18 Immunohistochemical techniques
Introduction
Many antibodies are now available to identify epitopes that survive the rigors of formalin fixation and processing to paraffin wax. In cases where morphology and clinical data alone do not allow a firm diagnosis, then immunohistochemistry is invaluable. The increasing use of prognostic and predictive markers permits the pathologist to make decisions that could profoundly affect patient management (Fig. 18.1).
In 1941 Albert H. Coons described a revolutionary new way of visualizing tissue constituents using an antibody labeled with a fluorescent dye. Visualization of the labeled complex was achieved by the use of a fluorescence microscope. The first fluorescent dye to be attached to an antibody was fluorescein isocyanate, but fluorescein isothiocyanate soon became the label of choice because the molecule was much easier to conjugate to the antibody and the result was more stable (Riggs et al. 1958). Fluorescein compounds emit a bright green fluorescence when excited at a wavelength of 490 nm. The technique has enormously expanded and developed following the early work. New labels have been introduced, including red, yellow, and blue fluorochromes. This permits the simultaneous visualization of several separately labeled antibodies on a single preparation. Currently, fluorescein isothiocyanate and rhodamine are among the most popular fluorochromes. This methodology, whilst useful in some diagnostic areas, such as determining the nature of protein deposits in skin and renal diseases, and bacteria in infected material, has certain limitations. A fluorescence microscope is necessary to visualize the fluorochrome, and this has a tendency to fade. More importantly and probably the greatest disadvantage of immunofluorescence is that it is difficult to demonstrate the morphological detail of the labeled cell and the associated tissue components. The success of immunohistochemistry in some areas of pathology stimulated interest in the development of alternative antibody labeling techniques that would avoid the difficulties and limitations associated with immunofluorescence.
Many limitations were overcome with the introduction of enzymes as labels. Cells that have been labeled with an enzyme such as horseradish peroxidase, conjugated to an antibody, and visualized with an appropriate chromogen such as diaminobenzidine (DAB) (Nakane & Pierce 1966), can be counterstained with traditional nuclear stains such as hematoxylin. This permits the simultaneous evaluation of both specific immunohistochemistry and morphological detail. In 1970 Sternberger et al. described the peroxidase-anti-peroxidase (PAP) technique. In 1971, Engvall and Perlman reported the use of alkaline phosphatase labeling, and Cordell et al. (1984) described the alkaline phosphatase-anti-alkaline phosphatase (APAAP) technique. Heggeness and Ash (1977) proposed the use of avidin-biotin for immunofluorescence. This technique was modified by Guesden et al. (1979) and Hsu et al. (1981) who used a horseradish peroxidase label. Avidin-biotin labeling was superseded by streptavidin-biotin labeling, and was one of the more popular techniques used in diagnostic laboratories. However, labeled polymer detection systems are now the most popular choice for most diagnostic laboratories.
Certain epitopes, for example the proliferation antigen, Ki67 and T-cell antigens CD2, CD4, CD5 (Fig. 18.2), CD7 and CD8, can only be demonstrated in formalin-fixed, paraffin wax-processed tissue after heat pretreatment, and then only with certain monoclonal antibody clones. Antibodies such as those directed against the leukocyte common antigen (clones PD7/2B11) and the CD20 antigen (clone L26) produce enhanced staining after citrate buffer (pH 6.0) heating. Surprisingly, heat pretreatment allows for considerably greater dilution factors. The demonstration of antigens such as cyclin D1 (with clone DCS-6), on the other hand, is better in a high pH solution (Tris-EDTA, pH 10.0). However, the use of the cyclin D1 rabbit monoclonal antibody (clone SP4) produces very good results using citrate buffer, pH 6.0 (Fig. 18.3).
Immunohistochemistry theory
Definitions
Antibody-antigen binding
The amino acid side-chains of the variable domain of an antibody form a cavity which is geometrically and chemically complementary to a single type of antigen epitope as described by Capra and Edmundson in 1977. The analogy of a lock (antibody) and key (antigen) has been used, and the precise fit required explains the high degree of antibody-antigen specificity seen. The associated antibody and antigen are held together by a combination of hydrogen bonds, electrostatic interactions, and van der Waals’ forces.
Production of primary reagents
Polyclonal antibodies
Polyclonal antibodies are produced by immunizing an animal with a purified specific molecule (immunogen) bearing the antigen of interest. The animal will mount a humoral response to the immunogen and the antibodies so produced can be harvested by bleeding the animal to obtain immunoglobulin-rich serum. It is likely that the animal will produce numerous clones of activated plasma cells (polyclonal). Each clone will produce an antibody with a slightly different specificity to the variety of epitopes present on the immunogen. A polyclonal antiserum is therefore a mixture of antibodies to different epitopes on the immunogen. Some of these antibodies may cross-react with other molecules and will need to be removed by absorption with the appropriate antigen. The antiserum will probably contain antibodies to impurities in the immunogen. Antibodies raised against the contaminating immunogens are often of low titer and affinity, and can be diluted out to zero activity for immunolabeling. In consequence of the high possibility of a wide spectrum of naturally occurring antibodies being present in the host animal in response to previous antigen challenges, serum removed from the animal before injection of the immunogen is important as a negative or pre-immune control. For precise details of polyclonal antibody production see De Mey and Moeremans (1986).
Monoclonal antibodies
The development of the hybridoma technique by Kohler and Milstein in 1975 to produce monoclonal antibodies has revolutionized immunohistochemistry by increasing enormously the range, quality, and quantity of specific antisera. Detailed descriptions of the technique have been given by Gatter et al. (1984) and Ritter (1986).
This approach to the production of monoclonals has dramatically increased the number of antibodies available for immunohistochemistry and has allowed for further evolution with the ability to identify more antigens in paraffin sections. Detailed comparisons of the values and limitations of polyclonal and monoclonal antibodies have been given by Warnke et al. (1983) and Gatter et al. (1984).
Lectins
Lectins are plant or animal proteins that can bind to tissue carbohydrates with a high degree of specificity according to the lectin and the carbohydrate group (Brooks et al. 1996). Since the carbohydrates may be characteristic of a particular tissue, lectin binding may have diagnostic significance (Damjanov 1987). They can be labeled in similar ways to antibodies, or identified by using lectin-specific antibodies as secondary reagents (Leatham 1986).
Labels
Enzyme labels
Horseradish peroxidase is the most widely used enzyme, and in combination with the most favored chromogen, i.e. 3,3α-diaminobenzidene tetrahydrochloride (DAB), it yields a crisp, insoluble, stable, dark brown reaction end-product (Graham & Karnovsky 1966). Although DAB has been reported to be a potential carcinogen, the risk is now thought to be low (Weisburger et al. 1978).
Horseradish peroxidase is commonly used as an antibody label for several reasons:
• Its small size does not hinder the binding of antibodies to adjacent sites.
• The enzyme is easily obtainable in a highly purified form and therefore the chance of contamination is minimized.
• It is a stable enzyme and remains unchanged during manufacture, storage, and application.
Other chromogens are available, including: 3-amino-9-ethylcarbazole (Graham et al. 1965; Kaplow 1975), which gives a red final reaction product; 4-chloro-1-naphthol (Nakane 1968), producing a blue final reaction product; Hanker-Yates reagent (Hanker et al. 1977), producing a dark blue product; and α-naphthol pyronin (Taylor & Burns 1974), which produces a red-purple final reaction product.
Endogenous peroxidase activity is present in a number of sites, particularly neutrophil polymorphs and other myeloid cells. Blocking procedures may be required, the hydrogen peroxide-methanol method (Streefkerk 1972) being the most popular. However, care should be taken with certain antigens – notably CD4, where too long an incubation in the blocking solution or too high a concentration of hydrogen peroxide can significantly diminish staining on formalin-fixed paraffin-embedded tissue. Performing the peroxidase block after the binding of the primary antibody to the tissue antigen is to be recommended for antibodies such as CD4.
Calf intestinal alkaline phosphatase is the most widely used alternative enzyme tracer to horseradish peroxidase, particularly since the development of the alkaline phosphatase-anti-alkaline phosphatase (APAAP) method in 1984 by Cordell et al. Fast red TR used with naphthol AS-MX phosphate sodium salt gives a bright red reaction end-product that is soluble in alcohol. New fuchsin has been reported as giving a permanent insoluble red product (Malik & Daymon 1982) when mounted in resinous mountant, but it is the experience of many workers in the field that the resistance of the reaction product to resinous mounting is inconsistent.
Some workers use glucose oxidase as a tracer and it can be developed to give a navy blue reaction (Suffin et al. 1979). This label lends itself to immunohistochemistry on animal tissue, as there is no endogenous enzyme activity in animals.
Bacterial-derived β–D-galactosidase has also been used as a tracer and can be developed using the indigogenic method to give a permanent turquoise-blue reaction end-product (Bondi et al. 1982). Endogenous enzyme activity is not a problem as the endogenous mammalian enzyme has a different optimum pH from the tracer enzyme.
Colloidal metal labels
When used alone, colloidal gold conjugates appear pink when viewed using the light microscope. A silver precipitation reaction can be used to amplify the visibility of the gold conjugates (Holgate et al. 1983a). In addition, both gold and silver-enhanced gold conjugates can be visually emphasized using polarized incident light (epi-illumination) microscopy (Ellis et al. 1988) (Fig. 18.4a, b).
Silver may also be used as a conjugate, and it gives a yellow color that is visible directly (Roth 1982). Other metals such as ferritin may be used, but they have not found wide usage among immunohistochemists using light microscope techniques. Colloidal gold has much wider usage with the electron microscope.
Radiolabels
The use of radioisotopes as tracers requires autoradiographic facilities, and developed from the need for quantitation in immunohistochemistry. Internally labeled antibodies are not widely available and labeling, at present, often limits the activity of the antibody. Techniques involving the use of radioisotopes as tracers have been discussed by Hunt et al. (1986).
Immunohistochemical methods
Traditional direct technique
The primary antibody is conjugated directly to the label. The conjugate may be either a fluorochrome (more commonly) or an enzyme (Fig. 18.5a). The labeled antibody reacts directly with the antigen in the histological or cytological preparation. The technique is quick and easy to use. However, it provides little signal amplification and lacks the sensitivity achieved by other techniques. Its use is mainly confined to the demonstration of immunoglobulin and complement in frozen sections of skin and renal biopsies. Low levels of antigen present in certain tumors may not be demonstrated by this technique and this could be crucial to an accurate and comprehensive diagnosis.
Two-step indirect technique
A labeled secondary antibody directed against the immunoglobulin of the animal species in which the primary antibody has been raised (Fig. 18.5b) visualizes an unlabeled primary antibody. Horseradish peroxidase labeling is most commonly used, together with an appropriate chromogen substrate. The method is more sensitive than the traditional direct technique because multiple secondary antibodies may react with different antigenic sites on the primary antibody, thereby increasing the signal amplification. The technique offers versatility in that the same labeled secondary antibody can be used with a variety of primary antibodies raised from the same animal species.
Polymer chain two-step indirect technique
This technology uses an unconjugated primary antibody, followed by a secondary antibody conjugated to an enzyme (horseradish peroxidase) labeled polymer (dextran) chain (Fig. 18.5c). This dextran chain has up to 70 enzyme molecules and 10 antibody molecules attached. Conjugation of both anti-mouse and anti-rabbit secondary antibodies enables the same reagent to be used for both monoclonal (rabbit and mouse) and polyclonal (rabbit) primary antibodies. The method is biotin free and therefore does not react with endogenous biotin. In addition to being quick, reliable, and easily reproducible, the technique offers great sensitivity. The technique is also useful for multi-color staining on single slide preparations. This technique is probably the most commonly used method in routine diagnostic use. There is a wide choice of commercially available polymer kits including EnVision™+ and FLEX+ from Dako, Novolink and Bond Polymer Refine from Leica, Immpress™ from Vector Laboratories, Excel + from Menarini and ultraVIEW from Ventana.
Unlabeled antibody-enzyme complex techniques (PAP and APAAP)
The original immunoenzyme bridge method using enzyme-specific antibody (Mason et al. 1969; Sternberger 1969) was rapidly superseded by an improved version using a soluble peroxidase-anti-peroxidase complex (PAP) (Sternberger et al. 1970). These complexes are formed from three peroxidase molecules and two anti-peroxidase antibodies (Fig. 18.5d), and are used as a third layer in the staining method. They are bound to the unconjugated primary antibody, e.g. rabbit anti-human IgG, by a second layer of ‘bridging’ antibody that is usually a swine anti-rabbit applied in excess so that one of its two identical binding sites binds to the primary antibody and the other to the rabbit PAP complex.
Alkaline phosphatase antibodies raised in the mouse, by the same principle, can be used to form the alkaline phosphatase-anti-alkaline phosphatase complexes (APAAP). This method, first developed by Cordell et al. in 1984, lends itself to amplification by further application of the bridge and APAAP reagent. For unknown reasons, this form of amplification with the APAAP is not so successful with the PAP technique, and excessive background can sometimes be a serious drawback. These techniques have largely been replaced by streptavidin/biotin methods or polymer-based techniques.
Immunogold silver staining technique (IGSS)
The use of colloidal gold as a label for immunohistochemistry was introduced by Faulk and Taylor (1971). It can be used in both direct and indirect methods and has found wide usage in ultrastructural immunolocalization. It is not widely used in light microscope immunohistochemistry even after the advantages of silver development reported by Holgate et al. (1983a). In this method the gold particles are enhanced by the addition of metallic silver layers (Fig. 18.5e) to produce a metallic silver precipitate which overlays the colloidal gold marker and which can be seen with the light microscope. The technique uses silver lactate as the ion supplier and hydroquinone as the reducing agent in a protective colloid of gum arabic at pH 3.5. In some instances section pretreatment with Lugol’s iodine and sodium after dewaxing and rehydration may be required to improve staining intensity. The method is generally accepted to be more sensitive than the PAP technique, but suffers from the formation of fine silver deposits in the background, especially in inexperienced hands. It can be confusing when trying to identify small amounts of antigen. Modifications to the original technique have been reviewed by De Mey et al. (1986).
(Strept) avidin-biotin techniques
Together with the indirect polymer-based techniques, the labeled streptavidin-biotin technique is the most widely used methodology in diagnostic immunohistochemistry. This is a three-step technique, which has an unconjugated primary antibody as the first layer, followed by a biotinylated secondary antibody (raised against the species of the primary animal). The third layer is either a complex of enzyme-labeled biotin and streptavidin, or enzyme-labeled streptavidin (Fig. 18.5f). The enzyme can be either horseradish peroxidase or alkaline phosphatase, used with a chromogen of choice.
Streptavidin can be isolated from the bacterium Streptomyces avidini, and like avidin it has four high-affinity binding sites for biotin. However, in practice due to the molecular arrangement of these binding sites, fewer than four biotin molecules actually bind. Biotin (vitamin H) is easily conjugated to antibodies and enzyme markers. Up to 150 biotin molecules can be attached to one antibody molecule, often with the aid of spacer arms. By spacing the biotins, the large streptavidin has room to bind and maximize its strong affinity for biotin. The streptavidin-biotin technique can employ either enzyme label bound directly to the streptavidin (Guesden et al. 1979); alternatively, the enzymes are biotinylated and the biotinylated label, forming the streptavidin-biotin complex (Hsu et al. 1981), occupies 75% of the streptavidin-binding sites. Usually the latter is commercially supplied as two separate reagents, biotinylated label and streptavidin, and they are added together 30 minutes before use in order for the complex to form fully. Careful stoichiometric control ensures that some binding sites remain free to bind with the biotinylated secondary antibody. As a large number of biotins can be attached to a single antibody, then numerous labeled streptavidin molecules may be bound on top. This produces increased sensitivity compared to the previously described enzyme techniques and allows a higher dilution of the primary antibody. Tissues rich in endogenous biotin such as liver and kidney will require the use of an avidin/biotin block before applying the primary antibody.
Hapten labeling technique
Bridging techniques using haptens such as dinitro-phenol and arsanilic acid have been advocated (Jasani et al. 1981, 1992). In this technique, the hapten is linked to the primary antibody and a complex is built up using an anti-hapten antibody and either hapten-labeled enzyme or hapten-labeled PAP complex.
Biotinylated tyramide signal amplification
Bobrow et al. first described the use of biotinylated tyramide to enhance signal amplification, in 1989. Subsequent work by Adams in 1992 and King et al. in 1997 enabled the development of a highly sensitive detection system. In conjunction with heat-induced epitope retrieval techniques, the use of biotinylated tyramide amplification enabled many antigens which had previously been unreactive in formalin-fixed paraffin-embedded tissue to be demonstrated. Antibodies could be used at far greater dilutions than in conventional techniques. The biotinylated tyramide amplification reagent was first available commercially from DuPont; this was subsequently followed by the CSA (Catalyzed Signal Amplification) kit from Dako.
The technique is based around the streptavidin-biotin technique. Application of the primary antibody is followed by subsequent incubations in biotinylated secondary antibody and then either horseradish peroxidase-labeled streptavidin or streptavidin-biotin-horseradish peroxidase complex. The critical stage is the subsequent treatment with the biotinylated tyramide amplification reagent. The bound peroxidase label in the presence of hydrogen peroxide catalyses the biotinyl tyramide to form free biotin radicals. These reactive biotin molecules bind covalently to proteins adjacent to the site of the reaction. Further incubation in either horseradish peroxidase-labeled streptavidin or streptavidin-biotin-horseradish peroxidase complex results in additional enzyme being deposited at the site of the reaction (Fig. 18.5g).
Biotin-free Catalyzed Signal Amplification (CSA II)
In an attempt to reduce the problems associated with endogenous biotin in conventional tyramide signal amplification, Dako produces a biotin-free system, available commercially as Catalyzed Signal Amplification system II (CSA II) for use with mouse monoclonal antibodies. Following incubation in primary antibody, a secondary anti-mouse immunoglobulin conjugated to horseradish peroxidase is bound to the primary antibody. The third layer involves peroxidase-catalyzed deposition of fluorescyl tyramide, which in turn is reacted with peroxidase conjugated anti-fluorescein, producing a greatly enhanced signal (Fig. 18.5h). This technique can be adapted easily to automated immunostaining protocols. Although a useful technique, in our hands the results fail to match the high sensitivity of conventional tyramide amplification.
Unmasking of antigen sites
A prerequisite for all routine histological and cytological investigations is to ensure preservation of tissue architecture and cell morphology by adequate and appropriate fixation (Chapter 4). The fixative of choice will depend on the individual laboratory; the most popular choice of fixatives for routine histology are formalin based, either as a 10% solution or with the addition of different chemical constituents. Prompt fixation of thin (3 mm) slices of tissue is essential to achieve consistent demonstration of tissue antigens. Delayed fixation or poor fixation may cause loss of antigenicity or diffusion of antigens into the surrounding tissue. Following fixation most material is routinely processed to paraffin wax to facilitate section cutting.
The concept that antigens can be masked by the chemical processes involved in formalin fixation and paraffin processing and that some form of unmasking of these antigens is required dates far back into the history of immunohistochemistry (Brandtzaeg 1983). The majority of antigen unmasking studies have been applied to formalin-fixed material. When formalin-based fixatives are used, intermolecular and intramolecular cross-linkages are formed with certain structural proteins. These are responsible for the masking of the tissue antigens. This adverse effect has been thought to be the result of the formation of methylene bridges between reactive sites on tissue proteins (Bell et al. 1987; Mason & O’Leary 1991). These reactive sites include primary amines, amide groups, thiols, alcoholic hydroxyl groups, and cyclic aromatic rings. The degree of masking of the antigenic sites depends upon the length of time in fixative, temperature, concentration of fixative, and availability of other nearby proteins able to undergo cross-linkage.
Manual methods for antigen unmasking include:
• Proteolytic enzyme digestion
• Combined microwave oven irradiation and proteolytic enzyme digestion
• Pressure cooker inside a microwave oven
Proteolytic enzyme digestion
Pretreating formalin-fixed routinely processed paraffin sections with proteolytic enzymes to unmask certain antigenic determinants was described by Huang et al. (1976), Curran and Gregory (1977), and Mepham et al. (1979). The most popular enzymes employed today are trypsin and protease, but other proteolytic enzymes such as chymotrypsin, pronase, proteinase K, and pepsin may also be used. The theory behind the unmasking properties of these proteolytic enzymes is not fully understood. Nevertheless, it is generally accepted that the digestion breaks down formalin cross-linking and hence the antigenic sites for a number of antibodies are uncovered.
Heat-mediated antigen retrieval techniques
Heat-based antigen retrieval methods have brought a great improvement in the quality and reproducibility of immunohistochemistry. They have also widened its use as an important diagnostic tool in histopathology. The rationale behind the heat pretreatment methods is unclear and several different theories have been suggested. One of these is that heavy metal salts (as described by Shi et al. 1991) act as a protein precipitant, forming insoluble complexes with polypeptides, and that protein-precipitating fixatives display better preservation of antigens than do cross-linking aldehyde fixatives.
Another possible theory was described by Morgan et al. (1997), who postulated that calcium coordination complexes formed during formalin fixation prevent antibodies from combining with epitopes on tissue-bound antigens. The underlying theory of calcium involvement is that hydroxymethyl groups and other unreacted oxygen-rich groups (e.g. carboxyl or phosphoryl groups) can interact with calcium ions to produce large coordinate complexes which can mask epitopic sites by steric hindrance. The high temperature weakens or breaks some of the calcium coordinate bonds, but the effect is reversible on cooling, because the calcium complex remains in its original position. The presence of a competing chelating agent at the particular temperature at which the coordinate bonds are disrupted removes the calcium complexes. Evidence to support this theory comes from the chemical nature of some of the antigen retrieval reagents, such as citrate buffer and EDTA. In addition it has been shown that the inclusion of calcium ions with an unmasking reagent inhibits its effectiveness (Morgan et al. 1994).
Microwave antigen retrieval
Shi et al. (1991) first established the use of microwave heating for antigen retrieval. However, the use of heavy metal salts posed a significant risk to the health and safety of the users. Gerdes et al. (1992) used microwave antigen retrieval with a non-toxic citrate buffer at pH 6.0 and demonstrated the Ki67 antigen, which had previously thought to be lost during formalin fixation and paraffin processing. The results were equivalent to those seen in frozen sections. Cattoretti et al. (1993) established microwave oven heating as an alternative to proteolytic enzyme digestion. The method improved the demonstration of well-established antibodies such as CD45 and CD20 and enabled the demonstration of a wide range of new antibodies, such as CD8 and p53.
Most domestic microwave ovens are suitable for antigen retrieval and operate at 2.45 GHz, corresponding to a wavelength in vacuo of 12.2 cm (Fig. 18.6). Uneven heating and the production of hot spots have been reported by some workers using the microwave oven. However, by using a volume of buffer between 400 and 600 ml in a suitably sized microwave-resistant plastic container, the problems of uneven heating may be minimized. A batch of 25 slides in a plastic staining rack can be irradiated at one time and accurate, even antigen retrieval achieved. The actual heating time will depend on the following factors:
• Wattage of the oven. Most domestic ovens use a magnetron with an output between 750 and 1000 W. An important point to remember is that the output of the magnetron will decrease with age and frequency of use. The magnetron should be checked for efficiency annually.
• Choice of antigen retrieval buffer.
• Volume of buffer being used.
• Fixation of the tissues under investigation, in terms of fixative used and duration of fixation. This is an important factor, although not as critical as when using proteolytic enzyme digestion. Tissue fixed for extended periods of time will require extended irradiation times. Conversely, poorly fixed tissues may require a reduction in the heating time.
• Thickness of the tissue section: 3 µm sections require less antigen retrieval than 5 µm sections.
• Antigen to be demonstrated. Certain nuclear antigens may require increased heating times.
Pressure cooker antigen retrieval
Norton et al. (1994) suggested the use of the pressure cooker as an alternative to the microwave oven. By using the pressure cooker, Norton et al. (1994) provided evidence that the batch variation and production of hot and cold spots in the microwave oven could be overcome. Pressure cooking is said to be more uniform than other heating methods. A pressure cooker at 15 psi (10.3 kPa) reaches a temperature of around 120°C at full pressure. It is this increased temperature that appears to be a major advantage when unmasking certain nuclear tissue antigens such as bcl-6, p53, p21, estrogen receptor, and progesterone receptor. The demonstration of these antigens can sometimes be weak when using microwave antigen retrieval.
It is preferable to use a stainless steel domestic pressure cooker, because aluminum pressure cookers are susceptible to corrosion from some of the antigen retrieval buffers (Fig. 18.7). The pressure cooker should have a capacity of 4–5 liters, thus allowing a large batch of slides to be treated at the same time. As with the microwave oven, the use of Superfrost Plus microscope slides or strong adhesives such as Vectabond or APES is required.
Steamer
Although quite a popular method in some parts of the world, steam heating appears to be less efficient than either microwave oven heating or pressure cooking (Pasha et al. 1995). Times in excess of 40 minutes are sometimes required, but the method does have the advantage in being less damaging to tissues than the other heating methods. Commercially available rice steamers are adequate for this purpose.
Water bath
Kawai et al. (1994) demonstrated that a water bath set at 90°C was adequate for antigen retrieval. However, by increasing the temperature to 95–98°C, antigen retrieval was improved and the incubation times could be decreased. The technique has the advantage of being gentler on the tissue sections because the temperature is set below boiling point. By using a lower temperature than other heating methods the antigen retrieval buffer does not evaporate and expensive commercial antigen retrieval solutions can be safely reused. The method has the disadvantage in that the antigen retrieval times are increased compared to other methods. This method is recommended with the Dako Hercept test for HER2 expression.
Combined microwave antigen retrieval and trypsin digestion
Advantages of heat pretreatment
Some antigens previously thought lost in routinely processed paraffin-embedded sections are now recovered by heat pretreatment. Many antigens are retrieved by uniform heating times, regardless of length of fixation: e.g., up to several weeks in formal saline (Singh et al. 1993). The demonstration of heavy-chain immunoglobulins is more reliable and reproducible than when proteolytic digestion is employed. The dilution factors of some primary antibodies ascertained with traditional methods can be increased when using heat pretreatment.
Pitfalls of heat pretreatment
Not all antigens are retrieved by heat pre-treatment, and the range of staining of some primary antibodies, for example PGP9.5, a neuroendocrine marker, is altered (Langlois et al. 1994).
Commercial antigen retrieval solutions
There is an ever-growing range of antibodies available. Table 18.1 is intended as a guide only. Individual laboratories need to choose their preferred supplier and finalize the optimum dilutions based on their own sections and the techniques/automation appropriate to their laboratory.
Detection of low levels of antigen
Enhancement and amplification
The optimum dilution of primary antibody for diagnostic immunohistochemistry is defined as the concentration of the primary antibody which gives the optimal specific staining with the least amount of background staining. The optimal dilution will depend upon the type and duration of fixation. Serial dilutions of antibody will often give the distribution of reactivity shown in Figure 18.8.
1. Increasing the concentration of the primary antibody. Usually this can be accomplished with most monoclonals without increasing the background staining significantly, as this type of antibody, especially in the form of tissue culture supernatant, does not contain any non-specific contaminants. Polyclonal antibodies can give excessive background problems and it is advisable to use a casein blocking solution as described in the methods later in this chapter. Sometimes the addition of a small amount of detergent, e.g. 0.01% Tween, to the washes helps to reduce background staining. Further details on dealing with background appear later in the text.
2. Prolonging incubation with the primary antibody overnight, at 4–8°C or at ambient temperature, can enhance staining. Many immunohistochemists employ this methodology for their routine work because higher dilution of primary reagents is achieved, allowing costs to be reduced. Dilutions must not be excessive, otherwise low levels of antigen will not be detected, resulting in false-negative staining.
3. Increasing the concentration of bridge reagent beyond the optimal dilution, or repeated application of the bridge reagent, marginally increases the sensitivity of the avidin-biotin systems. Furthermore, in the case of the CD15 primaries, which are IgM subclass antibodies, LeBrun et al. (1992) reported that an IgM link, as opposed to a broad-spectrum immunoglobulin bridge reagent, improves the rate of detecting CD15-positive Reed-Sternberg and Hodgkin cells (Fig. 18.9). Charalambous et al. not only confirmed this work in 1993, but they also indicated that when microwave antigen recovery was used in place of trypsin, further amplification was achieved.
4. Chemical enhancement of the reaction end-product of the peroxidase-diaminobenzidine method can be achieved by the addition of imidazole (Straus 1982), heavy metals such as copper or cobalt (Hsu & Soban 1982), osmication, or treatment with gold chloride. Colloidal gold labeling can be enhanced dramatically using silver salts with the IGSS technique (Holgate et al. 1983b).
5. Repeated applications of the bridge and label increase the sensitivity of the APAAP technique. Whilst the initial primary, bridge and label are incubated for 30 minutes each, the repeated applications of bridge and label require only 10 minutes each. After two such repeats enhancement is usually sufficient for most antibodies.
6. Changing the chromogen substrate used. Some chromogen substrate solutions, especially for alkaline phosphatase, give a more intense reaction product than other reagents. For example nitro-blue tetrazolium is not only more intense than fast red but also can be left on overnight to give probably the most intense reaction of all chromogens available today. The only drawback is that the blue-black reaction product does not contrast well with hematoxylin counterstaining. Improved commercial formulae of traditional substrates are superior to ‘in house’ formulae.
7. Techniques for elevating the sensitivity of the extended polymer-labeled antibodies and other pre-diluted reagents are more restricted. The disadvantage of pre-diluted antibodies is that the dilutions selected are not necessarily suitable for the multitude of fixation and processing protocols employed. Hence, weak staining can only be overcome by increasing the incubation times, elevating the temperature to 37°C, or chemical enhancement of the diaminobenzidine reaction product, or other appropriate substrate.
8. Tyramide signal amplification. In 1989, Bobrow and fellow workers described a novel signal amplification method, catalyzed reporter deposition, and its application to immunoassays. In 1992 the same group proposed that this method would be suitable for immunohistochemistry. Erber et al. and King et al. in 1997 quite independently published data showing that this novel signal amplification system employed with avidin-biotin systems showed greater sensitivity than that provided by the more conventional avidin-biotin methods.
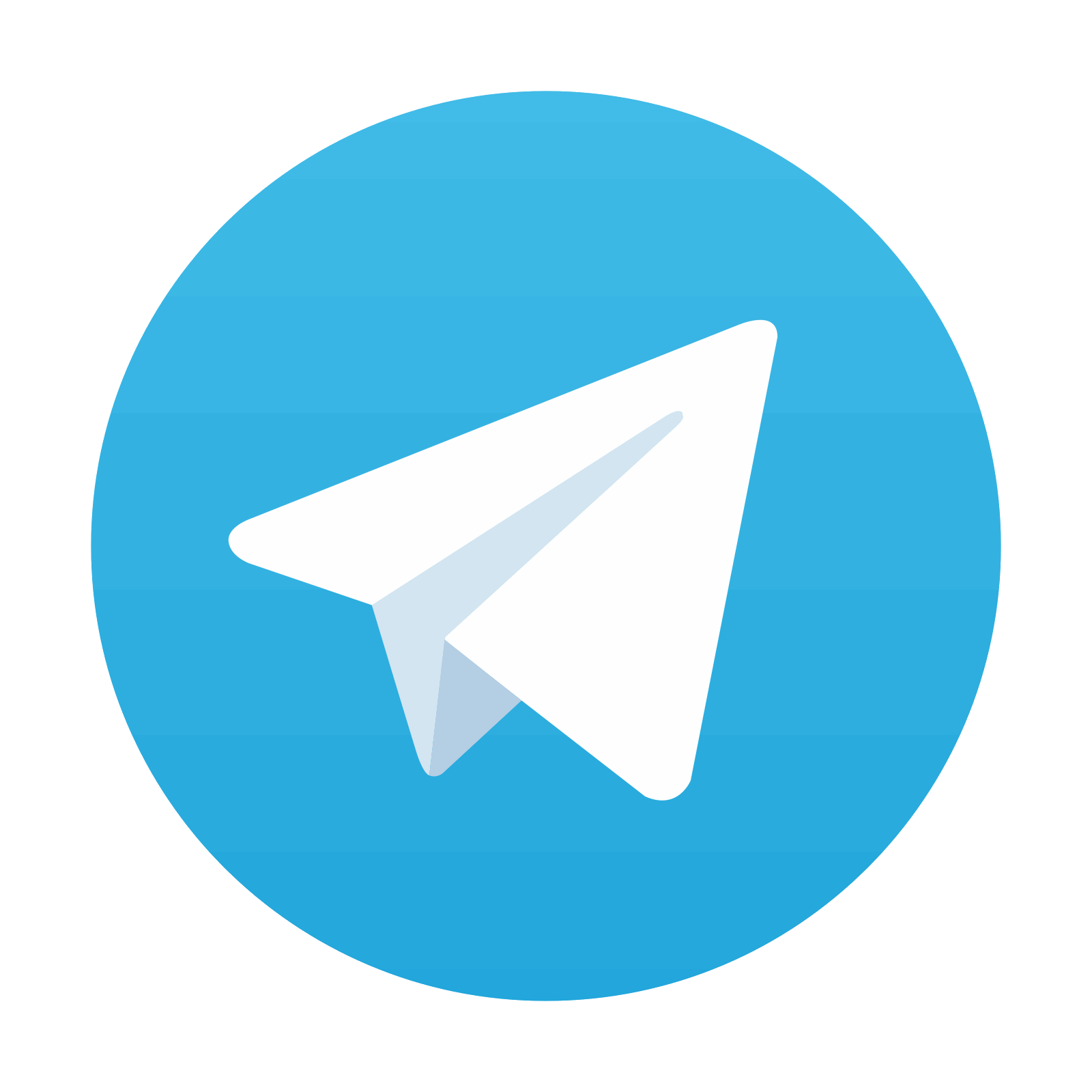
Stay updated, free articles. Join our Telegram channel

Full access? Get Clinical Tree
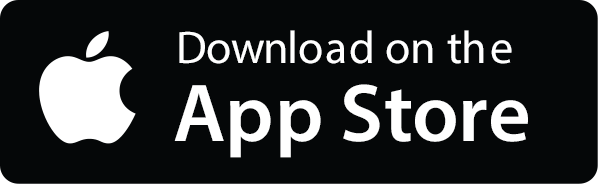
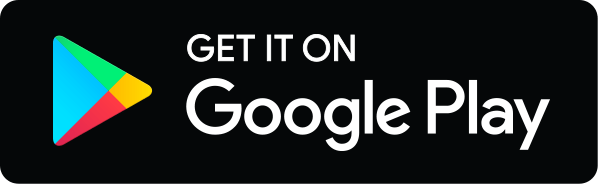