In patients with subacute presentations, fever is typically low-grade and rarely exceeds 39.4°C (103°F); in contrast, temperatures of 39.4°–40°C (103°–104°F) are often noted in acute endocarditis. Fever may be blunted in patients who are elderly, are severely debilitated, or have renal failure.
Cardiac Manifestations Although heart murmurs are usually indicative of the predisposing cardiac pathology rather than of endocarditis, valvular damage and ruptured chordae may result in new regurgitant murmurs. In acute endocarditis involving a normal valve, murmurs may be absent initially but ultimately are detected in 85% of cases. Congestive heart failure (CHF) develops in 30–40% of patients as a consequence of valvular dysfunction. Occasionally, CHF is due to endocarditis-associated myocarditis or an intracardiac fistula. Heart failure due to aortic valve dysfunction progresses more rapidly than does that due to mitral valve dysfunction. Extension of infection beyond valve leaflets into adjacent annular or myocardial tissue results in perivalvular abscesses, which in turn may cause intracardiac fistulae with new murmurs. Abscesses may burrow from the aortic valve annulus through the epicardium, causing pericarditis, or into the upper ventricular septum, where they may interrupt the conduction system, leading to varying degrees of heart block. Mitral perivalvular abscesses, which are usually more distant from the conduction system, only rarely cause conduction abnormalities; if such abnormalities occur in this setting, the conduction pathway is most likely disrupted near the atrioventricular node or in the proximal bundle of His. Emboli to a coronary artery occur in 2% of patients and may result in myocardial infarction.
Noncardiac Manifestations The classic nonsuppurative peripheral manifestations of subacute endocarditis (e.g., Janeway lesions; Fig. 155-2A) are related to prolonged infection; with early diagnosis and treatment, these have become infrequent. In contrast, septic embolization mimicking some of these lesions (subungual hemorrhage, Osler’s nodes) is common in patients with acute S. aureus endocarditis (Fig. 155-2B). Musculoskeletal pain usually remits promptly with treatment but must be distinguished from focal metastatic infections (e.g., spondylodiscitis), which may complicate 10–15% of cases. Hematogenously seeded focal infection occurs most often in the skin, spleen, kidneys, skeletal system, and meninges. Arterial emboli, one-half of which precede the diagnosis, are clinically apparent in up to 50% of patients. Endocarditis caused by S. aureus, vegetations >10 mm in diameter (as measured by echocardiography), and infection involving the mitral valve, especially the anterior leaflet, are independently associated with an increased risk of embolization. Symptoms, pain, or ischemia-induced dysfunction relate to the organ or area suffering embolic arterial occlusion (e.g., kidney, spleen, bowel, extremity). Cerebrovascular emboli presenting as strokes or occasionally as encephalopathy complicate 15–35% of cases of endocarditis. Again, one-half of these events precede the diagnosis of endocarditis. The frequency of stroke is 8 per 1000 patient-days during the week prior to diagnosis; the figure falls to 4.8 and 1.7 per 1000 patient-days during the first and second weeks of effective antimicrobial therapy, respectively. This decline exceeds that which can be attributed to change in vegetation size. Only 3% of strokes occur after 1 week of effective therapy. Emboli occurring late during or after effective therapy do not in themselves constitute evidence of failed antimicrobial treatment.
FIGURE 155-2 A. Janeway lesions on toe (left) and plantar surface (right) of the foot in subacute Neisseria mucosa endocarditis. (Image courtesy of Rachel Baden, MD.) B. Septic emboli with hemorrhage and infarction due to acute Staphylococcus aureus endocarditis.
Other neurologic complications include aseptic or purulent meningitis, intracranial hemorrhage due to hemorrhagic infarcts or ruptured mycotic aneurysms, and seizures. (Mycotic aneurysms are focal dilations of arteries occurring at points in the artery wall that have been weakened by infection in the vasa vasorum or where septic emboli have lodged.) Microabscesses in brain and meninges occur commonly in S. aureus endocarditis; surgically drainable intracerebral abscesses are infrequent.
Immune complex deposition on the glomerular basement membrane causes diffuse hypocomplementemic glomerulonephritis and renal dysfunction, which typically improve with effective antimicrobial therapy. Embolic renal infarcts cause flank pain and hematuria but rarely cause renal dysfunction.
Manifestations of Specific Predisposing Conditions Almost 50% of endocarditis associated with injection drug use is limited to the tricuspid valve and presents with fever but with faint or no murmur and no peripheral manifestations. Septic pulmonary emboli, which are common with tricuspid endocarditis, cause cough, pleuritic chest pain, nodular pulmonary infiltrates, or occasionally pyopneumothorax. Infection of the aortic or mitral valves presents with the typical clinical features of endocarditis, including peripheral manifestations.
If not associated with a retained intracardiac device or masked by the symptoms of concurrent comorbid illness, health care–associated endocarditis has typical manifestations. CIED endocarditis may be associated with obvious or cryptic generator pocket infection and results in fever, minimal murmur, and pulmonary symptoms due to septic emboli. Late-onset PVE presents with typical clinical features. In cases arising within 60 days of valve surgery (early onset), typical symptoms may be obscured by comorbidity associated with recent surgery. In both early-onset and more delayed presentations, paravalvular infection is common and often results in partial valve dehiscence, regurgitant murmurs, CHF, or disruption of the conduction system.
DIAGNOSIS
In order to avoid delayed or missed diagnosis, careful clinical, microbiologic, and echocardiographic evaluation should be pursued when febrile patients have endocarditis predispositions, cardiac or noncardiac features of endocarditis, or microbiologic findings consistent with endocarditis (e.g., a stroke or splenic infarct, multiple positive blood cultures for an endocarditis-associated organism).
The Duke Criteria The diagnosis of infective endocarditis is established with certainty only when vegetations are examined histologically and microbiologically. Nevertheless, a highly sensitive and specific diagnostic schema—known as the modified Duke criteria—is based on clinical, laboratory, and echocardiographic findings commonly encountered in patients with endocarditis (Table 155-3). While developed as a research tool rather than for patient management, the criteria can be a helpful diagnostic tool. If the criteria are to be maximally helpful in evaluating patients, appropriate data must be collected. Furthermore, clinical judgment must be exercised in order to use the criteria effectively. Documentation of two major criteria, of one major criterion and three minor criteria, or of five minor criteria allows a clinical diagnosis of definite endocarditis. The diagnosis of endocarditis is rejected if an alternative diagnosis is established, if symptoms resolve and do not recur with ≤4 days of antibiotic therapy, or if surgery or autopsy after ≤4 days of antimicrobial therapy yields no histologic evidence of endocarditis. Illnesses not classified as definite endocarditis or rejected as such are considered cases of possible infective endocarditis when either one major and one minor criterion or three minor criteria are fulfilled. Requiring some clinical features of endocarditis for classification as possible infective endocarditis increases the specificity of the schema without significantly reducing its sensitivity. Unless there are extenuating circumstances, patients with definite or possible endocarditis are treated as such.
THE MODIFIED DUKE CRITERIA FOR THE CLINICAL DIAGNOSIS OF INFECTIVE ENDOCARDITISa |
aDefinite endocarditis is defined by documentation of two major criteria, of one major criterion and three minor criteria, or of five minor criteria. See text for further details. bTransesophageal echocardiography is required for optimal assessment of possible prosthetic valve endocarditis or complicated endocarditis. cValvular disease with stenosis or regurgitation, presence of a prosthetic valve, congenital heart disease including corrected or partially corrected conditions (except isolated atrial septal defect, repaired ventricular septal defect, or closed patent ductus arteriosus), prior endocarditis, or hypertrophic cardiomyopathy. dExcluding single positive cultures for coagulase-negative staphylococci and diphtheroids, which are common culture contaminants, or for organisms that do not cause endocarditis frequently, such as gram-negative bacilli.
Source: Adapted from JS Li et al: Clin Infect Dis 30:633, 2000. With permission from Oxford University Press.
The criteria emphasize bacteremia and echocardiographic findings typical of endocarditis. The requirement for multiple positive blood cultures over time is consistent with the continuous low-density bacteremia characteristic of endocarditis. Among patients with untreated endocarditis who ultimately have a positive blood culture, 95% of all blood cultures are positive. The diagnostic criteria attach significance to the species of organism isolated from blood cultures. To fulfill a major criterion, the isolation of an organism that causes both endocarditis and bacteremia in the absence of endocarditis (e.g., S. aureus, enterococci) must take place repeatedly (i.e., persistent bacteremia) and in the absence of a primary focus of infection. Organisms that rarely cause endocarditis but commonly contaminate blood cultures (e.g., diphtheroids, CoNS) must be isolated repeatedly if their isolation is to serve as a major criterion.
Blood Cultures Isolation of the causative microorganism from blood cultures is critical for diagnosis and for planning treatment. In patients with suspected NVE, PVE, or CIED endocarditis who have not received antibiotics during the prior 2 weeks, three 2-bottle blood culture sets, separated from one another by at least 2 h, should be obtained from different venipuncture sites over 24 h. If the cultures remain negative after 48–72 h, two or three additional blood culture sets should be obtained, and the laboratory should be consulted for advice regarding optimal culture techniques. Pending culture results, empirical antimicrobial therapy should be withheld initially from hemodynamically stable patients with suspected subacute endocarditis, especially those who have received antibiotics within the preceding 2 weeks. Thus, if necessary, additional blood culture sets can be obtained without the confounding effect of empirical treatment. Patients with acute endocarditis or with deteriorating hemodynamics who may require urgent surgery should receive empirical treatment immediately after three sets of blood cultures are obtained over several hours.
Non-Blood-Culture Tests Serologic tests can be used to implicate organisms that are difficult to recover by blood culture: Brucella, Bartonella, Legionella, Chlamydia psittaci, and C. burnetii. Pathogens can also be identified in vegetations by culture, microscopic examination with special stains (i.e., the periodic acid–Schiff stain for T. whipplei), or direct fluorescence antibody techniques and by the use of polymerase chain reaction to recover unique microbial DNA or DNA encoding the 16S or 28S ribosomal unit (16S rRNA or 28S rRNA); sequencing of these DNAs allows identification of bacteria and fungi, respectively.
Echocardiography Echocardiography anatomically confirms and measures vegetations, detects intracardiac complications, and assesses cardiac function (Fig. 155-3). Transthoracic echocardiography (TTE) is noninvasive and exceptionally specific; however, it cannot image vegetations <2 mm in diameter, and in 20% of patients it is technically inadequate because of emphysema or body habitus. TTE detects vegetations in 65–80% of patients with definite clinical endocarditis but is not optimal for evaluating prosthetic valves or detecting intracardiac complications. TEE is safe and detects vegetations in >90% of patients with definite endocarditis; nevertheless, initial studies may yield false-negative results in 6–18% of endocarditis patients. When endocarditis is likely, a negative TEE result does not exclude the diagnosis but rather warrants repetition of the study once or twice in 7–10 days. TEE is the optimal method for the diagnosis of PVE, the detection of myocardial abscess, valve perforation, or intracardiac fistulae and for the detection of vegetations in patients with CIED. In patients with CIED and negative blood cultures, a mass adherent to the lead is likely to be a bland thrombosis rather than an infected vegetation.
FIGURE 155-3 Imaging of a mitral valve infected with Staphylococcus aureus by low-esophageal, four-chamber-view, transesophageal echocardiography (TEE). A. Two-dimensional echocardiogram showing a large vegetation with an adjacent echolucent abscess cavity. B. Color-flow Doppler image showing severe mitral regurgitation through both the abscess-fistula and the central valve orifice. A, abscess; A-F, abscess-fistula; L, valve leaflets; LA, left atrium; LV, left ventricle; MR, mitral central valve regurgitation; RV, right ventricle; veg, vegetation. (With permission of Andrew Burger, MD.)
Because S. aureus bacteremia is associated with a high prevalence of endocarditis, routine echocardiographic evaluation (TTE or preferably TEE) is recommended in these patients. Patients with nosocomial S. aureus bacteremia are at increased risk of endocarditis if one or more of the following are present: positive blood cultures for 2–4 days, hemodialysis dependency, a permanent intracardiac device, spine infection, nonvertebral osteomyelitis, or an endocarditis-predisposing valve abnormality. Ideally, these patients should be evaluated with TEE. In patients with none of these findings, the risk of endocarditis is low and evaluation with TTE may suffice.
Experts favor echocardiographic evaluation of all patients with a clinical diagnosis of endocarditis; however, the test should not be used to screen patients with a low probability of endocarditis (e.g., patients with unexplained fever). An American Heart Association approach to the use of echocardiography for evaluation of patients with suspected endocarditis is illustrated in (Fig. 155-4).
FIGURE 155-4 The diagnostic use of transesophageal and transtracheal echocardiography (TEE and TTE, respectively). †High initial patient risk for infective endocarditis (IE), as listed in Table 155-8, or evidence of intracardiac complications (new regurgitant murmur, new electrocardiographic conduction changes, or congestive heart failure). *High-risk echocardiographic features include large vegetations, valve insufficiency, paravalvular infection, or ventricular dysfunction. Rx indicates initiation of antibiotic therapy. (Reproduced with permission from Diagnosis and Management of Infective Endocarditis and Its Complications. Circulation 98:2936, 1998. © 1998 American Heart Association.)
Other Studies Many studies that are not diagnostic—i.e., complete blood count, creatinine determination, liver function tests, chest radiography, and electrocardiography—are important in the management of patients with endocarditis. The erythrocyte sedimentation rate, C-reactive protein level, and circulating immune complex titer are commonly increased in endocarditis (Table 155-2). Cardiac catheterization is useful primarily to assess coronary artery patency in older individuals who are to undergo surgery for endocarditis.
TREATMENT | INFECTIVE ENDOCARDITIS |
ANTIMICROBIAL THERAPY
To cure endocarditis, all bacteria in the vegetation must be killed. However, it is difficult to eradicate these bacteria because local host defenses are deficient and because the bacteria are largely nongrowing and metabolically inactive and thus are less easily killed by antibiotics. Accordingly, therapy must be bactericidal and prolonged. Antibiotics are generally given parenterally to achieve serum concentrations that, through passive diffusion, result in effective concentrations in the depths of the vegetation. To select effective therapy requires knowledge of the susceptibility of the causative microorganisms. The decision to initiate treatment empirically must balance the need to establish a microbiologic diagnosis against the potential progression of disease or the need for urgent surgery (see “Blood Cultures,” earlier). Simultaneous infection at other sites (such as the meninges), allergies, end-organ dysfunction, interactions with concomitantly administered medications, and risks of adverse events must be considered in the selection of therapy.
Although given for several weeks longer, the regimens recommended for the treatment of PVE (except that caused by staphylococci) are similar to those used to treat NVE (Table 155-4). Recommended doses and durations of therapy should be followed unless alterations are required by end-organ dysfunction or adverse events.
ANTIBIOTIC TREATMENT FOR INFECTIVE ENDOCARDITIS CAUSED BY COMMON ORGANISMSa |
Organism-Specific Therapies • STREPTOCOCCI Optimal therapy for streptococcal endocarditis is based on the minimal inhibitory concentration (MIC) of penicillin for the causative isolate (Table 155-4). The 2-week penicillin/gentamicin or ceftriaxone/gentamicin regimens should not be used to treat PVE or complicated NVE. Caution should be exercised in considering aminoglycoside-containing regimens for the treatment of patients at increased risk for aminoglycoside toxicity. The regimens recommended for relatively penicillin-resistant streptococci are advocated for treatment of group B, C, or G streptococcal endocarditis. Nutritionally variant organisms (Granulicatella or Abiotrophia species) and Gemella species are treated with the regimens for moderately penicillin-resistant streptococci, as is PVE caused by these organisms or by streptococci with a penicillin MIC of >0.1 μg/mL (Table 155-4).
ENTEROCOCCI Enterococci are resistant to oxacillin, nafcillin, and the cephalosporins and are only inhibited—not killed—by penicillin, ampicillin, teicoplanin (not available in the United States), and vancomycin. To kill enterococci requires the synergistic interaction of a cell wall–active antibiotic that is effective at achievable serum concentrations (penicillin, ampicillin, vancomycin, or teicoplanin) and an aminoglycoside (gentamicin or streptomycin) to which the isolate does not exhibit high-level resistance. An isolate’s resistance to cell wall–active agents or its ability to replicate in the presence of gentamicin at ≥500 μg/mL or streptomycin at 1000–2000 μg/mL—a phenomenon called high-level aminoglycoside resistance—indicates that the ineffective antimicrobial agent cannot participate in the interaction to produce killing. High-level resistance to gentamicin predicts that tobramycin, netilmicin, amikacin, and kanamycin also will be ineffective. In fact, even when enterococci are not highly resistant to gentamicin, it is difficult to predict the ability of these other aminoglycosides to participate in synergistic killing; consequently, they should not, in general, be used to treat enterococcal endocarditis. High concentrations of ampicillin plus ceftriaxone or cefotaxime, by expanded binding of penicillin-binding proteins, also kill E. faecalis in vitro and in animal models of endocarditis.
Enterococci must be tested for high-level resistance to streptomycin and gentamicin, β-lactamase production, and susceptibility to penicillin and ampicillin (MIC, <8 μg/mL) and to vancomycin (MIC, ≤4 μg/mL) and teicoplanin (MIC ≤2 μg/ml). If the isolate produces β-lactamase, ampicillin/sulbactam or vancomycin can be used as the cell wall–active component; if the penicillin/ampicillin MIC is ≥8 μg/mL, vancomycin can be considered; and if the vancomycin MIC is ≥8 μg/mL, penicillin or ampicillin can be considered. In the absence of high-level resistance, gentamicin or streptomycin should be used as the aminoglycoside (Table 155-4). Although the dose of gentamicin used to achieve bactericidal synergy in treating enterococcal endocarditis is smaller than that used in standard therapy, nephrotoxicity (or vestibular toxicity with streptomycin) is not uncommon during treatment lasting 4–6 weeks. Regimens in which the aminoglycoside component is given for only 2–3 weeks have been curative and associated with less nephrotoxicity than those using longer courses of gentamicin. Thus regimens wherein gentamicin is administered for only 2–3 weeks are preferred by some.
If there is high-level resistance to both gentamicin and streptomycin, a synergistic bactericidal effect cannot be achieved by the addition of an aminoglycoside; thus no aminoglycoside should be given. Instead, an 8- to 12-week course of a single cell wall–active agent can be considered; for E. faecalis endocarditis, high doses of ampicillin combined with ceftriaxone or cefotaxime are suggested (Table 155-4). Nonrandomized comparative studies suggest that ampicillin-ceftriaxone may be as effective as (and less nephrotoxic than) penicillin or ampicillin plus an aminoglycoside in the treatment of E. faecalis endocarditis. Given the reduced risk of nephrotoxicity with ampicillin-ceftriaxone therapy, this regimen may also be preferred in patients who are at increased risk for aminoglycoside nephrotoxicity.
If the enterococcal isolate is resistant to all of the commonly used agents, suppression of bacteremia followed by surgical treatment should be considered. The role of newer agents potentially active against multidrug-resistant enterococci (quinupristin/dalfopristin [E. faecium only], linezolid, and daptomycin) in the treatment of endocarditis has not been established.
STAPHYLOCOCCI The regimens used to treat staphylococcal endocarditis (Table 155-4) are based not on coagulase production but rather on the presence or absence of a prosthetic valve or foreign device, the native valve(s) involved, and the susceptibility of the isolate to penicillin, methicillin, and vancomycin. All staphylococci are considered penicillin-resistant until shown not to produce penicillinase. Similarly, methicillin resistance has become so prevalent among staphylococci that empirical therapy should be initiated with a regimen that covers methicillin-resistant organisms and should later be revised if the isolate proves to be susceptible to methicillin. The addition of 3–5 days of gentamicin to a β-lactam antibiotic or vancomycin to enhance therapy for native mitral or aortic valve endocarditis has not improved survival rates and may be associated with nephrotoxicity. Neither this addition nor the addition of fusidic acid or rifampin is recommended.
For treatment of endocarditis caused by methicillin-resistant S. aureus (MRSA), vancomycin, dosed to achieve trough concentrations of 15–20 μg/mL, is recommended, with the caveat that this regimen may be associated with nephrotoxicity. Although resistance to vancomycin among staphylococci is rare, reduced vancomycin susceptibility among MRSA strains is increasingly encountered. Isolates with a vancomycin MIC of 4–16 μg/mL have intermediate susceptibility and are referred to as vancomycin-intermediate S. aureus (VISA). Isolates with an MIC of 2 μg/mL may harbor subpopulations with higher MICs. These heteroresistant VISA (hVISA) isolates are not detectable by routine susceptibility testing. Because of the pharmacokinetics/pharmacodynamics of vancomycin, killing of MRSA with a vancomycin MIC of >1.0 μg/mL is unpredictable, even with aggressive vancomycin dosing. Although not approved by the U.S. Food and Drug Administration for this indication, daptomycin (6 mg/kg [or, as some experts prefer, 8–10 mg/kg] IV once daily) has been recommended as an alternative to vancomycin, particularly for left-sided endocarditis caused by VISA, hVISA, or isolates with a vancomycin MIC of >1.0 μg/mL. These isolates should be tested to document daptomycin susceptibility. Daptomycin activity against MRSA—even against some isolates with reduced daptomycin susceptibility—is enhanced by the addition of nafcillin or ceftaroline. Case reports suggest that either the latter combinations or ceftaroline alone (600 mg IV q8h) may be effective in recalcitrant MRSA endocarditis. Nevertheless, a discussion of treatment of endocarditis in which MRSA bacteremia persists despite therapy is beyond the scope of this chapter and requires consultation with an infectious disease specialist. The efficacy of linezolid for left-sided MRSA endocarditis has not been established. Although not widely adopted by other groups, the recommendation of the British Society for Antimicrobial Chemotherapy is that a second drug be added to vancomycin (rifampin) or to daptomycin (rifampin, gentamicin, or linezolid) for the treatment of NVE due to MRSA.
Methicillin-susceptible S. aureus endocarditis that is uncomplicated and limited to the tricuspid or pulmonic valve can often be treated with a 2-week course that combines oxacillin or nafcillin (but not vancomycin) with gentamicin. However, patients with prolonged fever (≥5 days) during therapy or multiple septic pulmonary emboli should receive standard-duration therapy. Vancomycin plus gentamicin for 2 weeks as treatment for right-sided endocarditis caused by MRSA yields suboptimal results; thus this entity is treated for 4 weeks with vancomycin or daptomycin (6 mg/kg as a single daily dose).
Staphylococcal PVE is treated for 6–8 weeks with a multidrug regimen. Rifampin is an essential component because it kills staphylococci that are adherent to foreign material in a biofilm. Two other agents (selected on the basis of susceptibility testing) are combined with rifampin to prevent in vivo emergence of resistance. Because many staphylococci (particularly MRSA and Staphylococcus epidermidis) are resistant to gentamicin, the isolate’s susceptibility to gentamicin or an alternative agent should be established before rifampin treatment is begun. If the isolate is resistant to gentamicin, then another aminoglycoside, a fluoroquinolone (chosen on the basis of susceptibility), or another active agent should be substituted for gentamicin.
OTHER ORGANISMS In the absence of meningitis, endocarditis caused by Streptococcus pneumoniae isolates with a penicillin MIC of ≤1 μg/mL can be treated with IV penicillin (4 million units every 4 h), ceftriaxone (2 g/d as a single dose), or cefotaxime (at a comparable dosage). Infection caused by pneumococcal strains with a penicillin MIC of ≥2 μg/mL should be treated with vancomycin. If meningitis is suspected or present, treatment with vancomycin plus ceftriaxone—at the doses advised for meningitis—should be initiated until susceptibility results are known. Definitive therapy should then be selected on the basis of meningitis breakpoints (penicillin MIC, 0.06 μg/mL; or ceftriaxone MIC, 0.5 μg/mL). P. aeruginosa endocarditis is treated with an antipseudomonal penicillin (ticarcillin or piperacillin) and high doses of tobramycin (8 mg/kg per day in three divided doses). Endocarditis caused by Enterobacteriaceae is treated with a potent β-lactam antibiotic plus an aminoglycoside. Corynebacterial endocarditis is treated with a penicillin plus an aminoglycoside (if the organism is susceptible to the aminoglycoside) or with vancomycin, which is highly bactericidal for most strains. Therapy for Candida endocarditis consists of amphotericin B plus flucytosine and early surgery; long-term (if not indefinite) suppression with an oral azole is advised. Echinocandin treatment of Candida endocarditis has been effective in sporadic cases; nevertheless, the role of echinocandins in this setting has not been established.
Empirical Therapy In designing therapy (largely with antimicrobials and doses from Table 155-4 to target putative microorganisms) to be administered before culture results are known or when cultures are negative, clinical clues (e.g., acute vs. subacute presentation, site of infection, patient’s predispositions) as well as epidemiologic clues to etiology must be considered. Thus empirical therapy for acute endocarditis in an injection drug user should cover MRSA and gram-negative bacilli. Treatment with vancomycin plus gentamicin, initiated immediately after blood samples are obtained for culture, covers these organisms as well as many other potential causes. Similarly, treatment of health care–associated endocarditis must cover MRSA. In the treatment of culture-negative episodes, marantic endocarditis must be excluded and fastidious organisms sought by serologic testing. In the absence of prior antibiotic therapy, it is unlikely that S. aureus, CoNS, or enterococcal infection will present with negative blood cultures; thus, in this situation, recommended empirical therapy targets not these organisms but rather nutritionally variant organisms, the HACEK group, and Bartonella species. Pending the availability of diagnostic data, blood culture–negative subacute NVE is treated with gentamicin plus ampicillin-sulbactam (12 g every 24 h) or ceftriaxone; doxycycline (100 mg twice daily) is added for enhanced Bartonella coverage. For culture-negative PVE, vancomycin, gentamicin, cefepime, and rifampin should be used if the prosthetic valve has been in place for ≤1 year. Empirical therapy for infected prosthetic valves in place for >1 year is similar to that for culture-negative NVE. If cultures may be negative because of confounding by prior antibiotic administration, broader empirical therapy may be indicated, with particular attention to pathogens that are likely to be inhibited by the specific prior therapy.
CIED Endocarditis Antimicrobial therapy for CIED endocarditis is adjunctive to complete device removal. The antimicrobial selected is based on the causative organism and should be used as recommended for NVE (Table 155-4). Bacteremic CIED infection may be complicated by coincident NVE or remote-site infection (e.g., osteomyelitis). A 4- to 6-week course of endocarditis-targeted therapy is recommended for patients with CIED endocarditis and for those with bacteremia that continues during ongoing antimicrobial therapy after device removal. Although S. aureus bacteremia (and persistent CoNS bacteremia) in patients who have a CIED in place is likely—in the absence of another source—to reflect endocarditis and should be managed accordingly, not all bloodstream infections in these patients indicate endocarditis. If evidence suggesting endocarditis is lacking, bloodstream infection due to gram-negative bacilli, streptococci, enterococci, and Candida species may not indicate device infection. However, in the absence of another source, relapse after antimicrobial therapy increases the likelihood of CIED endocarditis and warrants treatment as such.
Outpatient Antimicrobial Therapy Fully compliant, clinically stable patients who are no longer bacteremic, are not febrile, and have no clinical or echocardiographic findings that suggest an impending complication may complete therapy as outpatients. Careful follow-up and a stable home setting are necessary, as are predictable IV access and use of antimicrobial agents that are stable in solution. Recommended regimens should not be compromised to accommodate outpatient therapy.
Monitoring Antimicrobial Therapy Measurement of the serum bactericidal titer—the highest dilution of the patient’s serum during therapy that kills 99.9% of the standard inoculum of the infecting organism—is not recommended for assessment of standard regimens but may be useful for assessment of the treatment of endocarditis caused by unusual organisms. Serum concentrations of aminoglycosides and vancomycin should be monitored and doses adjusted to avoid or address toxicity.
Antibiotic toxicities, including allergic reactions, occur in 25–40% of patients and commonly arise after several weeks of therapy. Blood tests to detect renal, hepatic, and hematologic toxicity should be performed periodically.
Blood cultures should be repeated daily until sterile in patients with endocarditis due to S. aureus or difficult-to-treat organisms, rechecked if there is recrudescent fever, and performed again 4–6 weeks after therapy to document cure. Blood cultures become sterile within 2 days after the start of appropriate therapy when infection is caused by viridans streptococci, enterococci, or HACEK organisms. In S. aureus endocarditis, β-lactam therapy results in sterile cultures in 3–5 days, whereas in MRSA endocarditis, positive cultures may persist for 7–9 days with vancomycin or daptomycin treatment. MRSA bacteremia persisting despite an adequate dosage of vancomycin may indicate infection due to a strain with reduced vancomycin susceptibility and therefore may point to a need for alternative therapy. When fever persists for 7 days despite appropriate antibiotic therapy, patients should be evaluated for paravalvular abscess, extracardiac abscesses (spleen, kidney), or complications (embolic events). Recrudescent fever raises the possibility of these complications but also of drug reactions or complications of hospitalization. Vegetations become smaller with effective therapy; however, 3 months after cure, 50% are unchanged and 25% are slightly larger.
SURGICAL TREATMENT
Intracardiac and central nervous system complications are important causes of morbidity and death due to infective endocarditis. In some cases, effective treatment for these complications requires surgery. The indications for cardiac surgical treatment of endocarditis (Table 155-5) have been derived from observational studies and expert opinion. The strength of individual indications varies; thus the risks and benefits as well as the timing of surgery must be individualized (Table 155-6). From 25% to 40% of patients with left-sided endocarditis undergo cardiac surgery during active infection, with slightly higher surgery rates for PVE than NVE. Intracardiac complications (which are most reliably detected by TEE) and CHF are the most commonly cited indications for surgery. The benefit of surgery has been assessed primarily in studies comparing populations of medically and surgically treated patients matched for the necessity of surgery (indications assessed in studies as propensity), with adjustments for predictors of death (comorbidities) and timing of the surgical intervention. Although study results vary, surgery for currently advised indications appears to convey a significant survival benefit (27–55%) that becomes apparent only with follow-up for ≥6 months. During the initial weeks after surgery, mortality risk may appear increased (disease + surgery–related mortality).
INDICATIONS FOR CARDIAC SURGICAL INTERVENTION IN PATIENTS WITH ENDOCARDITIS |
aSurgery must be carefully considered; findings are often combined with other indications to prompt surgery.
TIMING OF CARDIAC SURGICAL INTERVENTION IN PATIENTS WITH ENDOCARDITIS |
Indications • CONGESTIVE HEART FAILURE Moderate to severe refractory CHF caused by new or worsening valve dysfunction is the major indication for cardiac surgery. At 6 months of follow-up, patients with left-sided endocarditis and moderate to severe heart failure due to valve dysfunction who are treated medically have a 50% mortality rate, while among matched patients who undergo surgery the mortality rate is 15%. The survival benefit with surgery, which is most predictable among patients with the most weighty indications (propensity), is seen in both NVE and PVE. Surgery can relieve functional stenosis due to large vegetations or restore competence to damaged regurgitant valves by repair or replacement.
PERIVALVULAR INFECTION This complication, which is most common with aortic valve infection, occurs in 10–15% of native valve and 45–60% of prosthetic valve infections. It is suggested by persistent unexplained fever during appropriate therapy, new electrocardiographic conduction disturbances, or pericarditis. TEE with color Doppler is the test of choice to detect perivalvular abscesses (sensitivity, ≥85%). For optimal outcome, surgery is required, especially when fever persists, fistulae develop, prostheses are dehisced and unstable, or infection relapses after appropriate treatment. Cardiac rhythm must be monitored since high-grade heart block may require insertion of a pacemaker.
UNCONTROLLED INFECTION Continued positive blood cultures or otherwise-unexplained persistent fevers (in patients with either blood culture–positive or –negative endocarditis) despite optimal antibiotic therapy may reflect uncontrolled infection and may warrant surgery. Surgical treatment is also advised for endocarditis caused by organisms against which effective antimicrobial therapy is lacking (e.g., yeasts, fungi, P. aeruginosa, other highly resistant gram-negative bacilli, Brucella species).
S. AUREUS ENDOCARDITIS The mortality rate for S. aureus PVE exceeds 50% with medical treatment but is reduced to 25% with surgical treatment. In patients with intracardiac complications associated with S. aureus PVE, surgical treatment reduces the mortality rate twentyfold. Surgical treatment should be considered for patients with S. aureus native aortic or mitral valve infection who have TTE-demonstrable vegetations and remain septic during the initial week of therapy. Isolated tricuspid valve endocarditis, even with persistent fever, rarely requires surgery.
PREVENTION OF SYSTEMIC EMBOLI Death and persisting morbidity may result from cerebral or coronary artery emboli. Predicting a high risk of systemic embolization by echocardiographic determination of vegetation size and anatomy does not by itself identify those patients in whom surgery to prevent emboli will result in increased chances of survival. Net benefits from surgery to prevent emboli are most likely when other surgical benefits can be achieved simultaneously—e.g., repair of a moderately dysfunctional valve or debridement of a paravalvular abscess. Only 3.5% of patients undergo surgery solely to prevent systemic emboli. Valve repair, with the consequent avoidance of prosthesis insertion, improves the benefit-to-risk ratio of surgery performed to address vegetations.
CIED ENDOCARDITIS Removal of all hardware is recommended for patients with established CIED infection (pocket or intracardiac lead) or erosion of the device through the skin. Percutaneous lead extraction is preferred. With lead vegetations of >3 cm and the resulting risk of a pulmonary embolus or with retained hardware after attempted percutaneous extraction, surgical removal should be considered. Removal of the infected CIED during the initial hospitalization is associated with increased 30-day and 1-year survival rates over those attained with antibiotic therapy and device retention. If necessary, the CIED can be reimplanted percutaneously or surgically (epicardial leads) at a new site after at least 10–14 days of effective antimicrobial therapy. CIEDs should be removed and replaced subsequently when patients undergo valve surgery for endocarditis.
Timing of Cardiac Surgery With the more life-threatening indications for surgery (valve dysfunction and severe CHF, paravalvular abscess, major prosthesis dehiscence), early surgery—i.e., during the initial week of therapy—is associated with a greater chance of survival than later surgery. With less compelling indications, surgery may reasonably be delayed to allow further treatment as well as improvement in overall health (Table 155-6). After 14 days of recommended antibiotic therapy, excised valves are culture-negative in 99% and 50% of patients with streptococcal and S. aureus endocarditis, respectively. Recrudescent endocarditis on a new implanted prosthetic valve follows surgery for active NVE and PVE in 2% and 6–15% of patients, respectively. These frequencies do not justify the risk of an adverse outcome due to a delay in surgery, particularly in patients with severe heart failure, valve dysfunction, and uncontrolled staphylococcal infections. Delay is justified when infection is controlled and CHF is resolved with medical therapy.
Neurologic complications of endocarditis may be exacerbated as a consequence of cardiac surgery. The risk of neurologic deterioration is related to the type of neurologic complication and the interval between the complication and surgery. Whenever feasible, cardiac surgery should be delayed for 2–3 weeks after a nonhemorrhagic embolic infarction and for 4 weeks after a cerebral hemorrhage. A ruptured mycotic aneurysm should be treated before cardiac surgery.
Antibiotic Therapy after Cardiac Surgery Organisms have been detected on Gram’s stain—or their DNA has been detected by polymerase chain reaction—in excised valves from 45% of patients who have successfully completed the recommended therapy for endocarditis. In only 7% of these patients are the organisms, most of which are unusual and antibiotic resistant, cultured from the valve. Detection of organisms or their DNA does not necessarily indicate antibiotic failure; in fact, relapse of endocarditis after surgery is uncommon. Thus, when valve cultures are negative in uncomplicated NVE caused by susceptible organisms, the duration of preoperative plus postoperative treatment should equal the total duration of recommended therapy, with ~2 weeks of treatment administered after surgery. For endocarditis complicated by paravalvular abscess, partially treated PVE, or cases with culture-positive valves, a full course of therapy should be given postoperatively.
Extracardiac Complications Splenic abscess develops in 3–5% of patients with endocarditis. Effective therapy requires either image-guided percutaneous drainage or splenectomy. Mycotic aneurysms occur in 2–15% of endocarditis patients; one-half of these cases involve the cerebral arteries and present as headaches, focal neurologic symptoms, or hemorrhage. Cerebral aneurysms should be monitored by angiography. Some will resolve with effective antimicrobial therapy, but those that persist, enlarge, or leak should be treated surgically if possible. Extracerebral aneurysms present as local pain, a mass, local ischemia, or bleeding; these aneurysms are treated surgically.
OUTCOME
Factors that can adversely affect outcome include older age, severe comorbid conditions and diabetes, delayed diagnosis, involvement of prosthetic valves or the aortic valve, an invasive (S. aureus) or antibiotic-resistant (P. aeruginosa, yeast) pathogen, intracardiac and major neurologic complications, and an association with health care. Death and poor outcome often are related not to failure of antibiotic therapy but rather to the interactions of comorbidities and endocarditis-related end-organ complications. In developed countries, overall survival rates are 80–85%; however, rates vary considerably among subpopulations of endocarditis patients. Survival rates for patients with NVE caused by viridans streptococci, HACEK organisms, or enterococci (susceptible to synergistic therapy) are 85–90%. For S. aureus NVE in patients who do not inject drugs, survival rates are 55–70%, whereas 85–90% of injection drug users survive this infection. PVE beginning within 2 months of valve replacement results in mortality rates of 40–50%, whereas rates are only 10–20% in later-onset cases.
PREVENTION
To prevent endocarditis (long a goal in clinical practice), past expert committees have supported systemic antibiotic administration prior to many bacteremia-inducing procedures. A reappraisal of the evidence for antibiotic prophylaxis for endocarditis by the American Heart Association and the European Society of Cardiology culminated in guidelines advising its more restrictive use. At best, the benefit of antibiotic prophylaxis is minimal. Most endocarditis cases do not follow a procedure. Although dental treatments have been widely considered to predispose to endocarditis, such infection occurs no more frequently in patients who are undergoing dental treatment than in matched controls who are not. Furthermore, the frequency and magnitude of bacteremia associated with dental procedures and a routine day’s activities (e.g., tooth brushing and flossing) are similar; because dental procedures are infrequent events, exposure of cardiac structures to bacteremic oral-cavity organisms is notably greater from routine daily activities than from dental care. The relation of gastrointestinal and genitourinary procedures to subsequent endocarditis is even more tenuous than that of dental procedures. In addition, cost-effectiveness and cost-benefit estimates suggest that antibiotic prophylaxis represents a poor use of resources.
Nevertheless, studies in animal models suggest that antibiotic prophylaxis may be effective. Thus it is possible that rare cases of endocarditis are prevented. Weighing the potential benefits, potential adverse events, and costs associated with antibiotic prophylaxis, the American Heart Association and the European Society of Cardiology now recommend prophylactic antibiotics (Table 155-7) only for those patients at highest risk for severe morbidity or death from endocarditis (Table 155-8). Maintaining good dental hygiene is essential. Prophylaxis is recommended only when there is manipulation of gingival tissue or the periapical region of the teeth or perforation of the oral mucosa (including surgery on the respiratory tract). Prophylaxis is not advised for patients undergoing gastrointestinal or genitourinary tract procedures. High-risk patients should be treated before or when they undergo procedures on an infected genitourinary tract or on infected skin and soft tissue.
ANTIBIOTIC REGIMENS FOR PROPHYLAXIS OF ENDOCARDITIS IN ADULTS WITH HIGH-RISK CARDIAC LESIONSa,b |
aDosing for children: for amoxicillin, ampicillin, cephalexin, or cefadroxil, use 50 mg/kg PO; cefazolin, 25 mg/kg IV; clindamycin, 20 mg/kg PO or 25 mg/kg IV; clarithromycin, 15 mg/kg PO; and vancomycin, 20 mg/kg IV. bFor high-risk lesions, see Table 155-8. Prophylaxis is not advised for other lesions. cDo not use cephalosporins in patients with immediate hypersensitivity (urticaria, angioedema, anaphylaxis) to penicillin.
Source: Table created using the guidelines published by the American Heart Association and the European Society of Cardiology (W Wilson et al: Circulation 116:1736, 2007; and G Habib et al: Eur Heart J 30:2369, 2009).
HIGH-RISK CARDIAC LESIONS FOR WHICH ENDOCARDITIS PROPHYLAXIS IS ADVISED BEFORE DENTAL PROCEDURES |
aNot a target population for prophylaxis according to recommendations of the European Society for Cardiology.
Source: Table created using the guidelines published by the American Heart Association and the European Society of Cardiology (W Wilson et al: Circulation 116:1736, 2007; and G Habib et al: Eur Heart J 30:2369, 2009).
In patients with aortic or mitral valve regurgitation or a prosthetic valve, treatment of acute Q fever with doxycycline plus hydroxychloroquine (for doses, see Table 155-4) for 12 months is highly effective in preventing C. burnetii endocarditis.
The National Institute for Health and Clinical Excellence in the United Kingdom has advised discontinuation of all antibiotic prophylaxis for endocarditis. Limited surveillance studies have not detected increased viridans streptococcal endocarditis subsequent to the promulgation of guidelines that are more restrictive or advise no prophylaxis.
156 | Infections of the Skin, Muscles, and Soft Tissues |
Skin and soft tissue infections occur in all races, all ethnic groups, and all geographic locations, although some have unique geographic niches. In modern times, the frequency and severity of some skin and soft tissue infections have increased for several reasons. First, microbes are rapidly disseminated throughout the world via efficient air travel, acquiring genes for virulence factors and antibiotic resistance. Second, natural disasters, such as earthquakes, tsunamis, tornadoes, and hurricanes, appear to be increasing in frequency, and the injuries sustained during these events commonly cause major skin and soft-tissue damage that predisposes to infection. Third, trauma and casualties resulting from combat and terrorist activities can markedly damage or destroy tissues and provide both endogenous and exogenous pathogens with ready access to deeper structures. Unfortunately, because the marvels of modern medicine may not be available during human-instigated and natural disasters, primary treatment may be delayed and the likelihood of severe infection and death increased.
ANATOMIC RELATIONSHIPS: CLUES TO THE DIAGNOSIS OF SOFT TISSUE INFECTIONS
Skin and soft tissue infections have been common human afflictions for centuries. However, between 2000 and 2004, hospital admissions for skin and soft tissue infections rose by 27%, a remarkable increase that was attributable largely to the emergence of the USA300 clone of methicillin-resistant Staphylococcus aureus (MRSA). This chapter provides an anatomic approach to understanding the types of soft tissue infections and the diverse microbes responsible.
Protection against infection of the epidermis depends on the mechanical barrier afforded by the stratum corneum, since the epidermis itself is devoid of blood vessels (Fig. 156-1). Disruption of this layer by burns or bites, abrasions, foreign bodies, primary dermatologic disorders (e.g., herpes simplex, varicella, ecthyma gangrenosum), surgery, or vascular or pressure ulcer allows penetration of bacteria to the deeper structures. Similarly, the hair follicle can serve as a portal either for components of the normal flora (e.g., Staphylococcus) or for extrinsic bacteria (e.g., Pseudomonas in hot-tub folliculitis). Intracellular infection of the squamous epithelium with vesicle formation may arise from cutaneous inoculation, as in infection with herpes simplex virus (HSV) type 1; from the dermal capillary plexus, as in varicella and infections due to other viruses associated with viremia; or from cutaneous nerve roots, as in herpes zoster. Bacteria infecting the epidermis, such as Streptococcus pyogenes, may be translocated laterally to deeper structures via lymphatics, an event that results in the rapid superficial spread of erysipelas. Later, engorgement or obstruction of lymphatics causes flaccid edema of the epidermis, another characteristic of erysipelas.
FIGURE 156-1 Structural components of the skin and soft tissue, superficial infections, and infections of the deeper structures. The rich capillary network beneath the dermal papillae plays a key role in the localization of infection and in the development of the acute inflammatory reaction.
The rich plexus of capillaries beneath the dermal papillae provides nutrition to the stratum germinativum, and physiologic responses of this plexus produce important clinical signs and symptoms. For example, infective vasculitis of the plexus results in petechiae, Osler’s nodes, Janeway lesions, and palpable purpura, which, if present, are important clues to the existence of endocarditis (Chap. 155). In addition, metastatic infection within this plexus can result in cutaneous manifestations of disseminated fungal infection (Chap. 240), gonococcal infection (Chap. 181), Salmonella infection (Chap. 190), Pseudomonas infection (i.e., ecthyma gangrenosum; Chap. 189), meningococcemia (Chap. 180), and staphylococcal infection (Chap. 172). The plexus also provides bacteria with access to the circulation, thereby facilitating local spread or bacteremia. The postcapillary venules of this plexus are a prominent site of polymorphonuclear leukocyte sequestration, diapedesis, and chemotaxis to the site of cutaneous infection.
Amplification of these physiologic mechanisms by excessive levels of cytokines or bacterial toxins causes leukostasis, venous occlusion, and pitting edema. Edema with purple bullae, ecchymosis, and cutaneous anesthesia suggests loss of vascular integrity and necessitates exploration of the deeper structures for evidence of necrotizing fasciitis or myonecrosis. An early diagnosis requires a high level of suspicion in instances of unexplained fever and of pain and tenderness in the soft tissue, even in the absence of acute cutaneous inflammation.
Table 156-1 indicates the chapters in which the infections described below are discussed in greater detail. Many of these infections are illustrated in the chapters cited or in Chap. 25e (Atlas of Rashes Associated with Fever).
SKIN AND SOFT TISSUE INFECTIONS |
INFECTIONS ASSOCIATED WITH VESICLES
(Table 156-1) Vesicle formation due to infection is caused by viral proliferation within the epidermis. In varicella and variola, viremia precedes the onset of a diffuse centripetal rash that progresses from macules to vesicles, then to pustules, and finally to scabs over the course of 1–2 weeks. Vesicles of varicella have a “dewdrop” appearance and develop in crops randomly about the trunk, extremities, and face over 3–4 days. Herpes zoster occurs in a single dermatome; the appearance of vesicles is preceded by pain for several days. Zoster may occur in persons of any age but is most common among immunosuppressed individuals and elderly patients, whereas most cases of varicella occur in young children. Vesicles due to HSV are found on or around the lips (HSV-1) or genitals (HSV-2) but also may appear on the head and neck of young wrestlers (herpes gladiatorum) or on the digits of health care workers (herpetic whitlow). Recurrent herpes labialis (HSV-1) and herpes genitalis commonly follow primary infection. Coxsackievirus A16 characteristically causes vesicles on the hands, feet, and mouth of children. Orf is caused by a DNA virus related to smallpox virus and infects the fingers of individuals who work around goats and sheep. Molluscum contagiosum virus induces flaccid vesicles on the skin of healthy and immunocompromised individuals. Although variola (smallpox) in nature was eradicated as of 1977, postmillennial terrorist events have renewed interest in this devastating infection (Chap. 261e). Viremia beginning after an incubation period of 12 days is followed by a diffuse maculopapular rash, with rapid evolution to vesicles, pustules, and then scabs. Secondary cases can occur among close contacts.
Rickettsialpox begins after mite-bite inoculation of Rickettsia akari into the skin. A papule with a central vesicle evolves to form a 1- to 2.5-cm painless crusted black eschar with an erythematous halo and proximal adenopathy. While more common in the northeastern United States and the Ukraine in 1940–1950, rickettsialpox has recently been described in Ohio, Arizona, and Utah. Blistering dactylitis is a painful, vesicular, localized S. aureus or group A streptococcal infection of the pulps of the distal digits of the hands.
INFECTIONS ASSOCIATED WITH BULLAE
(Table 156-1) Staphylococcal scalded-skin syndrome (SSSS) in neonates is caused by a toxin (exfoliatin) from phage group II S. aureus. SSSS must be distinguished from toxic epidermal necrolysis (TEN), which occurs primarily in adults, is drug-induced, and is associated with a higher mortality rate. Punch biopsy with frozen section is useful in making this distinction since the cleavage plane is the stratum corneum in SSSS and the stratum germinativum in TEN (Fig. 156-1). Intravenous γ-globulin is a promising treatment for TEN. Necrotizing fasciitis and gas gangrene also induce bulla formation (see “Necrotizing Fasciitis,” below). Halophilic vibrio infection can be as aggressive and fulminant as necrotizing fasciitis; a helpful clue in its diagnosis is a history of exposure to waters of the Gulf of Mexico or the Atlantic seaboard or (in a patient with cirrhosis) the ingestion of raw seafood. The etiologic organism (Vibrio vulnificus) is highly susceptible to tetracycline.
INFECTIONS ASSOCIATED WITH CRUSTED LESIONS
(Table 156-1) Impetigo contagiosa is caused by S. pyogenes, and bullous impetigo is due to S. aureus. Both skin lesions may have an early bullous stage but then appear as thick crusts with a golden-brown color. Epidemics of impetigo caused by MRSA have been reported. Streptococcal lesions are most common among children 2–5 years of age, and epidemics may occur in settings of poor hygiene, particularly among children in lower socioeconomic settings in tropical climates. It is important to recognize impetigo contagiosa because of its relationship to poststreptococcal glomerulonephritis. Rheumatic fever is not a complication of skin infection caused by S. pyogenes. Superficial dermatophyte infection (ringworm) can occur on any skin surface, and skin scrapings with KOH staining are diagnostic. Primary infections with dimorphic fungi such as Blastomyces dermatitidis and Sporothrix schenckii can initially present as crusted skin lesions resembling ringworm. Disseminated infection with Coccidioides immitis can also involve the skin, and biopsy and culture should be performed on crusted lesions in patients from endemic areas. Crusted nodular lesions caused by Mycobacterium chelonei have been described in HIV-seropositive patients. Treatment with clarithromycin looks promising.
FOLLICULITIS
(Table 156-1) Hair follicles serve as portals for a number of bacteria, although S. aureus is the most common cause of localized folliculitis. Sebaceous glands empty into hair follicles and ducts and, if these portals are blocked, form sebaceous cysts that may resemble staphylococcal abscesses or may become secondarily infected. Infection of sweat glands (hidradenitis suppurativa) also can mimic infection of hair follicles, particularly in the axillae. Chronic folliculitis is uncommon except in acne vulgaris, where constituents of the normal flora (e.g., Propionibacterium acnes) may play a role.
Diffuse folliculitis occurs in two settings. Hot-tub folliculitis is caused by Pseudomonas aeruginosa in waters that are insufficiently chlorinated and maintained at temperatures of 37–40°C. Infection is usually self-limited, although bacteremia and shock have been reported. Swimmer’s itch occurs when a skin surface is exposed to water infested with freshwater avian schistosomes. Warm water temperatures and alkaline pH are suitable for mollusks that serve as intermediate hosts between birds and humans. Free-swimming schistosomal cercariae readily penetrate human hair follicles or pores but quickly die and elicit a brisk allergic reaction, causing intense itching and erythema.
PAPULAR AND NODULAR LESIONS
(Table 156-1) Raised lesions of the skin occur in many different forms. Mycobacterium marinum infections of the skin may present as cellulitis or as raised erythematous nodules. Similar lesions caused by Mycobacterium abscessus and M. chelonei have been described among patients undergoing cosmetic laser surgery and tattooing, respectively. Erythematous papules are early manifestations of cat-scratch disease (with lesions developing at the primary site of inoculation of Bartonella henselae) and bacillary angiomatosis (also caused by B. henselae). Raised serpiginous or linear eruptions are characteristic of cutaneous larva migrans, which is caused by burrowing larvae of dog or cat hookworms (Ancylostoma braziliense) and which humans acquire through contact with soil that has been contaminated with dog or cat feces. Similar burrowing raised lesions are present in dracunculiasis caused by migration of the adult female nematode Dracunculus medinensis. Nodules caused by Onchocerca volvulus measure 1–10 cm in diameter and occur mostly in persons bitten by Simulium flies in Africa. The nodules contain the adult worm encased in fibrous tissue. Migration of microfilariae into the eyes may result in blindness. Verruga peruana is caused by Bartonella bacilliformis, which is transmitted to humans by the sandfly Phlebotomus. This condition can take the form of single gigantic lesions (several centimeters in diameter) or multiple small lesions (several millimeters in diameter). Numerous subcutaneous nodules may also be present in cysticercosis caused by larvae of Taenia solium. Multiple erythematous papules develop in schistosomiasis; each represents a cercarial invasion site. Skin nodules as well as thickened subcutaneous tissue are prominent features of lepromatous leprosy. Large nodules or gummas are features of tertiary syphilis, whereas flat papulosquamous lesions are characteristic of secondary syphilis. Human papillomavirus may cause singular warts (verruca vulgaris) or multiple warts in the anogenital area (condylomata acuminata). The latter are major problems in HIV-infected individuals.
ULCERS WITH OR WITHOUT ESCHARS
(Table 156-1) Cutaneous anthrax begins as a pruritic papule, which develops within days into an ulcer with surrounding vesicles and edema and then into an enlarging ulcer with a black eschar. Cutaneous anthrax may cause chronic nonhealing ulcers with an overlying dirty-gray membrane, although lesions may also mimic psoriasis, eczema, or impetigo. Ulceroglandular tularemia may have associated ulcerated skin lesions with painful regional adenopathy. Although buboes are the major cutaneous manifestation of plague, ulcers with eschars, papules, or pustules are also present in 25% of cases.
Mycobacterium ulcerans typically causes chronic skin ulcers on the extremities of individuals living in the tropics. Mycobacterium leprae may be associated with cutaneous ulcerations in patients with lepromatous leprosy related to Lucio’s phenomenon, in which immune-mediated destruction of tissue bearing high concentrations of M. leprae bacilli occurs, usually several months after initiation of effective therapy. Mycobacterium tuberculosis also may cause ulcerations, papules, or erythematous macular lesions of the skin in both immunocompetent and immunocompromised patients.
Decubitus ulcers are due to tissue hypoxemia secondary to pressure-induced vascular insufficiency and may become secondarily infected with components of the skin and gastrointestinal flora, including anaerobes. Ulcerative lesions on the anterior shins may be due to pyoderma gangrenosum, which must be distinguished from similar lesions of infectious etiology by histologic evaluation of biopsy sites. Ulcerated lesions on the genitals may be either painful (chancroid) or painless (primary syphilis).
ERYSIPELAS
(Table 156-1) Erysipelas is due to S. pyogenes and is characterized by an abrupt onset of fiery-red swelling of the face or extremities. The distinctive features of erysipelas are well-defined indurated margins, particularly along the nasolabial fold; rapid progression; and intense pain. Flaccid bullae may develop during the second or third day of illness, but extension to deeper soft tissues is rare. Treatment with penicillin is effective; swelling may progress despite appropriate treatment, although fever, pain, and the intense red color diminish. Desquamation of the involved skin occurs 5–10 days into the illness. Infants and elderly adults are most commonly afflicted, and the severity of systemic toxicity varies.
CELLULITIS
(Table 156-1) Cellulitis is an acute inflammatory condition of the skin that is characterized by localized pain, erythema, swelling, and heat. It may be caused by indigenous flora colonizing the skin and appendages (e.g., S. aureus and S. pyogenes) or by a wide variety of exogenous bacteria. Because the exogenous bacteria involved in cellulitis occupy unique niches in nature, a thorough history (including epidemiologic data) provides important clues to etiology. When there is drainage, an open wound, or an obvious portal of entry, Gram’s stain and culture provide a definitive diagnosis. In the absence of these findings, the bacterial etiology of cellulitis is difficult to establish, and in some cases staphylococcal and streptococcal cellulitis may have similar features. Even with needle aspiration of the leading edge or a punch biopsy of the cellulitis tissue itself, cultures are positive in only 20% of cases. This observation suggests that relatively low numbers of bacteria may cause cellulitis and that the expanding area of erythema within the skin may be a direct effect of extracellular toxins or of the soluble mediators of inflammation elicited by the host.
Bacteria may gain access to the epidermis through cracks in the skin, abrasions, cuts, burns, insect bites, surgical incisions, and IV catheters. Cellulitis caused by S. aureus spreads from a central localized infection, such as an abscess, folliculitis, or an infected foreign body (e.g., a splinter, a prosthetic device, or an IV catheter). MRSA is rapidly replacing methicillin-sensitive S. aureus (MSSA) as a cause of cellulitis in both inpatient and outpatient settings. Cellulitis caused by MSSA or MRSA is usually associated with a focal infection, such as a furuncle, a carbuncle, a surgical wound, or an abscess; the U.S. Food and Drug Administration preferentially refers to these types of infection as purulent cellulitis. In contrast, cellulitis due to S. pyogenes is a more rapidly spreading, diffuse process that is frequently associated with lymphangitis and fever and should be referred to as nonpurulent cellulitis. Recurrent streptococcal cellulitis of the lower extremities may be caused by organisms of group A, C, or G in association with chronic venous stasis or with saphenous venectomy for coronary artery bypass surgery. Streptococci also cause recurrent cellulitis among patients with chronic lymphedema resulting from elephantiasis, lymph node dissection, or Milroy’s disease. Recurrent staphylococcal cutaneous infections are more common among individuals who have eosinophilia and elevated serum levels of IgE (Job’s syndrome) and among nasal carriers of staphylococci. Cellulitis caused by Streptococcus agalactiae (group B Streptococcus) occurs primarily in elderly patients and those with diabetes mellitus or peripheral vascular disease. Haemophilus influenzae typically causes periorbital cellulitis in children in association with sinusitis, otitis media, or epiglottitis. It is unclear whether this form of cellulitis will (like meningitis) become less common as a result of the impressive efficacy of the H. influenzae type b vaccine.
Many other bacteria also cause cellulitis. It is fortunate that these organisms occur in such characteristic settings that a good history provides useful clues to the diagnosis. Cellulitis associated with cat bites and, to a lesser degree, with dog bites is commonly caused by Pasteurella multocida, although in the latter case Staphylococcus intermedius and Capnocytophaga canimorsus also must be considered. Sites of cellulitis and abscesses associated with dog bites and human bites also contain a variety of anaerobic organisms, including Fusobacterium, Bacteroides, aerobic and anaerobic streptococci, and Eikenella corrodens. Pasteurella is notoriously resistant to dicloxacillin and nafcillin but is sensitive to all other β-lactam antimicrobial agents as well as to quinolones, tetracycline, and erythromycin. Ampicillin/clavulanate, ampicillin/sulbactam, and cefoxitin are good choices for the treatment of animal or human bite infections. Aeromonas hydrophila causes aggressive cellulitis in tissues surrounding lacerations sustained in freshwater (lakes, rivers, and streams). This organism remains sensitive to aminoglycosides, fluoroquinolones, chloramphenicol, trimethoprim-sulfamethoxazole, and third-generation cephalosporins; it is resistant to ampicillin, however.
P. aeruginosa causes three types of soft tissue infection: ecthyma gangrenosum in neutropenic patients, hot-tub folliculitis, and cellulitis following penetrating injury. Most commonly, P. aeruginosa is introduced into the deep tissues when a person steps on a nail. Treatment includes surgical inspection and drainage, particularly if the injury also involves bone or joint capsule. Choices for empirical treatment while antimicrobial susceptibility data are awaited include an aminoglycoside, a third-generation cephalosporin (ceftazidime, cefoperazone, or cefotaxime), a semisynthetic penicillin (ticarcillin, mezlocillin, or piperacillin), or a fluoroquinolone (although drugs of the last class are not indicated for the treatment of children <13 years old).
Gram-negative bacillary cellulitis, including that due to P. aeruginosa, is most common among hospitalized, immunocompromised hosts. Cultures and sensitivity tests are critically important in this setting because of multidrug resistance (Chap. 189).
The gram-positive aerobic rod Erysipelothrix rhusiopathiae is most often associated with fish and domestic swine and causes cellulitis primarily in bone renderers and fishmongers. E. rhusiopathiae remains susceptible to most β-lactam antibiotics (including penicillin), erythromycin, clindamycin, tetracycline, and cephalosporins but is resistant to sulfonamides, chloramphenicol, and vancomycin. Its resistance to vancomycin, which is unusual among gram-positive bacteria, is of potential clinical significance since this agent is sometimes used in empirical therapy for skin infection. Fish food containing the water flea Daphnia is sometimes contaminated with M. marinum, which can cause cellulitis or granulomas on skin surfaces exposed to the water in aquariums or injured in swimming pools. Rifampin plus ethambutol has been an effective therapeutic combination in some cases, although no comprehensive studies have been undertaken. In addition, some strains of M. marinum are susceptible to tetracycline or to trimethoprim-sulfamethoxazole.
NECROTIZING FASCIITIS
(Table 156-1) Necrotizing fasciitis, formerly called streptococcal gangrene, may be associated with group A Streptococcus or mixed aerobic–anaerobic bacteria or may occur as a component of gas gangrene caused by Clostridium perfringens. Strains of MRSA that produce the Panton-Valentine leukocidin (PVL) toxin have been reported to cause necrotizing fasciitis. Early diagnosis may be difficult when pain or unexplained fever is the only presenting manifestation. Swelling then develops and is followed by brawny edema and tenderness. With progression, dark-red induration of the epidermis appears, along with bullae filled with blue or purple fluid. Later the skin becomes friable and takes on a bluish, maroon, or black color. By this stage, thrombosis of blood vessels in the dermal papillae (Fig. 156-1) is extensive. Extension of infection to the level of the deep fascia causes this tissue to take on a brownish-gray appearance. Rapid spread occurs along fascial planes, through venous channels and lymphatics. Patients in the later stages are toxic and frequently manifest shock and multiorgan failure.
Necrotizing fasciitis caused by mixed aerobic-anaerobic bacteria begins with a breach in the integrity of a mucous membrane barrier, such as the mucosa of the gastrointestinal or genitourinary tract. The portal can be a malignancy, a diverticulum, a hemorrhoid, an anal fissure, or a urethral tear. Other predisposing factors include peripheral vascular disease, diabetes mellitus, surgery, and penetrating injury to the abdomen. Leakage into the perineal area results in a syndrome called Fournier’s gangrene, characterized by massive swelling of the scrotum and penis with extension into the perineum or the abdominal wall and the legs.
Necrotizing fasciitis caused by S. pyogenes has increased in frequency and severity since 1985. There are two distinct clinical presentations: those with no portal of entry and those with a defined portal of entry. Infections in the first category often begin deep at the site of a nonpenetrating minor trauma, such as a bruise or a muscle strain. Seeding of the site via transient bacteremia is likely, although most patients deny antecedent streptococcal infection. The affected patients present with only severe pain and fever. Late in the course, the classic signs of necrotizing fasciitis, such as purple (violaceous) bullae, skin sloughing, and progressive toxicity, develop. In infections of the second type, S. pyogenes may reach the deep fascia from a site of cutaneous infection or penetrating trauma. These patients have early signs of superficial skin infection with progression to necrotizing fasciitis. In either case, toxicity is severe, and renal impairment may precede the development of shock. In 20–40% of cases, myositis occurs concomitantly, and, as in gas gangrene (see below), serum creatine phosphokinase levels may be markedly elevated. Necrotizing fasciitis due to mixed aerobic-anaerobic bacteria may be associated with gas in deep tissue, but gas usually is not present when the cause is S. pyogenes or MRSA. Prompt surgical exploration down to the deep fascia and muscle is essential. Necrotic tissue must be surgically removed, and Gram’s staining and culture of excised tissue are useful in establishing whether group A streptococci, mixed aerobic-anaerobic bacteria, MRSA, or Clostridium species are present (see “Treatment,” below).
MYOSITIS AND MYONECROSIS
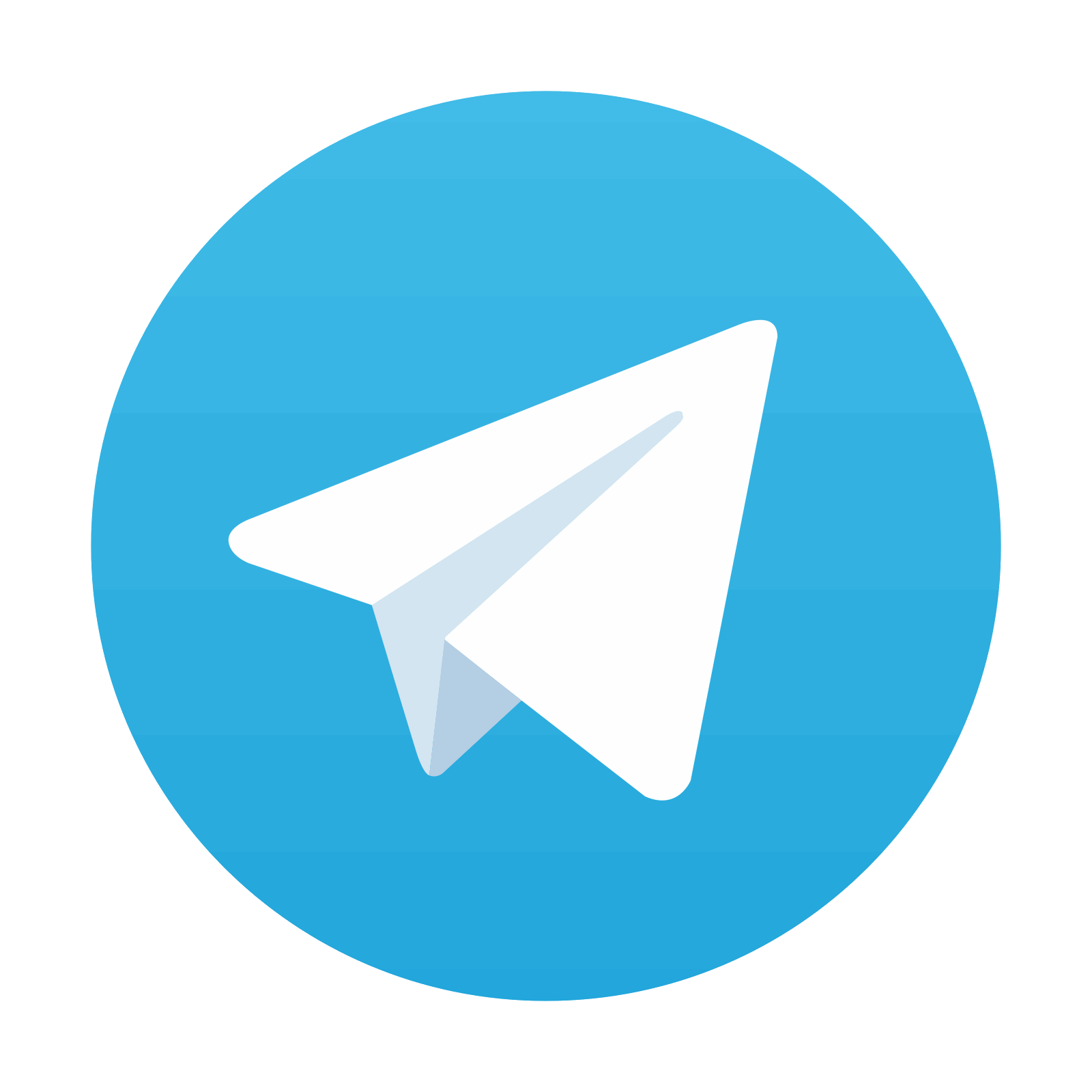
Stay updated, free articles. Join our Telegram channel

Full access? Get Clinical Tree
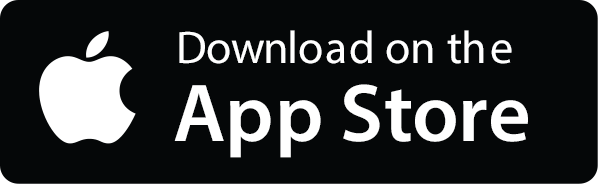
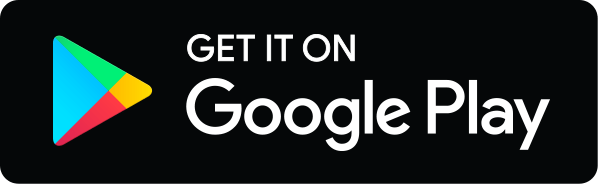