Chapter 15 The locomotor and nervous systems
Metabolic Bone Diseases
Bone has three important functions: it provides structural support to the body, it houses the haemopoietic bone marrow, and it is metabolically active, being essential for calcium and phosphate homoeostasis (see Chapter 12). Bone consists of a proteinaceous matrix, 90% of which is formed by type I collagen fibres, the remainder being glycoprotein and proteoglycan ground substance. This provides the support for the mineral component, which consists of spindle-shaped crystals of hydroxyapatite, a complex hydrated calcium phosphate. Bone has two structurally distinct components: dense, cortical bone (the major form) and spongy trabecular bone, which supports the bone marrow.
Mature bone undergoes constant remodelling, a process that involves up to approximately 10% of the skeleton at any one time. This process involves the resorption of small volumes of bone by osteoclasts (which subsequently undergo apoptosis) and their replacement by osteoid generated by osteoblasts (Fig. 15.1), which rapidly becomes mineralized to form mature bone. Some of the osteoblasts become trapped in the new bone and are transformed into osteocytes. The whole process is coordinated by numerous hormones, growth factors and cytokines, such that under normal circumstances the rates of resorption and bone formation are matched.
Metabolic bone diseases are a result of a disruption of this normally orderly process. The corresponding changes in plasma calcium and phosphate concentrations and alkaline phosphatase activity (a marker of osteoblastic activity) are summarized in Figure 15.2. Alkaline phosphatase promotes bone mineralization by breaking down pyrophosphate (an inhibitor of mineralization) to phosphate (which is required for the process).
Osteoporosis
These types of osteoporosis are classified as primary. Osteoporosis can also occur secondarily to a variety of conditions (Fig. 15.3), often in younger people. These conditions can also exacerbate primary osteoporosis.
Paget’s disease of bone
Case history 15.1
Renal osteodystrophy
Patients with chronic kidney disease develop a complex bone disease, primarily as a result of decreased renal synthesis of calcitriol. This condition is discussed in detail in Chapter 4.
Hyperparathyroid bone disease
Clinical evidence of bone involvement used to be a common feature of primary hyperparathyroidism (see Chapter 12), but most patients with hyperparathyroidism are now identified when hypercalcaemia is found incidentally. They are usually asymptomatic and have no abnormal physical signs or radiographic findings. Plasma alkaline phosphatase activity is normal unless there is significant bone involvement.
Articular Disease
Joints can be affected by a wide variety of diseases, both specifically and as part of multi-system disease. Osteoarthritis, rheumatoid arthritis and other inflammatory arthritides are a major source of pain and disability. For most, the role of the biochemical laboratory in management is limited: one example is the measurement of C-reactive protein (see p. 227) to monitor inflammatory conditions. For one group, however, biochemical tests are important in both diagnosis and management: these are the crystalline arthritides, in particular, gout.
Gout and hyperuricaemia
Introduction
Clinical gout is the result of the deposition of crystals of monosodium urate in the cartilage, synovium and synovial fluid of joints as a consequence of hyperuricaemia. It is the commonest inflammatory joint disease in men over the age of 40. Uric acid is the end product of purine metabolism in humans. At physiological pH, uric acid is 98% ionized and is therefore present mainly as the urate ion. In the extracellular fluid (ECF), where sodium is the major cation, uric acid effectively exists as a solution of its sodium salt, monosodium urate. This salt has low solubility, and the ECF becomes saturated at concentrations a little above those that normally prevail. In patients with hyperuricaemia, there is thus a tendency for crystals of monosodium urate to form. In addition to acute arthropathy, other manifestations of gout include renal calculi (which may lead to chronic kidney disease) and tophi (accretions of sodium urate in soft tissues). A sudden increase in urate production, typically seen as a consequence of treatment of haematological malignancy (tumour lysis syndrome, see p. 302), can lead to widespread crystallization in the renal tubules, causing obstruction and acute kidney injury (acute urate nephropathy). This is usually avoidable by giving allopurinol (see below), but if it does occur it can be treated with rasburicase, a recombinant fungus-derived uricase.
Uric acid metabolism
Purine nucleotides are essential components of nucleic acids: they are intimately involved in energy transformation and phosphorylation reactions and act as intracellular messengers. There are three sources of purines in humans: the diet, degradation of endogenous nucleotides and de novo synthesis (Fig. 15.5). As purines are metabolized to uric acid, the body urate pool (and hence plasma concentration) depends on the relative rates of both urate formation from these sources and urate excretion. Urate is excreted by both the kidneys and the gut, renal excretion accounting for approximately two-thirds of the total. Urate secreted into the gut is metabolized to carbon dioxide and ammonia by bacterial action (uricolysis).
Urate handling by the kidney is complex (Fig. 15.6). It is filtered at the glomeruli and almost totally reabsorbed in the proximal convoluted tubules; distally, both secretion and reabsorption occur. Normal urate clearance is about 10% of the filtered load. In normal subjects, urate excretion increases if the filtered load is increased. In chronic kidney disease, the plasma concentration rises only when the glomerular filtration rate falls below about 20 mL/min.
The metabolic pathways involved in uric acid synthesis are shown in outline in Figure 15.7. De novo synthesis leads to the formation of inosine monophosphate (IMP), which can be converted to the nucleotides adenosine monophosphate (AMP) and guanosine monophosphate (GMP). Nucleotide degradation involves the formation of the corresponding nucleosides (inosine, adenosine and guanosine); these are then metabolized to purines. The purine derived from IMP is hypoxanthine, which is converted by the enzyme xanthine oxidase first to xanthine and then to uric acid. Guanine can be metabolized to xanthine (and so to uric acid) directly, but adenine cannot. However, AMP can be converted to IMP by the enzyme AMP deaminase and, at the nucleoside level, adenosine can be converted to inosine. Thus, surplus GMP and AMP can be converted to uric acid and excreted.
Plasma urate concentrations are, in general, higher in men than in women. Marked increases occur at puberty in males (there is a lesser increase in females at this time) and perimenopausally in women (Fig. 15.8). Urate concentrations tend to be higher in people in the higher socio-economic groups and in the obese. There is considerable, genetically determined, variation in plasma urate concentrations between different ethnic groups, with particularly high concentrations, for example, in Polynesian and Maori people.
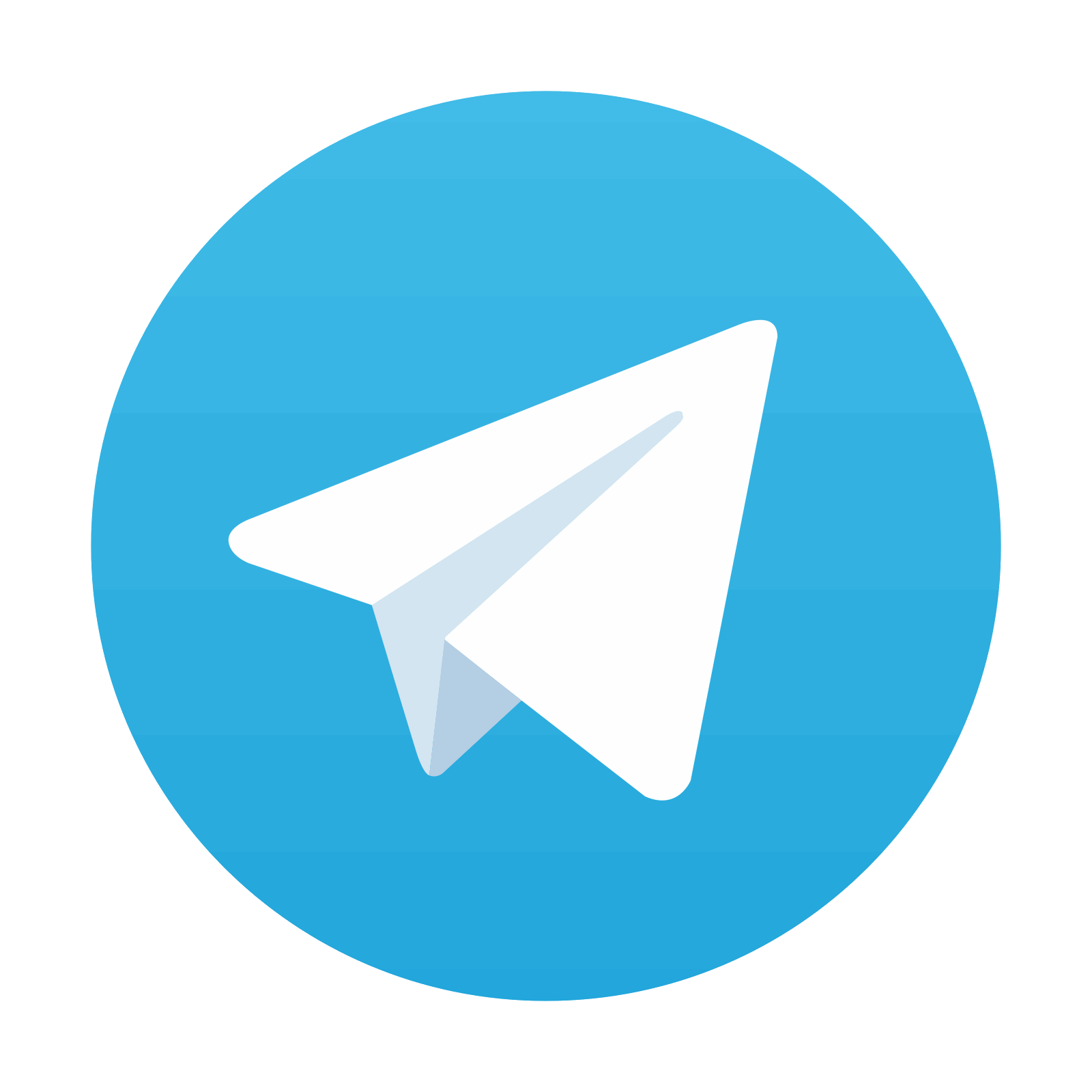
Stay updated, free articles. Join our Telegram channel

Full access? Get Clinical Tree
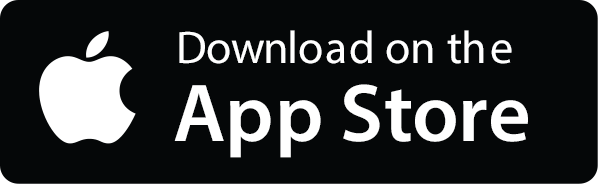
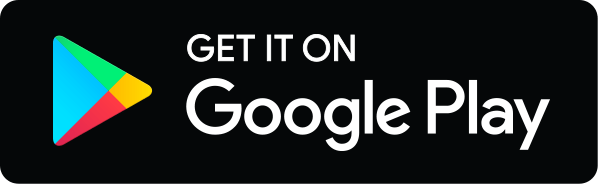