptake of cholesterol is stimulated by ATP-binding cassette protein A1 (ABCA1). Nascent HDL is disc shaped; in the circulation, it acquires apo C and apo A from other lipoproteins and from extrahepatic tissues, and in doing so assumes a spherical conformation. The free cholesterol is esterified by the enzyme lecithin-cholesterol acyltransferase (LCAT), which is present in nascent HDL and activated by its cofactor, apo A-I. This increases the density of the HDL particles, which are thus converted from HDL3 to HDL2.
The essential features of lipoprotein metabolism are as follows:
• dietary triglycerides are transported in chylomicrons to tissues where they can be used as an energy source or stored
• endogenous triglycerides, synthesized in the liver, are transported in VLDL and are also available to tissues as an energy source or for storage
• cholesterol synthesized in the liver is transported to tissues in LDL, derived from VLDL; dietary cholesterol reaches the liver in chylomicron remnants
• HDL acquire cholesterol from peripheral cells and other lipoproteins and this is esterified by LCAT. Cholesteryl esters are transferred to remnant particles, which are taken up by the liver, whence the cholesterol is excreted.
Reference ranges and laboratory investigations
At birth, the plasma cholesterol concentration is very low (total cholesterol less than 2.6 mmol/L, LDL cholesterol less than 1.0 mmol/L). There is a rapid increase in concentration in the first year of life; the mean value in childhood is approximately 4.2 mmol/L. In affluent societies particularly, concentrations rise further in early adulthood. Elevated plasma cholesterol concentrations are a major risk factor for CHD. The relationship between cholesterol concentration and CHD mortality is curvilinear (Fig. 14.9). The curve becomes increasingly steep as cholesterol concentration increases: CHD mortality doubles between concentrations of 5.2 and 6.5 mmol/L, and quadruples between 5.2 and 7.8 mmol/ L. Approximately two-thirds of adults in the UK have a plasma cholesterol concentration >5.2 mmol/L and one-quarter have >6.5 mmol/L. While at a concentration <5.2 mmol/L, the curve becomes shallow, it does not become flat. In individuals with other risk factors, for example cigarette smoking (see below), the curve is moved upwards and is steeper.
While there is an undoubted association between plasma cholesterol concentration (and, in particular, LDL cholesterol) and an increased risk of CHD, there is an inverse correlation between HDL cholesterol and CHD risk. Many physiological factors influence LDL and HDL cholesterol, some of which are indicated in Figure 14.10.
Separation of lipoproteins by ultracentrifugation is not a convenient technique for routine use and is primarily a research tool. Formerly, separation of lipoproteins by electrophoresis was widely used, but this only provides qualitative information and is now obsolete. Genotyping, or phenotyping, of apo E is required to confirm the diagnosis of remnant hyperlipidaemia (see p. 250). Assays for lipoprotein lipase and apo C-II are required for the diagnosis of the cause of fasting chylomicronaemia. Assays for other apoproteins are available and may prove useful in the future (e.g. apo B in place of LDL or non-HDL cholesterol, apo A-I in place of HDL), but so far there is insufficient evidence to support their use in diagnosis and management.
Selection of patients for investigation
It is therefore clear that lipid measurements should be made in all patients known to have vascular disease, and in those at increased risk (see p. 252). Thus plasma lipids should be measured in individuals with the following:
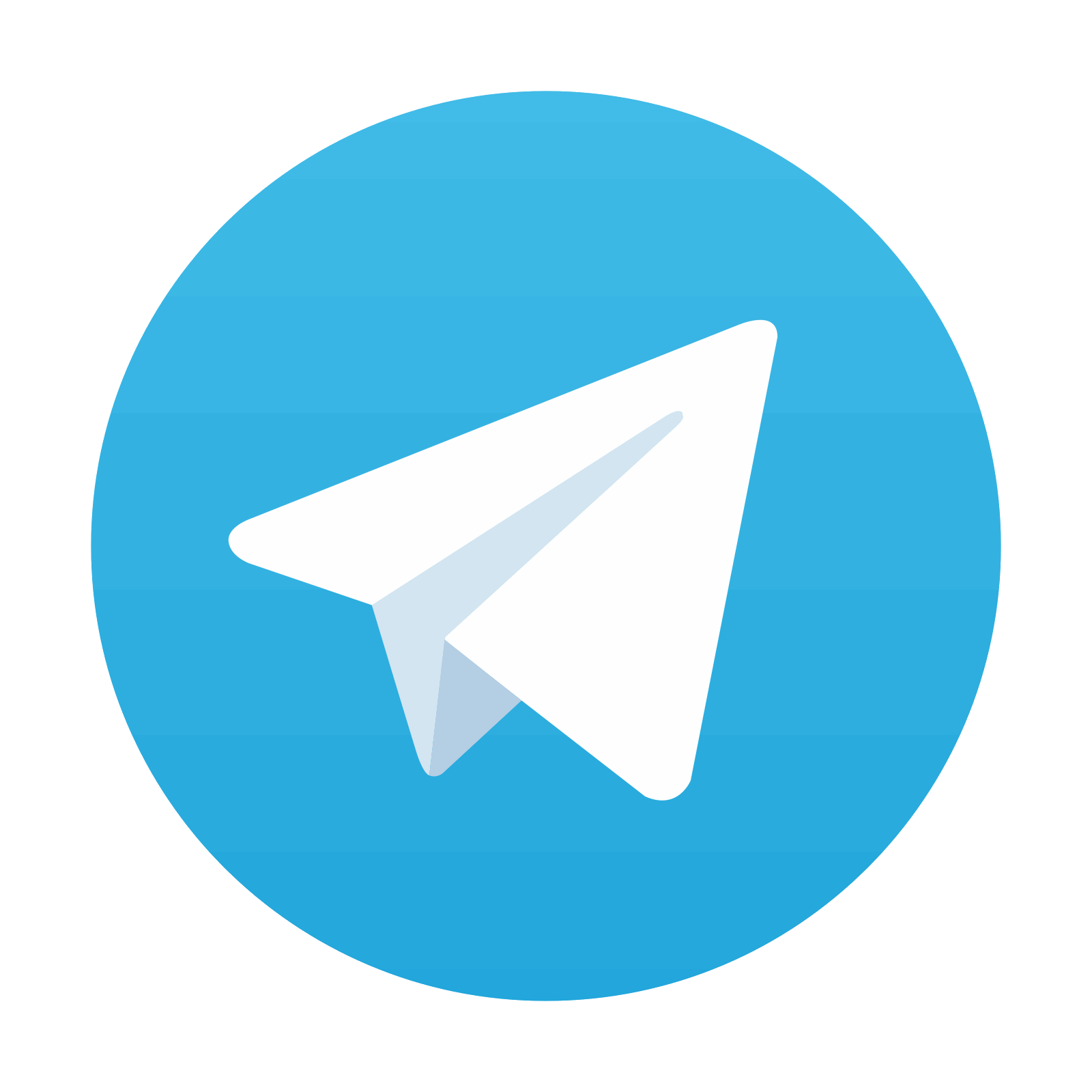
Stay updated, free articles. Join our Telegram channel

Full access? Get Clinical Tree
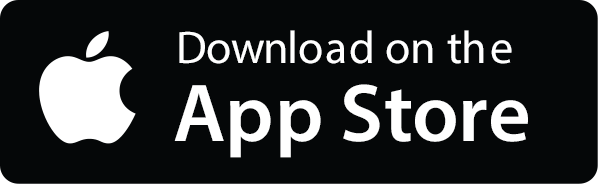
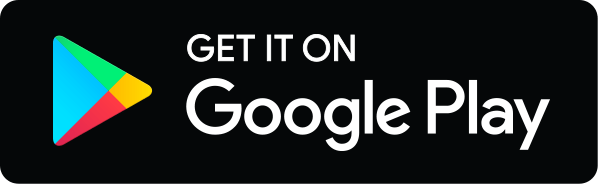