Schematic diagram comparing some features of the parasympathetic and sympathetic divisions of the autonomic nervous system with the somatic motor system. Parasympathetic ganglia are not shown as discrete structures because most of them are diffusely distributed in the walls of the organs innervated. Only 3 of the more than 20 sympathetic ganglia are shown alpha and beta adrenoceptors; ACh, acetylcholine; D, dopamine, D1, dopamine1 receptors; Epi, epinephrine; M, muscarinic; N, nicotinic; NE, norepinephrine.
(Modified and reproduced, with permission, from Katzung BG, editor: Basic & Clinical Pharmacology, 11th ed. McGraw-Hill, 2009: Fig. 6-1.)
There are many sensory (afferent) fibers in autonomic nerves. These are of considerable importance for the physiologic control of the involuntary organs but are directly influenced by only a few drugs.
Central Roots of Origin
The parasympathetic preganglionic motor fibers originate in cranial nerve nuclei III, VII, IX, and X and in sacral segments (usually S2-S4) of the spinal cord. The sympathetic preganglionic fibers originate in the thoracic (T1-T12) and lumbar (L1-L5) segments of the cord.
Location of Ganglia
Most of the sympathetic ganglia are located in 2 paravertebral chains that lie along the spinal column. A few (the prevertebral ganglia) are located on the anterior aspect of the abdominal aorta. Most of the parasympathetic ganglia are located in the organs innervated more distant from the spinal cord. Because of the locations of the ganglia, the preganglionic sympathetic fibers are short and the postganglionic fibers are long. The opposite is true for the parasympathetic system: preganglionic fibers are longer and postganglionic fibers are short.
Uninnervated Receptors
Some receptors that respond to autonomic transmitters and drugs receive no innervation. These include muscarinic receptors on the endothelium of blood vessels, some presynaptic receptors, and, in some species, the adrenoceptors on apocrine sweat glands and 2 and
adrenoceptors in blood vessels.
Neurotransmitter Aspects of the ANS
The synthesis, storage, release, receptor interactions, and termination of action of the neurotransmitters all contribute to the action of autonomic drugs (Figure 6-2).
FIGURE 6-2
Characteristics of transmitter synthesis, storage, release, and termination of action at cholinergic and noradrenergic nerve terminals are shown from the top downward. Circles represent transporters; ACh, acetylcholine; AChE, acetylcholinesterase; ChAT, choline acetyltransferase; DOPA, dihydroxyphenylalanine; NE, norepinephrine; NET, norepinephrine transporter; TCA, tricyclic antidepressant; TH, tyrosine hydroxylase.
Cholinergic Transmission
Acetylcholine (ACh) is the primary transmitter in all autonomic ganglia and at the synapses between parasympathetic postganglionic neurons and their effector cells. It is also the primary transmitter at the somatic (voluntary) skeletal muscle neuromuscular junction (Figure 6-1).
Synthesis and Storage
Acetylcholine is synthesized in the nerve terminal from acetyl-CoA (produced in mitochondria) and choline (transported across the cell membrane) by the enzyme choline acetyltransferase (ChAT). The rate-limiting step is probably the transport of choline into the nerve terminal. This transport can be inhibited by the research drug hemicholinium. Acetylcholine is actively transported into its vesicles for storage by the vesicle-associated transporter, VAT. This process can be inhibited by another research drug, vesamicol.
Release of Acetylcholine
Release of transmitter stores from vesicles in the nerve ending requires the entry of calcium through calcium channels and triggering of an interaction between SNARE (soluble N-ethylmaleimide-sensitive-factor attachment protein receptor) proteins. SNARE proteins include v-SNARES associated with the vesicles (VAMPs, vesicle-associated membrane proteins: synaptobrevin, synaptotagmin) and t-SNARE proteins associated with the nerve terminal membrane (SNAPs, synaptosome-associated proteins: SNAP25, syntaxin, and others). This interaction results in docking of the vesicle to the terminal membrane and, with influx of calcium, fusion of the membranes of the vesicles with the nerve-ending membranes, the opening of a pore to the extracellular space, and the release of the stored transmitter. The several types of botulinum toxins enzymatically alter synaptobrevin or one of the other docking or fusion proteins to prevent the release process.
Termination of Action of Acetylcholine
The action of acetylcholine in the synapse is normally terminated by metabolism to acetate and choline by the enzyme acetylcholinesterase in the synaptic cleft. The products are not excreted but are recycled in the body. Inhibition of acetylcholinesterase is an important therapeutic (and potentially toxic) effect of several drugs.
Drug Effects on Synthesis, Storage, Release, and Termination of Action of Acetylcholine
Drugs that block the synthesis of acetylcholine (eg, hemicholinium), its storage (eg, vesamicol), or its release (eg, botulinum toxin) are not very useful for systemic therapy because their effects are not sufficiently selective (ie, PANS and SANS ganglia and somatic neuromuscular junctions all may be blocked). However, because botulinum toxin is a very large molecule and diffuses very slowly, it can be used by injection for relatively selective local effects.
Adrenergic Transmission
Norepinephrine (NE) is the primary transmitter at the sympathetic postganglionic neuron-effector cell synapses in most tissues. Important exceptions include sympathetic fibers to thermoregulatory (eccrine) sweat glands and probably vasodilator sympathetic fibers in skeletal muscle, which release acetylcholine. Dopamine may be a vasodilator transmitter in renal blood vessels, but norepinephrine is a vasoconstrictor of these vessels.
Synthesis and Storage
The synthesis of dopamine and norepinephrine requires several steps (Figure 6-2). After transport across the cell membrane, tyrosine is hydroxylated by tyrosine hydroxylase (the rate-limiting step) to DOPA (dihydroxyphenyl-alanine), decarboxylated to dopamine, and (inside the vesicle) hydroxylated to norepinephrine. Tyrosine hydroxylase can be inhibited by metyrosine. Norepinephrine and dopamine are transported into vesicles and stored there. Monoamine oxidase (MAO) is present on mitochondria in the adrenergic nerve ending and inactivates a portion of the dopamine and norepinephrine in the cytoplasm. Therefore, MAO inhibitors may increase the stores of these transmitters and other amines in the nerve endings (Chapter 30). The vesicular transporter can be inhibited by reserpine , resulting in depletion of transmitter stores.
Release and Termination of Action
Dopamine and norepinephrine are released from their nerve endings by the same calcium-dependent mechanism responsible for acetylcholine release (see prior discussion). Termination of action, however, is quite different. Metabolism is not responsible for termination of action of the catecholamine transmitters, norepinephrine and dopamine. Rather, diffusion and reuptake (especially uptake-1, Figure 6-2, by the norepinephrine transporter, NET, or the dopamine transporter, DAT) reduce their concentration in the synaptic cleft and stop their action. Outside the cleft, these transmitters can be metabolized—by MAO and catechol-O-methyltransferase (COMT)—and the products of these enzymatic reactions are excreted. Determination of the 24-h excretion of metanephrine, normetanephrine, 3-methoxy-4-hydroxymandelic acid (VMA), and other metabolites provides a measure of the total body production of catecholamines, a determination useful in diagnosing conditions such as pheochromocytoma. Inhibition of MAO increases stores of catecholamines and has both therapeutic and toxic potential.
Drug Effects on Adrenergic Transmission
Drugs that block norepinephrine synthesis (eg, metyrosine) or catecholamine storage (eg, reserpine) or release (eg, guanethidine) have been used in treatment of several diseases (eg, pheochromocytoma, hypertension) because they block sympathetic but not parasympathetic functions. Other drugs promote catecholamine release (eg, the amphetamine-like agents) and predictably cause sympathomimetic effects.
Cotransmitters
Many (probably all) autonomic nerves have transmitter vesicles that contain other transmitter molecules in addition to the primary agents (acetylcholine or norepinephrine) previously described. These cotransmitters may be localized in the same vesicles as the primary transmitter or in a separate population of vesicles. Substances recognized to date as cotransmitters include ATP (adenosine triphosphate), enkephalins, vasoactive intestinal peptide, neuropeptide Y, substance P, neurotensin, somatostatin, and others. Their main role in autonomic function appears to involve modulation of synaptic transmission. The same substances function as primary transmitters in other synapses.
Skill Keeper: Drug Permeation
(See Chapter 1)
Botulinum toxin is a very large protein molecule and does not diffuse readily when injected into tissue. In spite of this property, it is able to enter cholinergic nerve endings from the extracellular space and block the release of acetylcholine. How might it cross the lipid membrane barrier? The Skill Keeper Answer appears at the end of the chapter.
Receptor Characteristics
The major receptor systems in the ANS include cholinoceptors, adrenoceptors, and dopamine receptors, which have been studied in some detail. The numerous receptors for cotransmitter substances have not been as fully defined.
Cholinoceptors
Also referred to as cholinergic receptors, these molecules respond to acetylcholine and its analogs. Cholinoceptors are subdivided as follows (Table 6-1):
Muscarinic Receptors
As their name suggests, these receptors respond to muscarine (an alkaloid) as well as to acetylcholine. The effects of activation of these receptors resemble those of postganglionic parasympathetic nerve stimulation. Muscarinic receptors are located primarily on autonomic effector cells (including heart, vascular endothelium, smooth muscle, presynaptic nerve terminals, and exocrine glands). Evidence (including their genes) has been found for 5 subtypes, of which 3 appear to be important in peripheral autonomic transmission. All 5 are G-protein-coupled receptors (see Chapter 2).
Nicotinic Receptors
These receptors are located on ion channels and respond to acetylcholine and nicotine, another acetylcholine mimic (but not to muscarine) by opening the channel. The 2 major nicotinic subtypes are located in ganglia and in skeletal muscle end plates. The nicotinic receptors are the primary receptors for transmission at these sites.
TABLE 6-1 Characteristics of the most important cholinoceptors in the peripheral nervous system.
Receptor Location Mechanism Major Functions M1
Nerve endings Gq-coupled
IP 3, DAG cascade
M2
Heart, some nerve endings Gi-coupled
cAMP, activates K+ channels
M3
Effector cells: smooth muscle, glands, endothelium Gq-coupled
IP3, DAG cascade
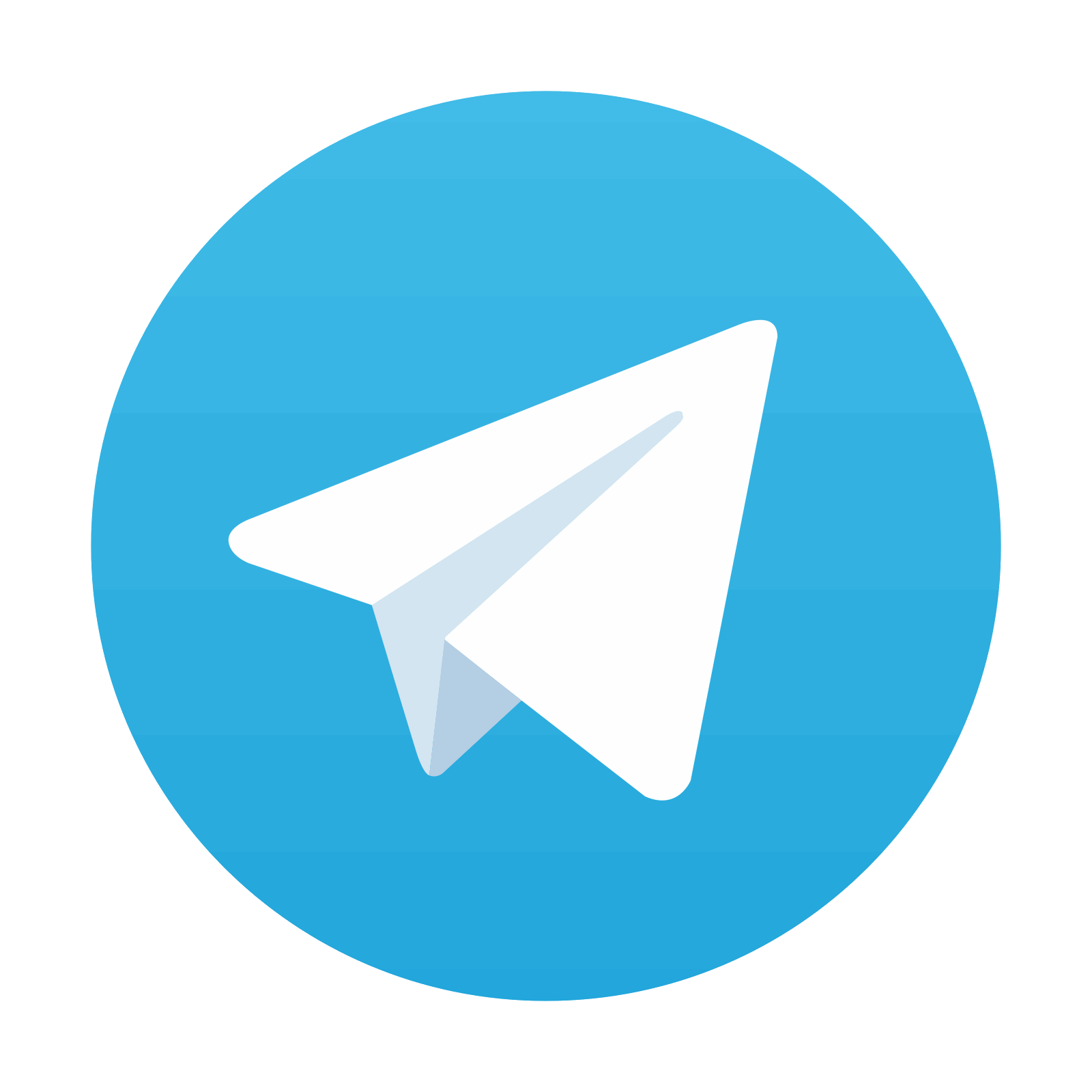
Stay updated, free articles. Join our Telegram channel

Full access? Get Clinical Tree
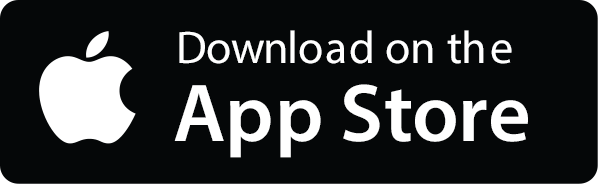
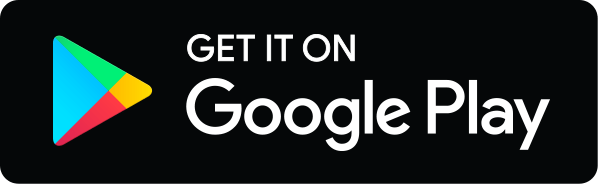