Cynthia Spry
Infection Prevention and Control
Advancements in surgical interventions and the science of infection prevention and control continue to evolve and have become increasingly complex. The complexity is derived from the changes in the healthcare environment, the advancements in minimally invasive and advanced surgical procedures, and the development of new, highly technical instrumentation and equipment. This chapter provides an overview of the etiology of infection, including emerging drug-resistant bacteria, and identifies various methods to control infection in the perioperative environment. Use of Standard Precautions along with engineering and work-practice controls assists perioperative practitioners in reducing the transmission of pathogenic organisms. Perioperative patient care is based on surgical aseptic principles. Careful adherence to these principles supports infection prevention and control, ultimately improving surgical patient safety and outcomes. Each member of the surgical team must demonstrate the highest integrity in the application of this knowledge. Finally, the potential for bioterrorism has forced the United States along with the rest of the world to strengthen security and emergency readiness. If a bioterrorist attack resulted in massive exposure or illness, perioperative personnel could and would be mobilized to serve in a variety of locations. Perioperative personnel need to be familiar with the most likely biologic weapons and the levels of precautions required for patient and personnel safety.
Causes of Infection
The human body has three lines of defense to combat infection. The first line of defense consists of external barriers, such as the skin and mucous membranes, which are usually impervious to most pathogenic organisms. The second line of defense is the inflammatory response, which prevents an invading pathogen from reproducing and possibly involving other tissue. The third line of defense, the immune response, is triggered after the inflammatory response. When a break in this defense mechanism occurs, the possibility for infection increases (Box 4-1 lists term definitions).
Microorganisms That Cause Infection
Microorganisms are living organisms that are too small to be seen with the naked eye. These organisms include bacteria, fungi, protozoa, algae, and viruses. Microorganisms are classified to determine appropriate treatment for an infection. Each organism is assigned two names; the genus is the first name, and the specific epithet (species) is the second. Bacteria are further described by shape, motility, growth in aerobic or anaerobic environments, and reaction to staining processes (Figure 4-1). Scientific names can be assigned to organisms in various ways. Staphylococcus aureus is a microorganism commonly found on the skin. Staphylo describes the clustered arrangement of the cells; coccus (Greek kokkos, “berry”) indicates that they are shaped like spheres. The species aureus is Latin for “golden,” the color of the colonies of the bacterium. Bacteria cause most surgical site infections (SSIs). Gram-positive cocci, as a group, are the most common cause of SSIs. The organisms most commonly found in postoperative SSIs include staphylococcal, enterococcal, pseudomonal, and streptococcal species. S. aureus is the most frequently identified organism.
Staphylococci.
Staphylococci are gram-positive cocci. They are facultative anaerobes but grow best under aerobic conditions. Staphylococci can be found in the indigenous flora of the skin and mucous membranes of the nasopharynx, urethra, and vagina. They are resistant to drying, heat, and high salt concentrations.
The two recognized species of staphylococci are S. aureus and S. epidermidis. Staphylococci are called coagulase positive when they are capable of clotting plasma and coagulase negative when the plasma clumps them. Coagulase-positive staphylococci are more virulent or pathogenic than coagulase-negative staphylococci. S. aureus is hemolytic, parasitic, pathogenic, and coagulase positive. S. epidermidis is parasitic, less pathogenic, and coagulase negative. S. aureus is a long-recognized cause of surgical site infections. More recently, S. epidermidis has been implicated in infections of prosthetic devices and indwelling medical devices (CDC, 2008a; Geipl 2009; Widerstrom et al, 2012). The skin surface is the most common site of S. epidermidis. Approximately 30% to 70% of individuals carry staphylococci on their skin. This can lead to contamination of clothing and dispersal of the microorganisms. For no known reason individuals who are skin carriers of staphylococci differ in the rate at which they shed the microorganisms. There is no obvious difference in hygiene and skin condition between light and heavy shedders, and no other contributing factor is apparent. Heavy shedders seem to be in normal good health.
S. aureus infections in hospitals can lead to prolonged hospital stays and may result in death. S. aureus is a medically important human pathogen that is found in the nares of approximately one third of the population (Pynnonen et al, 2011). Human nasal and throat cavities are the most important reservoirs that continually replenish the external environment. Among perioperative personnel, S. aureus has been found most commonly in the respiratory passages. The potential for patient infection increases greatly as the personnel carrier rate increases. Nasal carriers also may be skin carriers. Carriers usually harbor either coagulase-positive (pathogenic) or coagulase-negative (nonpathogenic) staphylococci; seldom are there both types, and rarely more than one strain is identified. Because an individual may be a carrier of staphylococci one day and a noncarrier the next, frequent swab testing of the nose as an infection control measure is impractical. Staphylococci survive for long periods in the air, dust, debris, bedding, and clothing. Pathogenic staphylococci grow in the sweat, urine, and tissue and on the human skin. They are more difficult to destroy than many other non–spore-forming organisms. Cleanliness of the environment; proper handling, and, when appropriate, sterilization of linens and equipment; and adherence to adequate hand hygiene practices are important controls to prevent transmission of infection (AORN, 2013a).
Enterococci.
Enterococci are gram-positive organisms that are found in the normal flora of the gastrointestinal and female genital tracts. These organisms are responsible for many serious healthcare-associated infections, including surgical site infections, bacterial endocarditis, septicemia, and urinary tract infections (UTIs). Enterococci also have been implicated in polymicrobial wound infections (CDC, 2007a; Scheithauer et al, 2012). Enterococcal infections are most often healthcare associated and seen in patients with comorbid conditions.
Pseudomonads.
The most common aerobic species of pseudomonads pathogenic to humans is Pseudomonas aeruginosa. It thrives in moist environments and is found frequently in soil, water, sewage, debris, and air. It also can be found in the normal flora of the skin and intestines. P. aeruginosa was considered a microorganism of slight pathogenic power but is now known to be associated with many infections in humans, including surgical site infections. P. aeruginosa seems to be pathogenic only when it is introduced into areas where normal defenses are absent, when it is superimposed on staphylococcal infection, or when it is present in a mixed infection. It may attack a debilitated patient who has extensive burns or major trauma. The organism is often seen in critical care and burn units. P. aeruginosa is resistant to most antimicrobial agents. Other species of pseudomonads have been associated with infections caused by contaminated intravascular cannulae (as from the use of pressure transducers) or by the lumens of equipment (such as endoscopes used in gastrointestinal [GI] studies). An important preventive measure is proper environmental sanitation and strict adherence to aseptic technique.
Streptococci.
Most streptococci are gram-positive, non–spore-forming facultative microorganisms. They are normally found in the indigenous flora of the upper respiratory, genitourinary, and GI tracts. Streptococci are classified as alpha, beta, or gamma according to chemical factors, biochemical tests, and their action on red blood cells.
Group A streptococci account for most streptococcal infections in humans. Known as the flesh-eating bacteria because of their ability to cause necrotizing fasciitis, group A streptococci are of concern because of the sporadic and deadly outbreaks of community-acquired and healthcare-associated infection (CDC, 2006a; Ramirez et al, 2011). An example is Streptococcus pyogenes, which is responsible for most soft tissue infections, otitis media, pharyngitis, impetigo, septicemia, and surgical site infections. Virulent streptococci are more serious invaders than staphylococci. Streptococci tend to involve wide areas of tissue and cause necrosis without localization. Streptococci are usually sensitive to penicillin, whereas staphylococci may not be. Streptococci also occur in mixed infections with other pathogens.
In surgical wounds, streptococci can be introduced into the incision and spread via the lymph vessels and nodes. This distribution can result in inflammation and cellulitis. Streptococcal transmission occurs by droplet transmission and by contamination of the environment. Inhalation of infectious droplets expelled from the nose and mouth of an infected individual is considered direct contact. Indirect contact occurs when infected air and dust from the environment enter a susceptible host. Group A streptococcus can be carried in the nasal passages, anus, or vagina. The upper respiratory tract is not a significant reservoir for microorganisms that cause surgical site infections except in the presence of an acute upper respiratory tract infection. In several randomized controlled studies, it was found that surgical wound infection rates are unaffected by the use or nonuse of surgical masks by noninfected individuals during the operative procedure. It is not always possible to know who might be infected, and the use of masks during operative procedures continues to be strongly recommended in the prevention of SSIs. Most bacteria in the operating room (OR) environment are shed from the skin of perioperative personnel (AORN, 2013b).
Clostridia.
The clostridia bacteria are spore-forming, anaerobic, gram-positive bacilli that produce virulent toxins. Clostridium difficile is a bacterium in the Clostridium genus, which also includes C. perfringens (gas gangrene), C. tetani (tetanus), and C. botulinum (botulism). C. difficile is an anaerobic, gram-positive, spore-forming bacillus. C. difficile is an overgrowth in the colon that can manifest symptoms of diarrhea, colitis, toxic megacolon, dehydration, colonic perforation, and death. The overgrowth of C. difficile in the colon usually results from alterations in the normal flora of the colon that are associated with the use of antibiotics (CDC, 2006a; Gould et al, 2008). For this organism to cause disease, C. difficile must already be in the GI system. Subsequently, there must be a change in the normal flora of the colon to allow the organism to grow and produce toxins. The two toxins produced are toxin A, which is an enterotoxin causing excretion of large amounts of fluid from the bowel, and toxin B, which is cytotoxic. Toxin B attacks and disintegrates cells of the intestines. Additionally, C. difficile produces tissue-degrading enzymes, which produce an inflammatory response within the colon that can result in a spectrum of disease entities.
In its spore form, C. difficile can withstand drying and heat and may be resistant to many disinfectants. The spores can survive up to 5 months in the environment. C. difficile can be transmitted between individuals and by touching objects contaminated with the organism. In the healthcare environment, C. difficile has been cultured in rooms of infected individuals 40 days after discharge. C. difficile also has been cultured from healthcare workers’ shoes, fingernails, fingertips, and the underside of rings (Mitchum, 2009).
Several interventions can assist in the prevention of C. difficile transmission in the healthcare environment, including following Contact Precautions, handwashing with antimicrobial soap and water, using personal protective equipment (PPE), cleaning and disinfecting all surfaces and equipment, and cleaning and disinfecting reusable devices in the perioperative suite. The use of disposable equipment only is recommended for infected patients (CDC, 2010a). Additionally, clinical practice guidelines from the Society for Healthcare Epidemiology of America (SHEA) and the Infectious Diseases Society of America (IDSA) recommend that in a setting in which there is an outbreak or an increased rate of C. difficile infection to instruct visitors and healthcare workers caring for patients who are infected with C. difficile to wash their hands with soap, or antimicrobial soap and water. Further, they recommend that the frequency and duration of antimicrobial therapy be minimized and to implement an antimicrobial stewardship program (Cohen et al, 2010).
Mycobacterium Tuberculosis.
M. tuberculosis is a non–spore-forming, nonmotile, aerobic bacillus. These bacilli can infect almost any tissue—skin, bones, kidney, lymph nodes, intestinal tract, and fallopian tubes—and cause tuberculosis (TB). Tubercle bacilli spread in the host through the lymphatic channels and bloodstream and by way of the alveoli and GI tract. Tuberculosis is responsible for more than 3 million deaths a year and is the most opportunistic infection associated with human immunodeficiency virus (HIV) (Neil, 2008).
Tubercle bacilli are transmitted directly by means of discharge from the respiratory tract (e.g., by inhalation of droplets expelled during coughing or kissing) and, less frequently, through the digestive tract. M. tuberculosis is carried via airborne droplet nuclei when infected people sneeze, cough, or speak. These nuclei particles are less than 5 micrometers in size and contain up to three bacteria, allowing them to be kept airborne for a prolonged period (CDC, 2006a). Infection occurs when individuals inhale these infected droplet nuclei. The droplet nuclei travel through the nasal passages, upper respiratory tract, and bronchi to reach the lung alveoli, where they are engulfed by macrophages and spread throughout the body. Symptoms of TB are dependent on the part of the body infected, but may be vague at the onset and include fever, fatigue, loss of appetite, cough, fever, and night sweats.
Generally 2 to 10 weeks after initial infection, an immune response limits additional multiplication and spread of the bacilli. Some of the bacilli can remain dormant for many years, however, a condition referred to as latent tuberculosis infection. Individuals with latent TB usually have positive purified protein derivative (PPD) results, but they exhibit none of the symptoms of active TB and are considered noninfectious. The probability that a person who is exposed to M. tuberculosis will become infected depends on the concentration of infectious droplet nuclei and the duration of exposure. When infected, an individual has approximately a 10% risk of developing active TB in his or her lifetime. The risk is greatest during the first 2 years after initial exposure. In individuals with compromised immune systems, there is a higher risk that latent TB will progress into active TB (CDC, 2006a). Latent TB is treated with isoniazid or rifampin. Active TB is treated with a combination of medications (e.g., isoniazid, rifampin, ethambutol, pyrazinamide) (Neil, 2008).
Viruses.
Viruses are classified as small particles rather than living cells because viruses have no metabolic activity and must receive all sustenance for survival from a host cell. Viral pathogens are transmitted via the oral and respiratory tracts (e.g., poxvirus, rhinovirus), the intestinal and urinary tracts (e.g., poliovirus, hepatitis A virus, hepatitis E virus), and the genital tract (e.g., herpes simplex 2, HIV) and through blood and some blood products (e.g., HIV, hepatitis B virus, hepatitis C virus, hepatitis D virus, and others). Some viruses have multiple routes of transmission.
When a virus invades a host cell, it combines with the host cell’s nucleic acid (deoxyribonucleic acid [DNA] or ribonucleic acid [RNA]) and reprograms the host cell metabolism to accommodate virus replication. Virus replication stimulates the antibody defense in the host. The presence of viruses may be detected by identifying the virus-specific antibodies that are produced by the infected individual’s immune system, by detecting the antigens elaborated by the virus and present in the blood, or by growing a culture of the virus itself. Detection of virus-specific antibodies or antigens is termed seropositivity, or seroconversion. Viruses are susceptible to destruction by high-level disinfection—a process that destroys most disease-producing microorganisms.
Hepatitis.
Hepatitis is defined as a widespread inflammation of the liver, often caused by a virus. It can be acute or chronic. Symptoms include hepatomegaly, right upper quadrant abdominal pain, and jaundice. Hepatitis is a frequently reported viral disease in the United States, with six identified strains (A through E and G).
Hepatitis A virus (HAV) is the causative agent of what is referred to as infectious hepatitis. HAV is spread through the fecal-oral route and can be prevented by proper hand hygiene. It is very common in underdeveloped countries with poor sanitation practices. HAV constitutes slightly less than half of all hepatitis cases reported in the United States and usually has an uneventful course and recovery. Immunization against HAV is available and has helped decrease the incidence of this strain of hepatitis.
Hepatitis B virus (HBV) is the causative agent for what is sometimes referred to as serum hepatitis. This worldwide virus has reached near-epidemic levels but is preventable via vaccination and the strict use of Universal Precautions. HBV can be transmitted by blood or body fluids, such as serum, saliva, semen, and vaginal fluids. It has an incubation period of 6 weeks to 4 months, depending on the type and amount of exposure. Almost 30% of infected individuals are asymptomatic; individuals with symptoms experience fatigue, abdominal pain, nausea, vomiting, and jaundice. Chronic hepatitis infection leads to progressive liver disease and possible death and is a leading cause of liver transplants. Most adults with HBV recover without incidence and develop immunity, but a small percentage may not develop immunity and will become carriers of the virus. HBV vaccine is recommended, by government regulation, for healthcare workers who may be exposed to blood and body fluids. Postexposure prophylaxis for percutaneous or permucosal exposure to HBV depends on the vaccination status of the healthcare worker. The risk of a susceptible person developing clinical HBV ranges from 1% to 31%, depending on the source person’s HBV status (Davenport and Myers, 2009). The Occupational Safety and Health Administration (OSHA) mandates that all healthcare workers report blood and body fluid exposures, and perioperative personnel should follow the institutional protocol for exposure reporting.
Hepatitis C virus (HCV) transmission is most often associated with direct exposure to blood (e.g., via intravenous or subcutaneous routes) or other infectious materials. The average incubation period is 7 weeks. Currently there is no vaccine for the prevention of HCV. Individuals who contract HCV infection are prone to develop chronic infection. A high proportion of this population is asymptomatic and develops chronic liver disease, such as cirrhosis, hepatocellular carcinoma, and portal hypertension. As with HBV, a small proportion of individuals infected with HCV die. Prevention of HCV requires strict use of Universal Precautions, implementation of and adherence to sharps safety programs, compliance with exposure reporting protocols, and completion of a postexposure treatment plan. It is crucial that all perioperative personnel be educated regarding the risk for and prevention of all bloodborne infections, including HCV. These education programs should be conducted routinely and updated for accurate information inclusive of the Centers for Disease Control and Prevention (CDC) guidelines. The risk of HCV transmission after percutaneous exposure is about 1.8% (Davenport and Myers, 2009).
Hepatitis D virus (HDV) requires the coinfection of HBV. HDV is transmitted by blood and body fluids. The incubation period is generally 14 to 56 days. Individuals immunized against HBV have protection against contracting HDV. HDV is suspected when individuals present with HBV superinfection. Treatment is supportive for acute infections. With chronic HDV infections, antiviral therapies and liver transplants may be required.
Hepatitis E virus (HEV) is spread by the fecal-oral route. HEV is similar to HAV in the transmission and disease process. Most outbreaks have occurred in developing countries, where fecally contaminated water is found to be the source. The incubation period is 15 to 64 days. No vaccine is available for the prevention of HEV. Prevention is similar to that for HAV, with the use of proper hand hygiene. Treatment for HEV is supportive and the infection generally resolves on its own. The prevalence of HEV in the United States is low.
Hepatitis G virus is a bloodborne pathogen, with a comparable rate of infection as that of HCV in units of banked blood. It is spread in a manner similar to HBV and HCV dissemination, by percutaneous or permucosal exposure. There is no vaccine to prevent hepatitis G virus infection. Similar to HBV and HCV, prudent and consistent use of barrier protection (e.g., Standard Precautions) is recommended. There are no concrete data at present on the chronic nature of this viral disease (CDC, 2006a).
Human Immunodeficiency Virus.
HIV is a retrovirus (e.g., a virus that carries genetic information in the RNA instead of the DNA) that attacks the immune system by destroying T-helper lymphocytes. Healthy individuals generally have 800 to 1000 T-helper cells/mm3 in their blood. Infection with HIV reduces these numbers, and when a person is HIV positive and his or her T-cell count falls below 200 cells/mm3 (or the person is HIV positive and is diagnosed with an atypical or opportunistic infection and/or cancer), a diagnosis of acquired immunodeficiency syndrome (AIDS) is made (Sampson and Workman, 2013). The period from HIV exposure to actual development of AIDS has been reported to be 12 years or longer. During this time the individual is a carrier of the virus. HIV has been isolated from all body fluids (e.g., blood, semen, vaginal secretions, saliva, tears, breast milk, cerebrospinal fluid, amniotic fluid, and urine) of infected individuals. Symptoms that result from HIV infection may be attributed to other causes and include fever, night sweats, chills, muscle aches, and headache.
Occupational exposure to HIV can occur by percutaneous injury (e.g., a needlestick or cut with a sharp instrument) or by mucous membrane or nonintact skin (e.g., exposed skin that is abraded) exposure to blood, tissue, or other body fluids that are potentially infectious. The CDC estimates that there is an average transmission rate of 0.3% per sharps injury (CDC, 2006b). Mucous membrane exposure is associated with a 0.09% transmission risk, and exposure through nonintact skin is probably lower (Davenport and Myers, 2009). If exposure occurs, appropriate postexposure management should take place as a part of the workplace safety program. The source patient should be informed of the incident. Serology testing should be done according to institutional policy and governmental requirements. Policies should be established for instances in which source patient consent cannot be obtained. The healthcare worker should be counseled about the risk of infection, and he or she should be evaluated clinically and serologically for evidence of HIV infection as soon as possible after exposure. Postexposure prophylaxis (PEP) regimens (Table 4-1) should be supervised by an expert in the management of exposures; follow-up should be provided for adherence to PEP; and adverse events, including seroconversion, should be monitored. Timely postexposure management and administration is important, and an exposure incident should be treated as an urgent medical condition.
TABLE 4-1
Postexposure Prophylaxis (PEP) for Occupational HIV Exposure*
Basic PEP | Expanded PEP |
Two drugs: zidovudine plus lamivudine or emtricitabine; or stavudine plus lamivudine or emtricitabine; or lopinavir/ritonavir | Three or more drugs: zidovudine plus lamivudine or emtricitabine plus lopinavir/ritonavir; or stavudine plus lamivudine or emtricitabine plus lopinavir/ritonavir; or tenofovir plus lamivudine or emtricitabine plus lopinavir/ritonavir |
Small-volume (few drops) exposure to mucous membranes or nonintact skin Source patient is HIV positive, asymptomatic with known low viral load Source patient is HIV positive, symptomatic or AIDS with high viral load | Large-volume (major splash) exposure to mucous membranes or nonintact skin Source patient is HIV positive, symptomatic or AIDS with high viral load |
Large-volume (major splash) exposure to mucous membranes or nonintact skin Source patient is HIV positive, asymptomatic with known low viral load Optional when source patient is unknown or known with HIV factors | Less severe percutaneous injury (solid needle or superficial injury) Source patient is HIV positive, symptomatic or AIDS with high viral load |
Less severe percutaneous injury (solid needle or superficial injury) Source patient is HIV positive, asymptomatic with known low viral load Optional when source patient is unknown or known with HIV factors | More severe percutaneous injury (large-bore hollow needle, deep punctures, visible blood on device, needle was in patient’s artery or vein) Source patient is HIV positive, asymptomatic with known low viral load Source patient is HIV positive, symptomatic or AIDS with high viral load |
AIDS, Acquired immunodeficiency syndrome; HIV, human immunodeficiency virus.
*PEP should be supported by policies and procedures that include sharps management, exposure documentation, and a procedure for staff members to follow if a percutaneous needlestick occurs. The key to any PEP plan is for the staff member to be able to undergo assessment as soon as possible after exposure so that if the administration of antiretroviral medication is recommended that the staff member is able to begin taking them before 72 hours to prevent the virus from rapidly replicating within the body. Medications need to be taken for 28 days.
Modified from Sampson JG, Workman ML: Care of patients with HIV disease and other immune deficiencies. In Ignatavicius DD, Workman ML, editors: Medical-surgical nursing: patient-centered collaborative care, ed 7, St Louis, 2013, Saunders.
Multidrug-Resistant Organism Infections: Emerging and Resurging Organisms
Historical Perspective.
The first antibiotics, sulfonamides, were introduced in 1935 to treat staphylococcal and streptococcal infections. Penicillin was introduced in the 1940s during World War II, followed by the discovery of streptomycin in the mid- to late 1940s. Although the introduction of these medications was viewed as miraculous and did save many lives, in the mid-1940s strains of previously susceptible microorganisms began showing resistance to some of these medications. During the 1960s, cephalosporin and semisynthetic penicillins were developed, and it was believed that the problem of healthcare-associated infections was solved. Many gram-negative bacteria were found to be susceptible to these new drugs. By the late 1970s, however, strains of S. aureus resistant to penicillin, methicillin, cephalosporins, aminoglycosides, clindamycin, erythromycin, and other antibiotics were isolated in hospital outbreaks of infection. This situation led to the development of new antibiotics, including carbapenems, cephamycins, and fluoroquinolones. These broad-spectrum antimicrobials were bactericidal at low concentrations and led to a false sense of euphoria during the 1980s, when it was believed that, at last, resistance to these antimicrobials would be impossible (Sandlin, 2008).
In the 1990s it became evident that the potential for resistance to any and all antimicrobials existed as healthcare-associated outbreaks of multidrug-resistant tuberculosis (MDR-TB) occurred. Significant outbreaks of methicillin-resistant Staphylococcus aureus (MRSA) (Surgical Pharmacology) and vancomycin-resistant Enterococcus (VRE) also occurred in the 1990s. More recently Klebsiella, a gram-negative bacteria associated with surgical site infection, has shown resistance to the carbapenems (CDC, 2010b). In some cases, organisms seemed to acquire resistance almost immediately on exposure to the particular antibiotic. As fewer new antimicrobial drugs are being developed by the pharmaceutical industry, many consider the 1990s to be the beginning of the postantibiotic era (Sandlin, 2008) (see Surgical Pharmacology).
Surgical Pharmacology
Treating Methicillin-Resistant Staphylococcus aureus (MRSA)
In the past, vancomycin was the only alternative for treating MRSA. With the increased use of vancomycin, however, strains of S. aureus with reduced susceptibility to vancomycin began to appear. Newer antibiotics include daptomycin, linezolid, teicoplanin, and quinupristin-dalfopristin. |
Modified from http://online.epocrates.com. Accessed December 13, 2012; http://www.nhs.uk/medicine-guides/pages/MedicineSideEffects.aspx?condition=Bacterialinfections&medicine=Teicoplanin&preparation=. Accessed December 13, 2012.
Mechanisms of Resistance.
Similar to their unwilling human hosts, pathogenic bacteria have an instinct to survive. When faced with an antimicrobial attack, they assemble their defensive resources. These microorganisms have the remarkable capability to incapacitate the threatening antibiotic and to mutate and outwit the most lethal clinical weapons. Although carrying only a single chromosome, bacteria by nature have extra minichromosomes called plasmids. These plasmids are hearty, and some may survive even the most aggressive antibiotic attack. Surviving plasmids are resistant and reproduce in kind, creating dominant organisms within the host. In addition, incompletely or ineffectively treated infections create the potential for reinfection with resistant organisms (CDC, 2006a).
Microbial resistance can be divided into three categories: (1) presence of a naturally resistant strain of an organism before any drugs are administered, (2) acquisition of a drug-resistant strain from an external source, and (3) drug resistance from treatment-related causes. Occurrence of naturally resistant strains of organisms without any exposure to drugs can occur by intrinsic resistance, genetic mutation, or transfer of genetic material. Some microorganisms possess genes that make them resistant to an antibiotic. These genes may always be present in the microorganism but remain in an inactive stage until challenged by an antibiotic. Genetic mutation can occur spontaneously in the course of rapid multiplication of the microbe. These antibiotic-resistant mutants reproduce within the host. New genetic material also can be transferred into bacteria by means of free DNA that contains resistant genes (CDC, 2006a).
Introduction of drug-resistant microorganisms can occur through a person or an inanimate object. Bacteria become mobile and accessible to humans on the hands or clothing of care providers, through instruments and procedures, or through food. Resistant microorganisms may travel from distant parts of the world by means of infected travelers. Surgical instruments that have been ineffectively cleaned and processed can contribute to the spread of resistant organisms. Use of antibiotics in agriculture and in animal feed products also has contributed to the development of resistant organisms. When the food is ingested, resistant genes may be spread to humans (CDC, 2006a).
Drug resistance from treatment-related causes is often the result of misuse (e.g., incorrect use, overuse, or underuse) of antibiotics. It is believed that 50% of all antibiotic use in the United States can be characterized by misuse in one form or another, and efforts to reduce surgical site infections include appropriate prophylactic antibiotic use in surgical patients (Evidence for Practice). It is estimated that half of all antibiotic prescriptions written are not warranted. During antibiotic therapy, the patient may have had a few resistant organisms. By natural selection, as the susceptible organisms are killed, the resistant organisms multiply and become predominant. Inadequate drug therapy may contribute to the phenomenon and may be the fault of the patient, the provider, or both. Failure to perform sensitivity testing along with inappropriate dosing can contribute to resistance. If the patient is noncompliant with the prescribed regimen or discontinues the drug prematurely, he or she may also be contributing to drug resistance (CDC, 2012a).
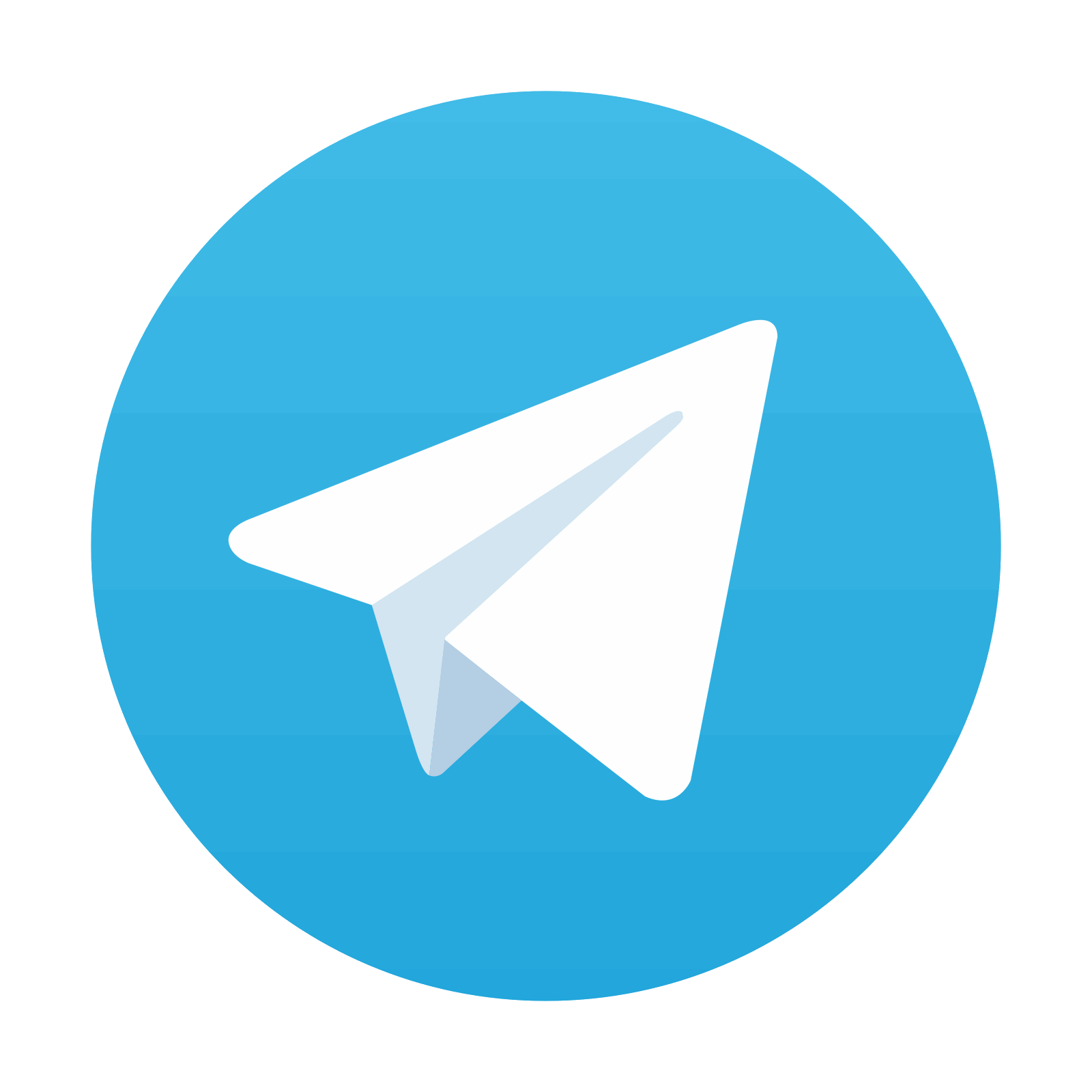
Stay updated, free articles. Join our Telegram channel

Full access? Get Clinical Tree
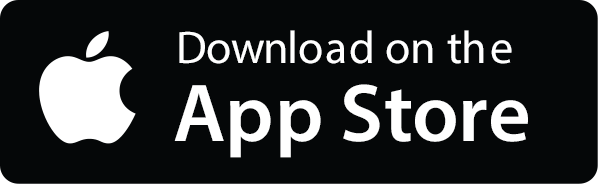
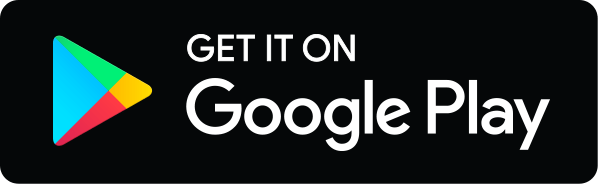