(1)
Canberra, Aust Capital Terr, Australia
Scientific Name
Styphnolobium japonicum (L.)Schott.
Synonyms
Anagyris chinensis Spreng., Macrotropis foetida DC., Ormosia esquirolii H. Lév., Pongamia chinensis DC., Robinia mitis Lour., Sophora angustifoliola Q.Q. Liu & H.Y. Ye, Sophora chinensis hort. ex Zabel, Sophora japonica L., Sophora japonica f. columnaris Schwer., Sophora japonica f. hybrida Carrière, Sophora japonica f. oligophylla Franch., Sophora japonica f. pendula Loudon, Sophora japonica var. praecox Schwer., Sophora japonica var. pubescens (Tausch) Bosse, Sophora japonica f. variegata Nichols, Sophora japonica var. vestita Rehder, Sophora japonica var. violacea Carrière, Sophora japonica var. violacea Dippel, Sophora korolkowii Dieck, Sophora mairei H. Lév., Sophora pendula Spach, Sophora pubescens Tausch, Sophora sinensis Forrest, Sophora vanioti H. Lév., Styphnolobium japonicum var. pubescens hort. ex Kirschner
Family
Fabaceae
Common/English Names
Chinese Scholar Tree, Japanese Pagoda Tree, Pagoda Tree, Scholar Tree, Umbrella Tree
Vernacular Names
Brazil: Sôfora Do Japão (Portuguese)
Chinese: Huái Hua, Huái Hua Mo, Huái Jiao, Huái Mi, Huái Shu
Czech: Jerlín Japonský
Danish: Pagodetræ
Eastonian: Jaapani Keepuu
French: Arbre Aux Pagodes, Arbre De Miel, Arbre Des Pagodes Du Japon, Sophora Du Japon
German: Japanischer Perlschnurbaum, Japanischer Schnurbaum, Pagodenbaum Sophore
Hungarian: Japánakác
Indonesia: Sari Cina
Italian: Sofora, Sofora Del Giappone
Japanese: Enju
Korean: Hoe-Wha-Na-Moo
Polish: Perełkowiec Japoński, Szupin Chiński, Szupin Japoński
Portuguese: Acácia-Do-Japão
Slovencina: Sofora Japonská
Spanish: Acacia Del Japón, Arbol De Las Pagodas, Sófora
Swedish: Pagodträd
Turkish: Japon Soforası
Vietnamese: Hoè
Origin/Distribution
The species is indigenous to East Asia—Central and Northern China and Korea. It is now widely cultivated in temperate and subtropical regions of the world and infrequently in the highlands in the tropics, e.g. in Southeast Asia, in Vietnam and Thailand.
Agroecology
A cool climate species, being native to the temperate and subtropical regions of Eastern Asia will withstand warm but not hot temperatures. It is frost tolerant down to –25 °C when mature except when young. It will grow in the cooler and drier areas of the highlands in the tropics as it is drought tolerant. The tree is found in wasteland and open country between 300 and 1,000 m altitude in its native range. The tree is well adapted to wide range of soil types including poor soils, but thrives best in well-drained, moderately fertile, sandy loams in full sun.
Edible Plant Parts and Uses
The young tender leaves, shoots and twigs, flowers and seeds are edible cooked (Uphof 1968; Read 1982; Kunkel 1984; Facciola 1990; Hu 2005). Leaves are cooked with rice and consumed (Uphof 1968; Read 1982). Shoots are sun-dried and boiled several times to remove the bitter elements before eating. The twigs are thoroughly boiled in water into which an egg is poached; the liquid is then drunk and the poached egg consumed as a home remedy for stopping haemorrhages (Hu 2005). Flowers and buds are gathered and used as an important ingredient in the traditional Five Flower Tea (‘Wu Hua Cha’) for the hot summer season in Hong Kong. The leaves can also be made into tea and an edible starch is obtained from the seed (Facciola 1990). The seed endosperm is cooked with sugar to prepare a dessert that is eaten in Northern China (Hu 2005).
Botany
A small to medium-sized, deciduous perennial tree, up to 5–15(−30) m tall with a short stout bole, rough, greyish-brown, fissured bark and upright, spreading branches and glabrous, terete, pale green, lenticillate twigs and broad round crown (Plates 1 and 2). Leaves are arranged spirally, imparipinnate, 15–25 cm long with caducous stipules; leaflets are subopposite and shortly petiolulate, 13–17, elliptical to ovate-lanceolate (Plates 2 and 5), 1.5–6 cm by 1–2.5 cm, acute to subacute apex, obtuse at the base, margin entire, sparsely pubescent below, bright green tuning to yellow in autumn. Flowers are bisexual, papilionaceous, mildly fragrant, small pentamerous, in terminal, 15–35 cm long upright panicles (Plates 3 and 4), bracteoles present, calyx campanulate, 3–4 mm long with 5-teeth, corolla 5 white or greenish-white petals, standard broad and cordate, 12–15 mm long with curled margins (Plate 5), stamens 10 free but filaments joined near the base, ovary superior and pilose. Fruit an indehiscent, terete leguminous pod, 3–12 cm by 7–12 mm, green turning to yellow when mature, constricted between the seeds, stipitate, glabrous, distinctly beaked, 2–5-seeded. Seeds ellipsoid to ovoid, 8 mm × 4–5 mm, slightly compressed, yellowish-brown.






Plate 1
Tree habit with a short stout bole (Nguyen VT)

Plate 2
Ascending slender branches and twigs

Plate 3
Large panicle with flower buds (Nguyen VT)

Plate 4
Panicle with some opened flowers (Nguyen VT)

Plate 5
Pinnate leaves and inflorescence with white flowers (Nguyen VT)
Nutritive/Medicinal Properties
Flower Phytochemicals
Blossoms were found to contain sophorin (A,B, C) (Brauns and Schmidt 1904; Vo 1997) and four to five times as much rutin as does buckwheat, the best domestic source (Couch et al. 1952). Rutin content declined as the bud opened and continued to fall as the seed pod formed. No rutin was found in the seed while traces occurred in the leaves. Sophoroflavonoside, kaempferol-3-rhamnodiglucoside, genistein-7-d-cellobioside, sophoricoside, sophorabioside, betulin and sophoradiol were isolated from the flower buds (Kariyone et al. 1956; Takahashi et al. 1960; Ishimasa 1960), flavonoids: quercetin, rutin and isorhamnetin-3-rutinoside, kaempferol-3-rutinoside (Kimura and Yamada 1984); and isorhamnetin 2-(3-methoxy-4-hydroxyphenyl)-3,5,7-trihydroxy-4H-1-benzopyran-4-one were isolated from the flower buds (Ishida et al. 1998). Three new glucuronide saponins, named kaikasaponin I, kaikasaponin II and kaikasaponin III, were isolated from buds of Sophora japonica together with five known glucuronide saponins soyasaponin I, soyasaponin III, azukisaponin I, azukisaponin II and azukisaponin V (Kitagawa et al. 1988). The structures of kaikasaponins I, II and III were elucidated as 3-O-[β-d-galactopyranosyl-(1 → 2)-β-d-glucuronopyranosyl]sophoradiol, 3-O-[α-l-rhamnopyranosyl-(l → 2)-β-d-glucopyranosyl-(l → 2)-β-d-glucuronopyranosyl]sophoradiol and 3-O-[α-l-rhamnopyranosyl-(1 → 2)-β-d-galactopyranosyl-(1 → 2)-β-d-glucuronopyranosyl]sophoradiol, respectively.
Accumulation of rutin started with initiation of the flower buds, reaching a maximum content of 287 mg/g (dry weight) during the earliest stages of flower development (Gevrenova et al. 2007). A further decline in the amount of rutin up to 44 mg/g was found proportionally with the decline of flavonoids throughout and after the flowering period. Rutin was the predominant glycoside and accounted for up to 90 % of flavonoid glycoside mixture in young buds, whereas sophorabioside (64.7 mg/g, calculated as rutin equivalent) and kaempferol 3-rutinoside (46.8 mg/g) dominated at later development stages of the fruits. Balbaa et al. (2009) reported that rutin reached its maximum in the flower buds (23 %). Maximum yield and efficiency of extraction of rutin from Sophora japonica flowers was improved by the application of ultrasound to methanol extraction but was dependent on the solvent employed (Paniwnyk et al. 2001). Rutin being a compound with antioxidant activity and aqueous solvents appeared to be unsuitable for ultrasonic extractions due to the formation of free radicals from the insonation of the solvent. The flower buds (Huai Mi) and flowers (Huai Hua) of S. japonicum (collectively Flos Sophorae) contained rutin as the main flavonoid and lacked the flavone glycosides that were present in flower buds and flowers of Sophora flavescens (Kite et al. 2009).
Fruit/Seed Phytochemicals
Sophorobioside, a glycoside, was isolated from the fruit (Farkas et al. 1968). Flavonoids isolated from the fruit included rutin, quercetin, genistein, kaempferol, sophorobioside, sophoricoside and sophoroflavonoloside (Tulaganov and Gaibnazarava 2001). Two kaempferol triglycosides: kaempferol 3-O-β-d-sophoroside-7-O-α-l -rhamnoside and kaempferol 3-O-(2″-O-β-d-glucosyl)-β-d-rutinoside (Tang et al. 2001a), four isoflavone triglycosides, genistein 7-O-β-d-glucopyranoside-4′-O-[(α-l-rhamnopyranosyl)-(1 → 2)-β-d-glucopyranoside], genistein 7-O-β-d-glucopyranoside-4′-O-[(β-d-glucopyranosyl)-(1 → 2)-β-d-glucopyranoside], genistein 7-O-α-l-rhamnopyranoside-4′-O-[(α-l-rhamnopyranosyl)-(1 → 2)-β-d-glucopyranoside] and genistein 7-O-α-l-rhamnopyranoside-4′-O-[(β-d-glucopyranosyl)-(1 → 2)-β-d-glucopyranoside], together with nine known compounds, namely, genistein 7-O-β-d-glucopyranoside-4′-O-β-d-glucopyranoside, sophorabioside, prunetin 4′-O-β-d-glucopyranoside, sophoricoside, genistin, rutin, kaempferol 3-O-β-rutinoside, quercetin 3-O-β-d-glucopyranoside and kaempferol 3-O-β-d-glucopyranoside (Tang et al. 2001b); new flavonol triglycoside, kaempferol 3-O-α-l-rhamnopyranosyl-(1 → 6)-β-d-glucopyranosyl-(1 → 2)-β-d-glucopyranoside (1), as well as two known kaempferol 3-O-[α-l-rhamnopyranosyl-(1 → 6)]-[β-d-glucopyranosyl-(1 → 2)]-β-d-glucopyranoside (2) and kaempferol 3-O-β-d-glucopyranosyl-(1 → 2)-β-d-glucopyranoside-7-O-α-l-rhamnopyranoside (3) (Tang et al. 2002b); a coumaronochromone derivative, sophorophenolone, along with 13 known compounds, l-maackiain, medicagol, 7-O-methylpseudobaptigenin, pseudobaptigenin, 7,3′-di-O-methylorobol, genistein, prunetin, daidzein, formononetin, di-O-methyldaidzein, quercetin, kaempferol and isorhamnetin (Tang et al. 2002a) were isolated from the fruit. Flavone compounds genistein-7,4′-di-O-β-d-glucoside (I), genistein-7-O-β-d-glucopyranoside-4′-O-[(α-l-rhamnopyranosyl)-(1 → 2)-β-d-glucopyranoside] (II), kaempferol-3-O-β-d-sophoroside(III), quercetin-3-O-β-l-ramnopyranosyl-(1 → 6)-β-d-glucopyranoside (IV), genistein-4′-β-l-rhamnopyransoyl-(1 → 2)-α-d-glucopyranoside (V) and kaempferol-3-O-β-l-ramnopyranosyl-(1 → 6)-β-d-glucopyranoside (VI) were purified from the fruit crude extract using high-speed countercurrent chromatography combined with macroporous resin column separation (Sun et al. 2007). The purities of compounds I, II, III, IV, V and VI were 98.7, 98.2, 97.8, 98.5, 99.3 and 98.9 %, respectively, as determined by HPLC. The flavonoids kaempferol glycosides—kaempferol 3-O-β-glucopyranosyl(1 → 2)-β-galactopyranoside-7-O-α-rhamnopyranoside and kaempferol 3-O-β-xylopyranosyl(1 → 3)-α-rhamnopyranosyl(1→6)[β-glucopyranosyl(1 → 2)]-β-glucopyranoside and two tetraglycosides: kaempferol 3-O-β-glucopyranosyl(1 → 2)[α-rhamnopyranosyl(1→6)]-β-glucopyranoside-7-O-α-rhamnopyranoside and kaempferol 3-O-β-glucopyranosyl(1 → 2)[α-rhamnopyranosyl(1 → 6)]-β-galactopyranoside-7-O-α-rhamnopyranoside—were identified in the fruit; the latter was the main flavonoid in mature seeds (Kite et al. 2009). Three flavonoids were isolated from the fruit: sophorabioside, genistin, quercetrin (Kim and Yun-Choi 2008). Flavonoids sophorabioside, sophora-flavanoloside and genistein glucoside appeared at the beginning of the fruit formation, while sophoricoside appeared only at the stage of its maturation (Balbaa et al. 2009). Rutin was found in the fruit reaching a minimum in the ripe fruits (4.3 %).
Phytoestrogens genistin, genistein, rutin, kaempferol and quercetin were determined in the fruit and seed of S. japonica by high-performance capillary electrophoresis with electrochemical detection (CE-ED) method (Chu et al. 2005). Detection limits ranged from 1.1 × 10−7 to 2.8 × 10−7 g/ml for all five analytes.
Seed Phytochemicals
Cytisine, N-methylcytisine, sophocarpine and matrine were isolated from the epigeal parts of the seed (Abdusalamov et al. 1972). Stizolamine were found in the seeds (Toshida and Hasegawa 1977). Triterpene glycosides: soyasapogenol B-3-[O-β-d-glucopyranosyl-(1 → 2)-O-β-d-glucopyranuronoside] (adzukisaponin II), soyasapogenol B [3-O-β-galactopyranosyl-(1 → 2)-O-β-l-glucopyranuronoside] (soyasaponin III), soyasapogenol B 3-(O-α-l-rhamnopyranosyl-(1 → 2)-O-β-l-glucopyranosyl-(1 → 2)-O-β-d-glucopyranuronoside) (adzukisaponin V), soyasapogenol B 3-(O-β-d-rhamnopyranosyl-(1 → 2)-O-β-d-galactopyranosyl-(1 → 2)-O-β-d-glucopyranuronoside (soyasaponin I) and the new glycoside (1)—soyasapogenol B 3-[O-β-d-glucopyranuronoside] were isolated from the seeds (Grishkovets and Gorbacheva 1995). Five triterpene glycosides with soyasapogenol B as the aglycone were isolated from the seeds (Gorbacheva et al. 1996). A new saponin 3-O-β-d-glucuronopyranosyl-soyasapogenol B was identified as s a minor component.
Six compounds were isolated from the seed alcohol extract and identified as follows: genistein 7-O-β-d-glucopyranoside-4′-O-β-d-glucopyranoside, genistein 7-O-β-d-glucopyranoside-4′-O-[α-l-rhamnopyranosyl-(1 → 2)-β-d-glucopyranoside], tectorigenin, tectoridin, 1, 6-di-O-galloyl-β-d-glucose and ethyl gallate (Wang et al. 2002). A flavonol tetraglycoside and kaempferol 3-O-α-l-rhamnopyranosyl(1 → 6)-β-d-glucopyranosyl(1 → 2)-β-d-glucopyranoside-7-O-α–l rhamnopyranoside, together with nine known compounds, were isolated from the seeds (Wang et al. 2003).
Total extractive from ground ripe S. japonica seeds consisted of neutral lipids, polar lipids, carbohydrates, amino acids, pigments, etc. (Mukhamedova and Glushenkova 1997). Total yield of neutral lipids was 7 %, phospholipids 1.1 % of seed weight and phosphorus content of the total neutral lipids was 3.3 %. Nine phospholipid classes were detected: lysophosphatidylcholines (lyso-PCs), phosphatidylcholines (PCs), phosphatidylinositols (PIs), phosphatidylethanolamines (PEs), N-acyl-phosphatidylethanolamines (N-acyl-PEs), N-acyl-lyso-phosphatidylethanolamines (N-acyl-lyso PEs), phosphatidic acids (PAs), phosphatidylglycerols (PGs) and d-phosphatidylglycerols (DPGs). A high level of 16:0 and 18:2 fatty acids were found in all phospholipid samples. In the (N-acyl-lyso PEs), 18:2 made up 51.7 % of total acid weight. In total phospholipid, trace amount of 21:0 fatty acids were found, mainly localized in the N-acyl-PEs. PGs contained a higher level of SFA (64.5 %) than other phospholipids, while N-acyl-lyso PEs contained the least (15.1 %) In sequence of decreasing unsaturation, the individual phospholipids were ranked as follows: N-acyl-lyso PEs → PCs → PEs → N-acyl-PEs → lyso-PCs → PIs → DPGs → PAs → PGs.
A haemagglutinin protein was purified from the seeds (Poretz et al. 1974). It was found to contain relatively large amounts of hydroxyl and acidic amino acids, no methionine and about five residues of half-cystine/mol. The presence of covalently bound carbohydrates was determined as 14–15 residues of 2-amino-2deoxyglucose and 5.9 % neutral saccharide of mannose and xylose. Seeds were found to contain N-acetylgalactosamine-specific lectins, glycoproteins with molecular weights of about 130,000 and possessing substantial sequence homology (Mcpherson et al. 1987). The lectin isolated from Sophora japonica seeds was found to be a glycoprotein, its carbohydrate moiety comprising fucose, xylose, mannose and N-acetylglucosamine (Fournet et al. 1987). The lectin was found to bind oligosaccharides with nonreducing terminal Gal beta(1-3/4)GlcNac beta 1 units. S. japonica seeds contained a GalNAc-specific lectin which was highly homologous to though not identical with the GalNAc-specific lectin from the bark (Van Damme et al. 1997). Sophora japonica seed was found to contain an incomplete partial phytohaemagglutinin anti-B2 of the blocking type (Potapov 2004).
Leaf Phytochemicals
Two flavonol tetraglycosides 3-O-α-rhamnopyranosyl(1 → 2)[α-rhamnopyranosyl(1 → 6)]-β-glucopyranoside-7-O-α-rhamnopyranosides of quercetin and kaempferol were isolated from the leaves of Styphnolobium japonicum (Kite et al. 2007). The 3-O-α-rhamnopyranosyl(1 → 2)[α-rhamnopyranosyl(1 → 6)]-β-galactopyranoside-7-O-α-rhamnopyranoside of kaempferol, the 3-O-α-rhamnopyranosyl(1 → 2)[α-rhamnopyranosyl(1 → 6)]-β-glucopyranosides of kaempferol and quercetin and the 3-O-α-rhamnopyranosyl(1 → 2)[α-rhamnopyranosyl(1 → 6)]-β-galactopyranoside of kaempferol were also isolated. An additional constituent obtained was identified as the maltol derivative, 3-hydroxy-2-methyl-4H-pyran-4-one 3-O-(4′-O–p-coumaroyl-6′-O-(3-hydroxy-3-methylglutaroyl))-β-glucopyranoside.
Two isoflavone tetraglycosides genistein 7-O-β-d-glucopyranoside-4′-O-(6″′-O-α-l-rhamnopyranosyl)-β-sophoroside and genistein 7-O-α-l-rhamnopyranoside-4′-O-(6″′-O-α-l-rhamnopyranosyl)-β-sophoroside and six known compounds (genistein 7-O-β-d-glucopyranoside-4′-O-α-d-glucopyranoside, sophorabioside, genistin, rutin, quercetin3-O-β-d-glucopyranoside and kaempferol 3-O-β-d-glucopyranoside) were isolated from S. japonica leaves (Tang et al. 2008a, b). Three flanoids were isolated from the leaves genistein, tectoridin rutin (Kim and Yun-Choi 2008).
The rutin content decreased from young (8.7 %) to mature leaves (4.1 %) and then remained without significant changes all over flowering and fruiting seasons (Balbaa et al. 2009). Two new flavanones with a C15 isoprenoid group, japonicasins A and B (1 and 2), were isolated from the leaves (Zhang et al. 2013a) and represented the first report on the presence of the (2E,7E)-6-isopropyl-3,9-dimethyldeca-2,7,9-trien-1-yl group (C15 isoprenoid group) in isoprenylated flavonoids. The quinolizidine alkaloid, lupanine, was found in the leaves and cell cultures (Wink et al. 1983).
Two lectins, Leaf Lectin I and Leaf Lectin II (LLI and LLII), were purified from the leaves (Hankins et al. 1987). Like the Sophora seed lectin, LLI and LLII were found to be tetrameric glycoproteins containing a single subunit with respect to size. The subunits of LLI (32 kDa) and LLII (34 kDa) were slightly larger than those of the seed lectin (29.5 kDa). The three Sophora lectins displayed indistinguishable specificities, amino acid compositions, specific haemagglutinin activities and extinction coefficients. Although very closely related to the seed lectin, the leaf and seed lectins were not immunologically identical, and they differed in subunit molecular weights, carbohydrate content and in the pH sensitivity of their haemagglutinin activities. The leaf lectins were found to be exclusively sequestered in the protein-storage vacuoles of these tissues (Herman et al. 1988).
Stem/Bark Phytochemicals
Two isoflavone triglycosides, genistein 4′-O-(6″-O-α-l-rhamnopyranosyl)-β-sophoroside and genistein 4′-O-(6″′-O-α-l-rhamnopyranosyl)-β-sophoroside, together with five known compounds, namely, sophorabioside, genistin, rutin, quercetin 3-O-β-d-glucopyranoside and kaempferol 3-O-β-d-glucopyranoside, were isolated from the small branches of Sophora japonica (Tang et al. 2008b). Four flavonoids were isolated from the stem: biochanin A, iridolidone, sissotrin and apigenin (Kim and Yun-Choi 2008). A new isoflavone glycoside, 6-methoxy-7-hydroxy-4′-O-β-d-glucosyl isoflavone, glycitein-4′-O-β-d-glucoside, along with known flavonoids (daidzein, calycosin, trifolirhizin, puerol A, ononin, 5-hydroxypseudobaptigenin-7-O-glucoside, glycitin, calycosin-7-O-glucoside, paratensein-7-O-glucoside), were isolated from the stem bark of Sophora japonica (Park et al. 2010).
Five N-acetyl-galactosamine-specific lectins were isolated from the bark but were absent in other tissues (Hankins et al. 1988). These lectins were immunologically and structurally very similar, but not identical, to the Sophora seed and leaf lectins. The carbohydrate specificities and haemagglutinin activities of these lectins were indistinguishable at pH 8.5 but their activities differed markedly at pH values below 8. All five lectins were found to be tetrameric glycoproteins made up of different combinations of subunits of about 30,000, 30,100, 33,000 M(r) containing 3–5 % covalently attached sugar. Mannose/glucose-specific lectins were found in the bark (Van Damme et al. 1997). The bark lectins were found to be exclusively sequestered in the protein-storage vacuoles of these tissues (Herman et al. 1988). Sophoragrin, a d-mannose/d-glucose-specific lectin (B-SJA-II), was isolated from the bark of S. japonica (Ueno et al. 1991). It was found to have 4subunits a-1 (Mr = 19,400), a-2 (Mr = 18,200), b-1 (Mr = 15,000) and b-2 (Mr = 13,200). Each subunit had a sugar-binding site for the mannosyl core of N-linked oligosaccharide chains and recognized each other sugar specifically to form aggregates. Further studies showed that self-aggregation of sophoragrin was shown to be a unique homopolymerization due to the sugar-specific interaction (Ueda et al. 2004). Sophoragrin was found to sequester endogenous glycoprotein ligands via sugar-specific interactions.
Unidentified Plant Parts Phytochemicals
A new compound, N-feruloyl-N′-cis-feruloyl-putrescine, together with four flavonoids (rutin, quercetin, 3-methylquercetin, orobol) and three putrescine derivatives (N,N′-diferuloyl-putrescine, N,N-dicoumaroyl-putrescine and N-coumaroyl-N-feruloyl-putrescine), was isolated from S. japonica (Lo et al. 2009).
Root Phytochemicals
dl-maackiain and d-maackiain—mono-β-d-glucoside under the proposed name sophojaponicin, a diastereomer of trifolirhizin, were isolated from the roots (Shibata and Nishikawa 1963). Sophora japonica roots were found to contain anhydropisatin (flemichapparin B); irisolidone; 5,7-dihydroxy-3′, 4′-methylenedioxyisoflavone; biochanin A; d-maackiain and β-sitosterol (Komatsu et al. 1976); and oxymatrine (Gan et al. 2011). An aromatic glycoside, named sophoroside A with the structure puerol B 5-O-β-d-glucopyranoside together with puerol A and puerol B, was isolated from the roots (Shirataki et al. 1987). Oxymatrine and matrine were found in the roots (Cheng et al. 2008).
Antioxidant Activity
A flavonol triglycoside, kaempferol 3-O-α-l-rhamnopyranosyl-(1 → 6)-β-d-glucopyranosyl-(1 → 2)-β-d-glucopyranoside, and kaempferol 3-O-[α-l-rhamnopyranosyl-(1 → 6)]-[β-d-glucopyranosyl-(1 → 2)]-β-d-glucopyranoside and kaempferol 3-O-β-d-glucopyranosyl-(1 → 2)-β-d-glucopyranoside-7-O-α-l-rhamnopyranoside isolated from the fruit, exhibited antioxidant activity in DPPH (2,2-diphenyl-1-picrylhydrazyl) and cytochrome-c assay using HL-60 cell system (Tang et al. 2002b). It was found that rutin administration sharply suppressed free radical production in liver microsomes and by phagocytes of iron-overloading rats and only slightly affected these processes in normal rats (Afanas’ev et al. 1995). The selective inhibitory effect of rutin under pathologic conditions induced by iron overload was thought to be due to the formation of inactive iron–rutin complexes which were unable to catalyze the conversion of superoxide ion into reactive hydroxyl radicals, a process responsible for the free-radical-mediated toxic effects of iron overload. In cholesterol-enriched erythrocytes and control erythrocytes, quercetin provided greater protection against lipid peroxidation and reactive oxygen species (ROS) formation, and it preserved better cellular integrity than rutin (López-Revuelta et al. 2006). Both antioxidants suppressed the alterations in membrane fluidity and lipid losses with similar efficiency, reducing haemoglobin oxidation by 30 % and GSH losses by 60 % in the above-mentioned erythrocytes. Cholesterol depletion reduced the efficiency of the antioxidant power of both flavonoids against oxidative damage induced in the erythrocyte membrane, while a stronger degree of protection of GSH and haemoglobin contents was observed, mainly in the presence of rutin.
Wang et al. (2008) found that the polysaccharides of Sophora japonica scavenged hydroxyl and superoxide anion free radicals. Of four plants, Sophora japonica showed significant antioxidant activity in both yeast model and also DPPH free radical scavenging methods. The ethanol extract of S. japonica showed highest levels of phenolics and flavonoids (91.33 GAE mg/g and 151.86 QE mg/g, respectively). A positive linear correlation between antioxidant activity and the total phenolics and flavonoid contents indicates that these compounds are likely to be the main antioxidants contributing to the observed activities (Zhang et al. 2011).
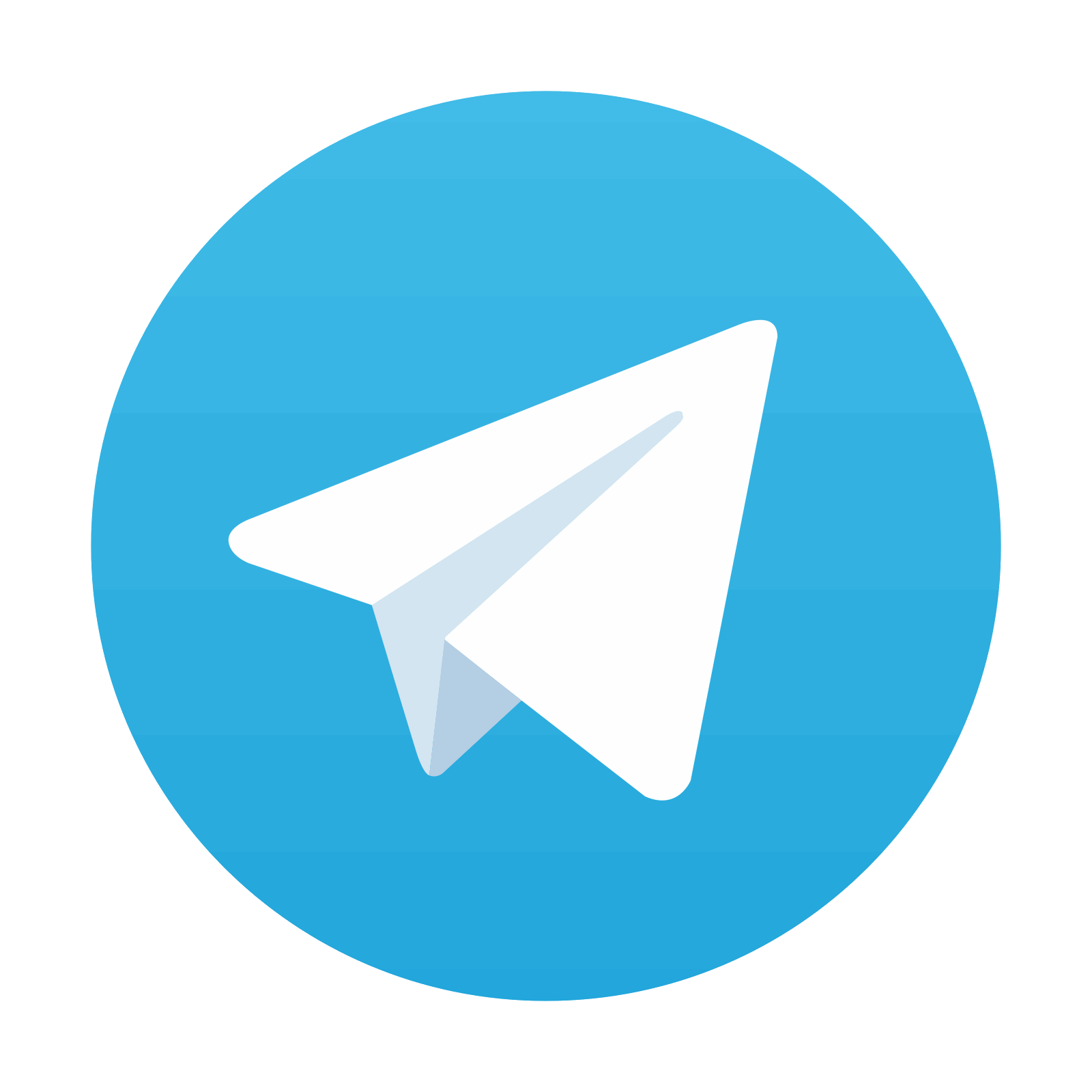
Stay updated, free articles. Join our Telegram channel

Full access? Get Clinical Tree
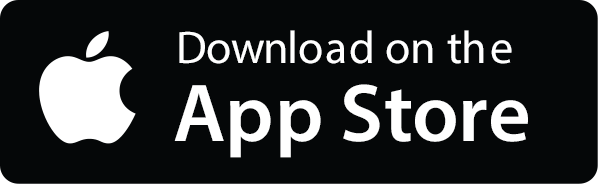
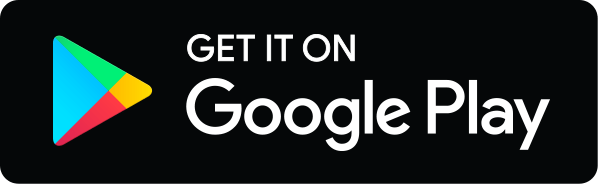