Immunosuppression in Organ Transplantation
Pierre-Alain Clavien
Philipp Dutkowski
Historical Evolution
Since the classical studies of Medawar et al. in the early 1950s, it became clear that transplanted grafts between individuals from the same species are liable to be destroyed by an immune reaction, which involves both cellular and humoral elements. In the subsequent 50 years, solid organ transplantation has evolved as an extraordinarily successful form of treatment for patients who previously would have died.
In the early history of transplantation, there were two approaches to prevent rejection of allogeneic tissue. One was acquired immunologic tolerance discovered naturally in dizygotic twins, and then, demonstrated experimentally between inbred strains of mice by Medawar’s group. The other approach was to destroy the immune system of the recipient completely by total body irradiation and then rescue the patient by transplanting bone marrow from a putative donor.
Although these two ideas did not seem to have a direct bearing on organ grafting, much has been learned of the basic immunology of tolerance and from the development of bone marrow transplantation. In the clinic, every successful bone marrow transplant is an example of “immunologic tolerance” since the patient accepts the graft without the need for any continuing immunosuppressive treatment, and if a kidney or a liver from the same donor is transplanted to the recipient of the marrow, no further immunosuppression is needed and the graft is accepted. The mechanisms of graft rejection have been studied intensively in many laboratories. The characteristics of each individual, as defined by red blood cell groups and histocompatibility or human leukocyte antigens (HLA), provide a unique mosaic for each human being. Identity of all factors can only be expected between identical twins, but matching for the major red blood cell groups and avoiding mismatches of the six major HLA antigens provide a setting in which relatively mild immunosuppression is likely to get very good long-term results. The minor incompatibilities can be controlled with low-dose standard immunosuppression.
There is no doubt, however, that immunologic engagement between donor and recipient occurs in all organ allografts and this inevitably lead to graft rejection. The allograft becomes infiltrated with T-lymphocytes, plasma cells, and macrophages; alloantibodies may develop; and small blood vessels tend to bear the brunt of rejection damage in the acute phase and also in patients who undergo so-called chronic rejection, in which there is damage to the endothelium of the graft vessels with reduplication of the intima leading to thickening and eventual obstruction of graft arterioles and small arteries. The story of immunosuppression can be summarized in terms of major agents that have been shown to prevent graft rejection, or at least attenuate the process.
The early attempts at clinical kidney transplantation utilized total body irradiation, but this tool was too nonspecific and ineffective to be of value, except in the closely related sibling–sibling transplants. There were two famous cases, one in Boston and the other in Paris, of transplants between nonidentical twins, who were in fact, disclosed identical matches for the six major HLA antigens. The success of these cases led to disastrous trials using similar immunosuppressive protocol in recipients, who received an organ from a donor not so closely related. These failures demonstrated the need for a different approach. In 1959, Schwartz and Dameshek reported that the antileukemia drug 6-mercaptopurine impaired the ability of rabbits to respond immunologically to foreign antigens. Following this report, 6-mercaptopurine was investigated in dogs with renal allografts and was shown to prolong survival. Afterwards, the imidazole derivative of 6-mercaptopurine, azathioprine, was shown to be somewhat superior to 6-mercaptopurine in a dog model of allograft renal transplantation, and this drug was used in the first clinical trials of immunosuppression in renal transplantation. Initial results were again disappointing and, could be improved only when anti-inflammatory corticosteroids were combined with azathioprine. These two drugs then constituted the initial phase of clinical immunosuppression for kidney grafting with the expectation of reasonable results (e.g., graft survival and function at 1 year around 50%), compared with any other previous strategies including the disastrous experience with total body irradiation. In fact, some of the earliest patients treated with this regimen are still alive.
The tentative development of kidney transplantation was carried out in a few centers in the United States and Europe, but it took more than 20 years to see the implementation of such programs in many centers. The routine application of solid organ transplantation had to wait for the landmark discovery by J.F. Borel and H. Stähelin, working in the Sandoz laboratories, who described the immunosuppressive effects, both in vitro and in vivo of the cyclic peptide cyclosporine (CsA). Use of this agent in animals with organ grafts, first with heart grafts in rats, and then in dogs with kidney grafts, and subsequently in pigs with orthotopic heart grafts, showed that CsA was far more powerful than any other products that had been investigated; in addition, the early use of cyclosporine with and without steroids resulted in a better outcome, and life-supporting graft survival of 80% per year was reported. The unexpected observation of nephrotoxicity in patients treated with cyclosporine was a serious setback, so that although early graft survival was much improved with the use of cyclosporine, by 10 years results were little different from those in patients treated with azathioprine and steroids. It was suspected that the nephrotoxicity of CsA was mainly responsible for the late failures. A strategy was developed for combining the three drugs—cyclosporine, azathioprine, and corticosteroids—in lower dose each.
In the mid-1990s, mycophenolate mofetil (MMF) replaced azathioprine in many centers in view of trials demonstrating its superiority. The rationale of this strategy combining several drugs was to benefit from the additional immunosuppressive effects of each of the drugs, while avoiding the serious side effects associated with high doses of each of the agents. Protocols based on this theme were used worldwide and the number of centers engaged in organ transplantation increased from a handful to more than a thousand in a few years. Confidence developed in clinical transplantation of the liver and the heart, and to a lesser extent the lung and the pancreas. In some centers polyclonal antilymphocyte antibodies were added to the protocol or used for treatment of acute rejection. The principle extensively investigated by Woodruff et al. was to inject human lymphocytes into animals and use the animal serum as an
immunosuppressive agent. Unfortunately, batches tended to vary. Some were effective without toxicity, while others were ineffective and/or toxic.
immunosuppressive agent. Unfortunately, batches tended to vary. Some were effective without toxicity, while others were ineffective and/or toxic.
Kohler and Milstein’s discovery of how to make monoclonal antibodies has been a major step forward. The advantage of monoclonal antibodies is that each one is directed against a single molecular target, and it has been possible to modify the antibodies to remove most of the animal origin components, so that such “humanized” or chimeric antibodies are unlikely to elicit xenoantibody responses.
Nowadays, clinicians are more experienced in recognizing early acute rejections at the stage when corticosteroid bolus treatment for a few days is usually successful in reversing the process. One attack of acute rejection managed efficaciously does not seem to have a serious long-term deleterious effect on the graft, but repeated acute rejections, particularly when they do not fully respond to corticosteroids or antibodies, are a harbinger for chronic rejection and graft failure later.
Over the years, many new agents have been investigated; some have reached the clinic and unfortunately there has been a tendency for clinicians to add new agents to the standard protocol, often with the result of overimmunosuppression and certainly interfering with any natural biologic switch-off mechanisms that are part of every immune response.
Rejection Mechanisms and Immunosuppressive Agents
The majority of the commonly used immunosuppressive drugs control rejection mechanisms by targeting the immune response in a nonspecific manner. Despite this shortcoming, acute allograft rejection can currently be prevented or treated with modern immunosuppressive agents, leading to an 85% to 90% 1-year survival for most organs. Besides, there remains a certain loss of transplanted grafts with an annual rate of 3% to 5% due to chronic allograft rejection through a still incompletely understood process. In addition, most of the currently used drugs lead to significant side effects, including nephrotoxicity, increased infection rates, and a risk of tumor development, as well as increased incidence of cardiovascular diseases. Minimizing these side effects is one dominant task for future research. For example, within the era of liver allocation through the model for end-stage liver disease (MELD) system, most candidates for liver transplantation have renal dysfunction already at the time of transplant; further, exacerbation by the use of calcineurin inhibitors appears at present as one of the major problems, postoperatively.
Another goal is the induction of a sustained specific tolerance to donor alloantigens in the absence of any immunosuppressive therapy. However, the pathways of allograft recognition leading to rejection are extremely complex and far away of complete discovery. Current knowledge is shortly summarized as follows.
Immune reactions are systematically divided into: (a) alloantigen recognition, (b) lymphocyte activation, (c) clonal expansion, and (d) graft inflammation. Progression from alloantigen recognition to cellular activation depends on the presence of several stimulatory signals presented mostly to T-cell lymphocytes. Immune responses can either be induced by recognition of allogenic molecules of the major histocompatability complex (MHC) at the surface of donor cells (direct pathway) or by peptides derived from MHC molecules presented on recipient cells (indirect pathway). Direct alloresponse is currently believed to occur more in the early posttransplant period, while indirect pathways may contribute to chronic rejection. In the early stages after transplantation, tissue-resident immature dendritic cells migrate out of the graft toward lymphoid organs where they mature and involve memory T-cells. It is conceivable, on the other hand, that graft dendritic cells may also present antigens without migration. However, the trafficking and maturation of dendritic cells is triggered by inflammatory signals produced as a result of tissue injury during organ procurement or transplant surgery, and appears as cornerstone for the initiation of effective adaptive immune response. Whether the first meeting point between host T-cells and foreign transplant antigens occurs in the secondary lymphoid organs or in the graft itself remains controversial.
Full T-cell activation requires two distinct signals. The first signal (signal 1) is delivered through the T-cell receptor by recognition of peptide antigens presented in the context of allograft MHC molecules on the antigen-presenting cells. Additional costimulatory signals (signal 2) are delivered via inducible receptors on the T-cell surface. These activating signals are balanced by inhibitory signals allowing a downregulation of the response after initial T-cell activation.
Activated T-cells mediate through intracellular calcium and several promoters and increased transcription of interleukin-2 (IL-2) gene. Once IL-2 expression started, T-cells undergo a burst of proliferative activity, dependent on IL-2 receptor activation. When their population is expanded, these T-cells exhibit the same regulatory and cytotoxic functions as would be predicted by the immune response to infection. The damage occurs through a number of different routes, including the release of destructive enzymes, vasoactive and toxic proteins, and direct cell-mediated cytotoxicity. The resulting injury is clinically apparent as acute rejection; for example, manifested histologically in the liver as endotheliitis and bile duct damage.
Based on these pathways, modern immunosuppressive agents can be separated into four effector classes (Fig. 1):
Class 1: Depletion of T-cells by polyclonal or monoclonal antibodies against lymphocytes (e.g., anti-thymocyte globulin, OKT3)
Class 2: Inhibition of T-cell activation by blockage of the T-cell receptor-dependent activation pathway (e.g., calcineurin inhibitors: cyclosporine, tacrolimus)
Class 3: Inhibition of the IL-2 receptor by specific antibodies or biochemical interference (e.g., basiliximab, daclizumab)
Class 4: Inhibition of T-cell proliferation downstream the IL-2 receptor, such as cell cycle blockade (e.g., azathioprine, MMF or enteric-coated mycophenolic acid, and mammalian target of rapamycin (mTOR) inhibitors (sirolimus and everolimus).
Over the last 10 years, many additional agents were identified as promising candidates for clinical investigation, but several of those failed the rigors of clinical development (e.g., FTY720, FK778). Consequently, there has been no new approval for transplant therapeutics within this period in contrast to the 1990s. The reasons for this are: (1) a continuous reduction of acute rejection after liver, lung, and kidney transplantation and (2) toxicities associated with current regimens. Besides the search for new immune targets, the dominant goal of the transplant drug development is therefore the reduction of long-term side effects associated with current regimes.
Yet, seven drugs are in phase II/III clinical trials, including ISA247, AEB071, CP690550, belatacept, efalizumab, and alefacept. They can also be attributed to the above-mentioned classes (Fig. 1):
Isa247
ISA247 is a semisynthetic structural analogue of cyclosporine with modification at
the first amino acid of the molecule (Class 2). Studies in rats, rabbits, dogs, and monkeys suggest that ISA3247 has no nephrotoxicity, despite its even higher immunosuppressive effect compared to cyclosporine. A phase II trial in renal transplant recipients showed promising results in terms of low incidence of rejection and preserved renal function. A phase III trial is therefore awaited.
the first amino acid of the molecule (Class 2). Studies in rats, rabbits, dogs, and monkeys suggest that ISA3247 has no nephrotoxicity, despite its even higher immunosuppressive effect compared to cyclosporine. A phase II trial in renal transplant recipients showed promising results in terms of low incidence of rejection and preserved renal function. A phase III trial is therefore awaited.
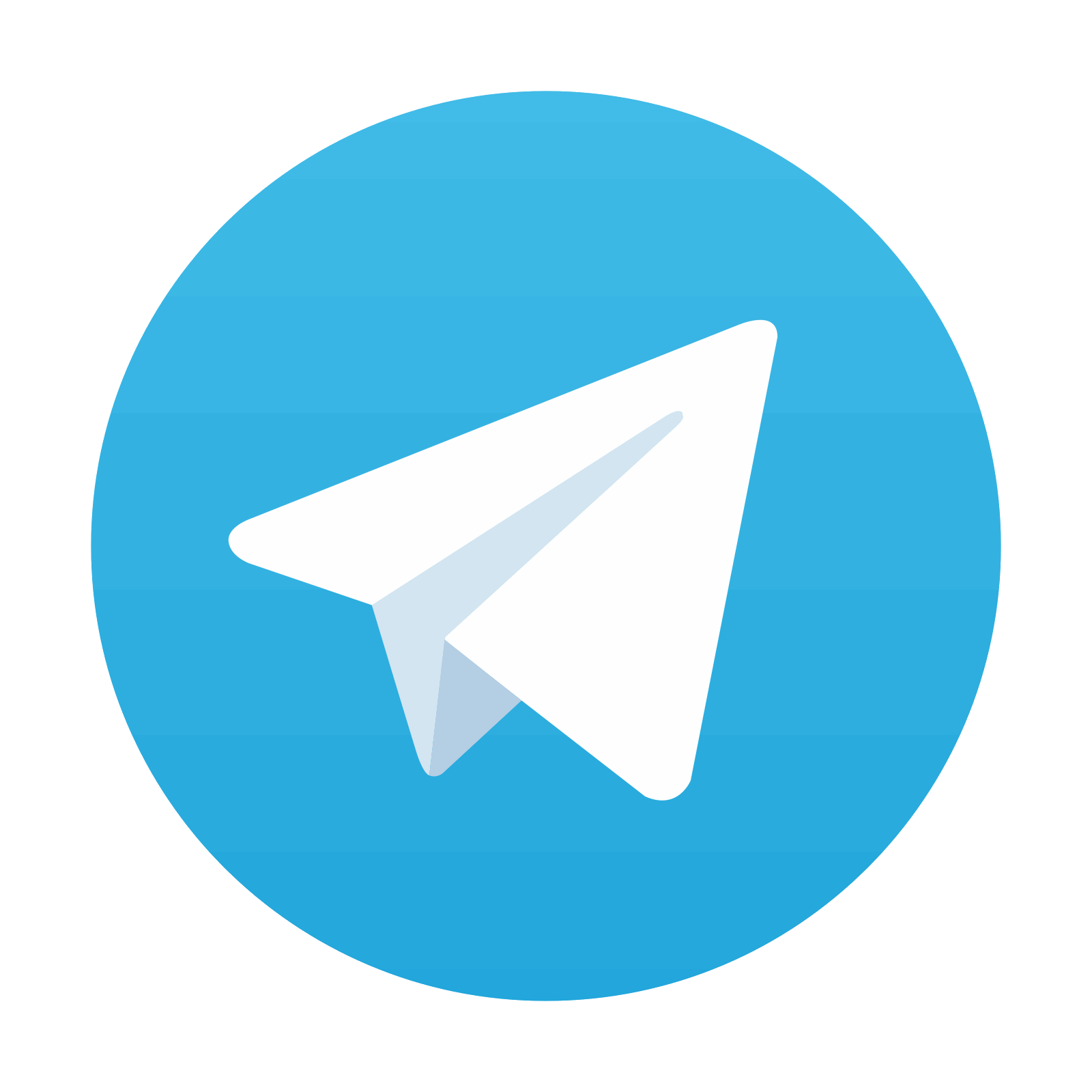
Stay updated, free articles. Join our Telegram channel

Full access? Get Clinical Tree
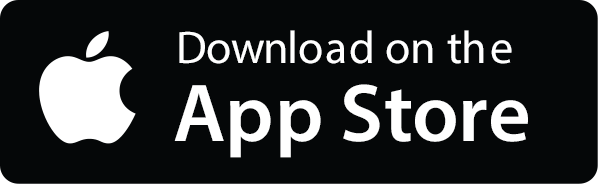
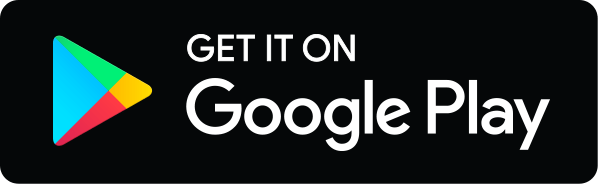