26 Robbie Kerr Following the pioneering work of Ludwig and Ringer almost 150 years ago, the first tissue culture media were developed from simple salts solutions based on the chemical properties of blood serum and were able to support the beating frog heart in vitro (Ringer 1882; Ringer 1883). This simple medium was composed of sodium chloride (NaCl), potassium chloride (KCl), calcium chloride (CaCl2), and sodium bicarbonate (NaHCO3). Known as Krebs–Ringer bicarbonate (KRB), it was discovered by the accidental addition of tap water to culture medium being developed for another study by Ringer’s assistant Carl Ludwig. They discovered that the key component in tap water which was critical to maintaining a regular heartbeat in vitro was CaCl2. In 1907, Locke and Rosenheim made further modifications to Ringer’s saline by the addition of 11.1 mM glucose to study the perfused rabbit heart (Locke and Rosenheim 1907). Further modifications were made by the addition of sodium dihydrogen phosphate (NaH2PO4), magnesium chloride (MgCl2), increasing the concentration of NaHCO3 to provide extra buffering capacity (Tyrode 1910), and increasing the concentration of NsHCO3, coupled with the removal of glucose from the formulation (Krebs and Henseleit 1932), to form the basis of Krebs–Ringer bicarbonate solution which, when gassed with 5% CO2 in air or nitrogen is still used to regulate pH in many culture media. The first recorded culture of mammalian embryos was by Brachet in 1912 in the rabbit, followed by studies on the culture of rabbit embryos using a variety of poorly defined culture media, such as coagulated blood plasma (Lewis and Gregory 1929). In 1941, the first successful culture of mouse embryos on a blood clot was reported (Khul 1941). However, In 1949 John Hammond, considered the father of embryo culture, made a significant breakthrough when he reported the culture of mouse embryos from the four‐cell stage through to the blastocyst stage in a medium based on Krebs–Ringer solution but supplemented with glucose and 5% egg white (Hammond 1949). In this seminal work Hammond demonstrated that glucose was essential for compaction and development to the blastocyst stage, a discovery that sparked significant research to model mammalian preimplantation development in vitro. In 1956, an elegant study by Wesley Whitten showed that 90% of eight‐cell mouse embryos could develop to the blastocyst stage in media based on Krebs–Ringer solution supplemented with 5.55 mM glucose, penicillin, and streptomycin and replacing egg white as a protein source with bovine serum albumin (BSA) (Whitten 1956). The development of Whitten’s media was followed by a major breakthrough in 1958 when McLaren and Biggers reported the first successful live births following the transfer of mouse blastocysts derived by culture from the eight‐cell stage in Whitten’s medium (McLaren and Biggers 1958). At the time this development opened ‘Pandora’s box’ in terms of primary scientific research and clinical medicine and was one the key events which lead to human in vitro fertilization (IVF) becoming a reality with the birth of Louise Brown in 1978 (Steptoe and Edwards 1978). Significant developments in embryo culture took place at this time including the addition of lactate to facilitate culture of mouse embryos from the two‐cell stage to the blastocyst stage (Whitten 1957). However, this feat could not be reproduced with pronuclear stage embryos, which led to the identification of the ‘two‐cell block’ in preimplantation mouse embryos. With the exception of some inbred and F1 strains (Brinster 1963; Brinster 1968; Whittingham, 1971), one‐cell mouse embryos exhibit a ‘two‐cell block’ to development in vitro, whereby they undergo a single cleavage division and arrest at the two‐cell stage. Further refinements to Whitten’s media were used to improve the effectiveness of culture from the two‐ and eight‐cell stages to the blastocyst stage which included the addition of phosphenolpyruvate, pyruvate, lactate, and oxaloacetate (Brinster 1963; Brinster 1968; Whittingham 1971). The development of both Brinster’s medium and Whitten’s M16 medium were significant developments but neither could overcome the two‐cell block in the mouse, a phenomenon replicated in other mammalian species at differing cell stages, and which is known to coincide with the activation of the embryonic genome in each species studied (Rieger et al. 1992). The first reported evidence of the two‐cell block being successfully overcome in outbred strains was reported in 1989 when Chatot et al. showed that a high lactate to pyruvate ratio with the addition of 0.1 mM ethylenediaminetetraacetic acid (EDTA) to Brinster’s medium, alongside the removal of glucose, could support development from zygote to morula stage. However, culture to the blastocyst stage could only be achieved through the addition of glucose at the three‐ to four‐cell stage (Chatot et al. 1989) (see Table 26.1 for a summary). Table 26.1 Back to nature: the refinement of embryo culture medium. IVF, in vitro fertilization. Throughout the 1970s and 1980s a major focus of research on the culture of mammalian embryos was centred on refining the composition of media to mimic more closely the environment found in the female reproductive tract. This so called ‘back to nature’ concept is based on the principle that the differences in the composition of the oviductal and uterine fluids that exist throughout the reproductive cycle may reflect the varying metabolic needs of the embryo during early development (Leese 1998). David Gardner developed sequential media for human embryos using the principles, in part, of the back to nature concept, specifically for pyruvate lactate and glucose in the human oviduct and uterus (Gardner et al. 1996; Gardner and Leese 1990). The remaining part of the concept relied on mouse experiments, which may not be directly comparable to the environment in which the human embryo exists in vivo. Controversially, it was suggested that to truly optimize embryo culture, sequential media were required which would meet the changing environments and requirements of the early embryo (Gardner and Leese 1990). The hypothesis was that five distinct media would be required for true sequential culture (Leese, 1995; Biggers and Summers 2008): One of the fundamental issues with this approach to culture media was that the composition of the oviduct and uterine fluids is highly variable and prone to sampling errors in both human and mouse studies (Summers and Biggers 2003). Furthermore, measurement of the composition of fluids from the female reproductive tract are not a true reflection of the microenvironments to which the embryo is exposed and represent only the overall composition. It is also of note that the environment to which embryos are exposed in vitro differs from that in vivo. Embryos cultured in vitro are exposed to a relatively large volume of media in which nutrients are depleted over time and lactate and ammonia concentrations increase. However, in vivo embryos exist in a dynamic environment where contact with maternal tissue and reduced levels of fluid lead to a rapid exchange of nutrients, gases, and waste products. These differences will undoubtedly give rise to different stresses on the developing embryo in the laboratory than those found in vivo. A major advance in the design of embryo culture media was the use of an industrial modelling system known as simplex optimization to determine the optimal concentration of each individual component of the culture media (Lawitts and Biggers 1991a,b). In a series of complex and lengthy experiments they generated a base culture medium based on M16 and CZB medium containing defined concentrations of NaCl, KCl, KH2PO4, MgSO4, lactate, pyruvate, and glucose using BSA as a protein source. Using this base medium a series of media were developed, each with an elevated concentration of a single component. These media were tested for their ability to sustain the development of mouse embryos from the zygote to the blastocyst stage and a computer model was used to optimize each component. This approach required several thousand mouse embryos per optimization, making it impossible as a means for optimizing a medium for human IVF. Nevertheless, through this work the authors discovered that high levels of NaCl, pyruvate, KH2PO4, and glucose were detrimental to mouse embryo development while low levels of NaCl and corresponding low osmolarity were able to overcome the two‐cell block in the mouse. Building on these findings, it was subsequently shown that by increasing the concentration of KCl to 2.5 mM improved blastocyst formation rates (Erbach et al. 1994), which reflected the K+ content of the oviductal fluid. This media, known as KSOM, became the cornerstone of many developments and modifications in culture media over the coming years and through various modifications was shown to support cattle, rabbit, rhesus monkey, porcine, rat, and critically, human embryos (Biggers 1998). However, it should be appreciated that the original optimization was performed in mouse embryos. On reviewing the historical development of embryo culture media it becomes apparent that the human embryo has a capacity to adapt to a variety of culture environments due to a high degree of ‘plasticity’ in the early embryo, as first suggested by Anne McLaren in 1972. However, adaptation by an embryo to suboptimal conditions is not without cost in terms of reduced viability. The more an embryo has to adapt to its environment the more its viability is compromised. Strict control of the components that make up all embryo culture media is therefore imperative. A discussion of these components follows, although it is important to note that this is not exhaustive as IVF culture media contain a huge variety of components, not all of which are fully declared in the published formulations. All commercially available embryo culture media share the same inorganic ions (Na+, K+,Cl−,Ca2+, Mg2+, SO42−, and in some cases PO42−) (Biggers 1998). The use of these salts in media formulation can be traced back to the original KRB. Patrick Quinn devised the first commercial medium specifically for human embryo culture, known as ‘human tubal fluid’ (HTF) in 1985. The name, however, was a misnomer, since the concentrations of salts used were not taken from fluid collected from human Fallopian tubes, but were based on those for mouse embryo culture such as M16 and Whitten’s media, with the exception of Mg2+ and PO42− which were at much lower concentrations in Quinn’s HTF (Quinn et al. 1985a,b). These days a wide array of both single‐step and sequential culture media are available. However, historically a direct comparison was difficult as most commercial media are proprietary formulations. Recently, through the use of high purity liquid chromatography and mass spectrometry, two studies have analysed the formulations of several commercially available sequential and single‐step culture systems (Morbeck et al. 2014; Morbeck et al. 2017). These studies confirm that several media do contain salt concentrations similar to those observed in the original SOM and KSOM formulations (Lawitts and Biggers 1991a). Inorganic ions serve a variety of roles in cell function. One such function is in maintaining an intracellular environment in which K+ concentrations are high and Na+ concentrations are low (Kaplan 2002). This distribution is largely mediated by the Na+,K+‐ATPase (sodium pump) enzyme and is crucial to maintain the cell membrane potential, which indirectly provides an energy source for numerous ‘electrogenic’ transporters critical for the transport of metabolites and amino acids as well as the regulation of intracellular pH (Baltz and Zhou 2012). The concentration of inorganic ions is fundamental to the regulation of the external and internal osmolality of the early embryo. Osmolality is a measure of the total osmotic pressure in a solution (osmol/kg). Osmolarity is closely connected with the regulation of cell volume as mammalian cells control cellular volume by adjusting intracellular concentrations of osmotically active solutes to regulate osmotic pressure (Hallows and Knauf 1994; Hallows et al. 1994). The influx of osmolytes raises intracellular pressure, which in turn causes an increase in cell volume, whereas an efflux of osmolytes leads to a reduction in cell volume. The early embryo is extremely sensitive to increases in external osmolarity as evidenced during the development of KSOM media that had a significantly lower osmolarity (250 mOsM) than that of M16 and CZB media (290 mOsM and 275 mOsM respectively). The addition of NaCl to KSOM to raise the osmolarity to levels similar to that seen in M16 and CZB was sufficient to initiate the two‐cell block in mouse embryo development (Hadi et al. 2005; Wang et al. 2005). Why do preimplantation embryos show improved development in low osmolarity? One possibility is that such conditions mimic the low osmolarity present in the female tract. However, this is unlikely as analysis of mouse, rat, and porcine oviductal fluid showed a range of osmolarities from 290 to 310 mOsM (Waring, 1976; Collins and Baltz 1999; Li et al. 2007). It was also shown in a series of experiments at higher osmolarity, blocks to embryo development could be avoided through the addition of any of the following organic osmolytes: amino acids or amino acid derivatives such as glutamine, glycine, proline, betanine, and β‐alanine (Van Winkle et al., 1990; Lawitts and Biggers 1991b; Hammer and Baltz 2003). Indeed, glycine has been shown to be the principle organic osmolyte used by the preimplantation embryo as the high affinity glycine transporter GLYT1 is expressed and active in mouse embryos until the four‐cell stage and regulates the accumulation of glycine in the early embryo (Steeves et al. 2003). Furthermore, GLYT1 is also expressed in human embryos indicating a similar role (Hammer et al., 2000). GLYT1, however, cannot regulate the uptake of betaine and proline which are regulated by SIT1 which is also active in the early embryo and appears to work in tandem with GLYT1 as betaine is accumulated at lower levels than glycine in the early embryo (Anas et al. 2008). These findings were consistent with the hypothesis that the preimplantation embryo maintains cellular volume via mechanisms similar to those observed in somatic cells. However, unlike somatic cells the levels of inorganic ions required to maintain normal cell volume can exert a negative effect on development such that the early embryo can replace a portion of these with organic osmolytes (Van Winkle et al. 1990). During the first 6 days of development in vivo, the human preimplantation embryo most likely exists in a constantly changing microenvironment, containing a variety of nutrients as it grows from fertilization through to the blastocyst stage. However, this cannot be confirmed since the microenvironment cannot be sampled and, thinking logically, the environment in the oviduct should, one imagines, be focused in maintaining homeostasis and minimizing variation. The key stages in the early development of the embryo include rapid early mitotic cleavage divisions to the eight‐cell stage in the absence of cellular growth as a consequence of a reduction in cellular biomass through reducing blastomere size with each division. This is followed by compaction of the early embryo and the formation of gap and tight junctions between the cells of the early embryo to facilitate cell to cell communication and nutrient exchange during the process of blastocyst formation during which the first major event of cellular differentiation occurs giving rise to two distinct cell populations; the trophectoderm and the inner cell mass. During this stage, major increases in protein synthesis and increased metabolic activity are evident. With regard to energy sources and metabolites, we can ask: (i) how does the preimplantation embryo regulate these processes?; (ii) what are the metabolic requirements of the embryo during this time?; (iii) what nutrients are required in a culture medium? In the early cleavage stages of development, genetic control of the embryo relies mainly on maternal mRNA stores prior to activation of the embryonic genome between the four‐ and eight‐cell stage in the human embryo. During early cleavage, metabolic activity is low compared with that required for blastocyst formation activation when metabolic activity increases significantly (Leese 1991; Leese 1995). The major nutrients available to the early embryo in vivo are: (i) pyruvate; (ii) lactate; (iii) glucose; (iv) amino acids; and (v) endogenous triglyceride. The role of amino acids as nutrients in embryo culture media are mainly discussed later. In the female reproductive tract, namely the oviduct and uterus, the embryo is exposed to differing concentrations of pyruvate, lactate, and glucose (Table 26.2). Pyruvate and lactate concentrations are relatively high in the early cleavage stages of development in the human oviduct before falling significantly during compaction and blastocyst formation in the uterus (Gardner et al. 1996). The importance of pyruvate as an energy source for the cleavage stage embryos was first established in the 1960s and 1970s (Biggers and Stern 1973). The early embryo generates adenosine triphosphate (ATP) through oxidative phosphorylation of pyruvate and lactate and amino acids such as aspartate and glutamine (Leese and Barton 1985; Gardner et al. 1996; Lane and Gardner 2005). However, excess concentrations of lactate can alter the rate of pyruvate oxidation in the cell, which in turn affects the availability of NAD+ and NADH, and of ATP production. For this reason, both pyruvate and lactate are key components of all commercial culture media with the balance of these nutrients being critical due to their ability to sustain the metabolism and viability of the developing embryo. Table 26.2 The differing metabolic requirements of the embryo as it develops in the female tract based on the analysis of the oviductal and uterine fluids. As the embryo develops low glucose combined with high pyruvate and lactate is required during the cleavage stages in the oviduct, switching to high glucose and low pyruvate and lactate as the embryo undergoes compaction and blastulation prior to implantation in the uterus. In common with the situation observed in vivo some commercial sequential IVF media such as the G series™ (Vitrolife, Göteborg, Sweden) have pyruvate and lactate concentrations which are higher in the cleavage stage medium compared with levels in the blastocyst stage medium, with the ratio of pyruvate to lactate being optimized to avoid negative effects from the impact of lactate on the regulation of redox potential. This is not always the case, as in other systems such SAGE Quinn’s Advantage™ (Origio, Målov, Denmark) and Sydney IVF™ (Cook Medical, Bloomington, IN, USA) the difference in lactate between the stage one and stage 2 media is less pronounced (Morbeck et al. 2014). To date several studies have assessed the uptake of pyruvate in relation to pregnancy and blastocyst formation rates. Embryos that develop to the blastocyst stage show increased pyruvate uptake between Days 2.5 and 4.5, whereas those arrested at cleavage stages had significantly lower pyruvate uptake. Interestingly, polyspermic and parthenogenetic embryos also had lower pyruvate uptakes at later stages indicating the impaired developmental potential of these embryos (Hardy et al. 1989b). These findings were further supported in a study where the levels of pyruvate and glucose uptake, and lactate production in embryos which arrested were below those which developed normally (Gott et al. 1990). Furthermore, culture of embryos in pyruvate‐free medium resulted in developmental arrest in the majority of cleavage‐stage embryos and those that did reach the blastocyst stage showed significantly reduced metabolic activity during the morula to blastocyst stage transition (Conaghan et al. 1993a). These studies were limited to the assessment of the levels of uptake of pyruvate with a view to its potential as a biomarker of developmental potential. It is important to consider the metabolic fate of pyruvate, as high uptake may indicate inappropriate or inefficient use of an energy substrate in the developing embryo (Zander‐Fox and Lane 2012). A recent retrospective study has proposed that there may actually be a threshold at which overconsumption of pyruvate exerts a negative effect. In this study, the authors proposed the existence of a ‘’Goldilocks zone’, or as it is known in Sweden, of lagom meaning ‘just the right amount’ within which embryos with maximum developmental potential can be classified (Leese et al. 2016).
In Vitro Culture of Gametes and Embryos – The Culture Medium
Introduction
Historical Development of Embryo Culture Media
Year
Discovery
Author
1561
First correct anatomical description of the Fallopian tube
G. Fallopius
1677
Discovery of mammalian spermatozoa
A. Van Leeuwenhoek
1797
Recovery of embryos from rabbit Fallopian tube
W.C. Cruikshank
1827
Identification of an egg in a mammalian ovarian follicle
K.E. Von Baer
1890
Understanding that fertilization requires the fusion of one sperm with one egg in sea urchin, rabbits and starfish
O. Hertwik (sea urchin)
E. Van Beneden (rabbit)
H. ol (starfish)
1890
First documented embryo transfer in rabbits
W. Heape
1912
First documented culture of mammalian embryos (rabbits)
A. Brachet
1930
First published experiments on IVF carried out in rabbits
G. Pincus
1932
‘Brave New World’ was published by Aldous Huxley. Realistically describing the technique of IVF as we know it
A. Huxley
1944
First attempted at IVF using human oocytes
J. Rock and M. Menkin
1949
Retrieval and successful culture of mouse oocytes
J. Hammond Jr
1949
Successful development of culture medium to support development of mouse embryos from eight‐cell to blastocyst stage
J. Hammond Jr
1951
Capacitation in spermatozoa
M.C. Chang; C.R. Austin
1958
Successful embryo transfer of mouse blastocysts to a recipient female followed by birth of live young
A. McLaren and J.D. Biggers
1959
Unequivocal demonstration of IVF in the rabbit
M.C. Chang
1963
Development of culture media to support culture of mouse embryos from two‐cell to blastocyst stage
R.L. Brinster
1969
Successful fertilization of the human oocyte in vitro
R.G. Edwards, B.D. Bavister and P.C. Steptoe
1972
Successful cryopreservation of mouse embryos
I. Wilmut, D.G. Whittingham
1976
Development of the first specific culture medium to reflect the follicular, tubal, and uterine environments of the sheep, rabbits, and humans
Y. Menezo
1976
First human IVF pregnancy (ectopic)
P.C. Steptoe and R.G. Edwards
1978
Birth of the world’s first child from IVF (Louise Brown)
P.C. Steptoe and R.G. Edwards
Back to Nature: The Refinement of Embryo Culture Medium
Salts and Osmolality
Energy Sources and Metabolites
Site
Pyruvate (mM)
Lactate (mM)
Glucose (mM)
Oviduct
0.32
10.5
0.50
Uterus
0.10
5.87
3.15
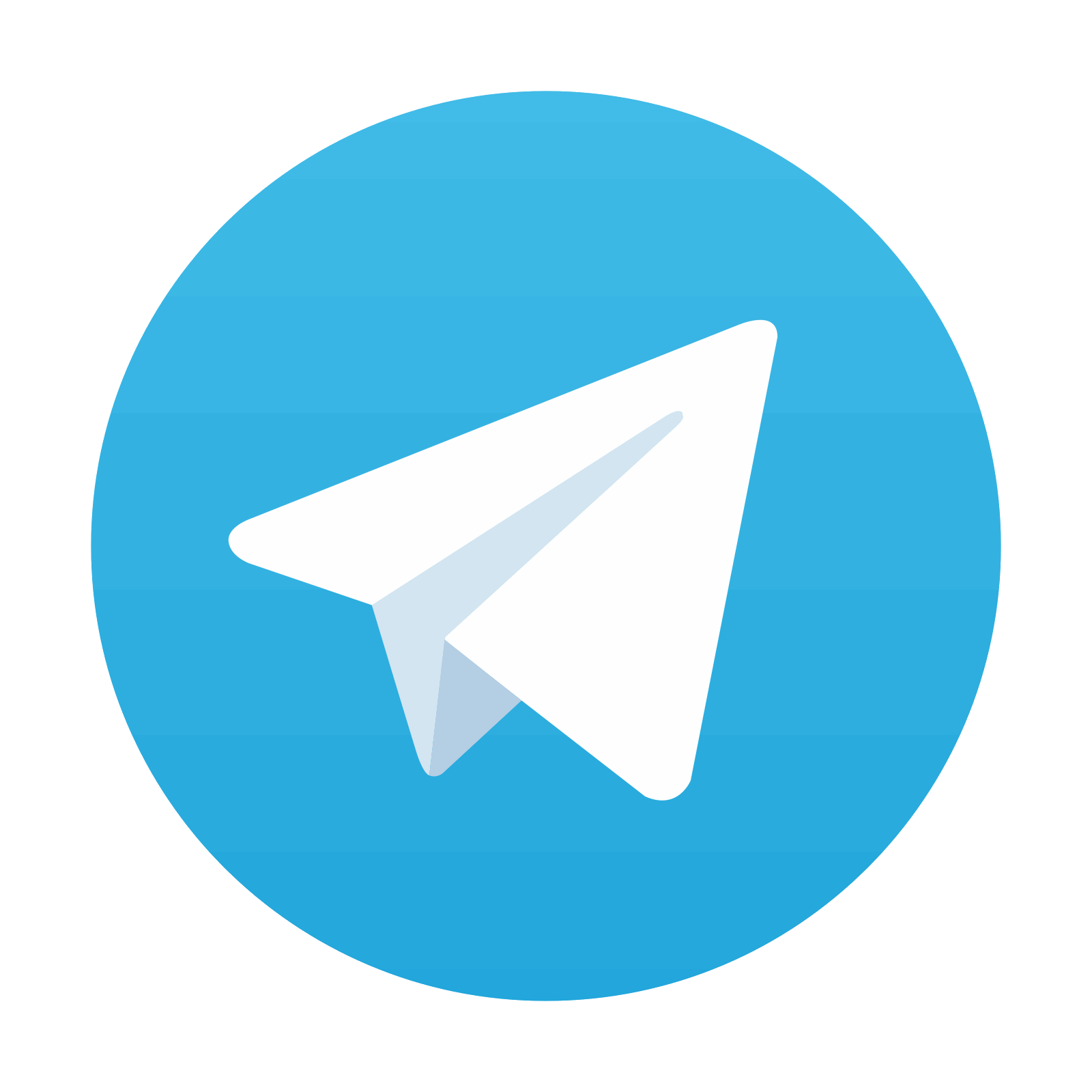
Stay updated, free articles. Join our Telegram channel

Full access? Get Clinical Tree
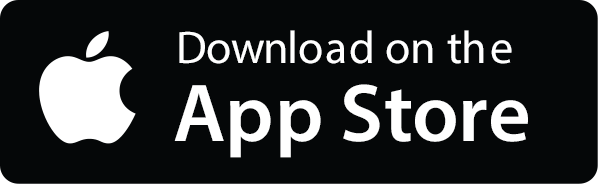
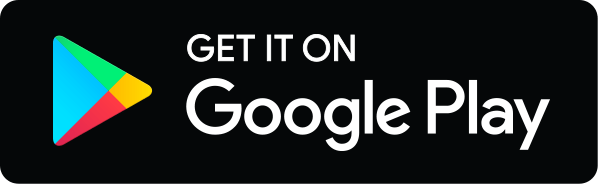