OBJECTIVES
After reading this chapter, you should be able to:
-
Describe the anatomic connections between the hypothalamus and the pituitary gland and the functional significance of each connection.
-
List the factors that control water intake, and outline the way in which they exert their effects.
-
Describe the synthesis, processing, storage, and secretion of the hormones of the posterior pituitary.
-
Discuss the effects of vasopressin, the receptors on which it acts, and how its secretion is regulated.
-
Discuss the effects of oxytocin, the receptors on which it acts, and how its secretion is regulated.
-
Name the hypophysiotropic hormones, and outline the effects that each has an anterior pituitary function.
-
List the mechanisms by which heat is produced in and lost from the body, and comment on the differences in temperature in the hypothalamus, rectum, oral cavity, and skin.
-
List the temperature-regulating mechanisms, and describe the way in which they are integrated under hypothalamic control to maintain normal body temperature.
-
Discuss the pathophysiology of fever.
INTRODUCTION
HYPOTHALAMUS: ANATOMIC CONSIDERATIONS
The hypothalamus (Figure 17–1) is the portion of the anterior end of the diencephalon that lies below the hypothalamic sulcus and in front of the interpeduncular nuclei. It is divided into a variety of nuclei and nuclear areas.
The principal afferent and efferent neural pathways to and from the hypothalamus are mostly unmyelinated. Many connect the hypothalamus to the limbic system. Important connections also exist between the hypothalamus and nuclei in the midbrain tegmentum, pons, and hindbrain.
Norepinephrine-secreting neurons with their cell bodies in the hindbrain end in many different parts of the hypothalamus (see Figure 7–2). Paraventricular neurons that secrete oxytocin and vasopressin project in turn to the hindbrain and the spinal cord. Neurons that secrete epinephrine have their cell bodies in the hindbrain and end in the ventral hypothalamus.
FIGURE 17–2
Secretion of hypothalamic hormones. The hormones of the posterior lobe (PL) are released into the general circulation from the endings of supraoptic and paraventricular neurons, whereas hypophysiotropic hormones are secreted into the portal hypophysial circulation from the endings of arcuate and other hypothalamic neurons. AL, anterior lobe; MB, mamillary bodies; OC, optic chiasm.
An intrahypothalamic system is composed of dopamine-secreting neurons that have their cell bodies in the arcuate nucleus and end on or near the capillaries that form the portal vessels in the median eminence. Serotonin-secreting neurons project to the hypothalamus from the raphe nuclei.
There are neural connections between the hypothalamus and the posterior lobe of the pituitary gland and vascular connections between the hypothalamus and the anterior lobe. Embryologically, the posterior pituitary arises as an evagination of the floor of the third ventricle. It is made up in large part of the endings of axons that arise from cell bodies in the supraoptic and paraventricular nuclei and pass to the posterior pituitary (Figure 17–2) via the hypothalamohypophysial tract. Most of the supraoptic fibers end in the posterior lobe itself, whereas some of the paraventricular fibers end in the median eminence. The anterior and intermediate lobes of the pituitary arise in the embryo from the Rathke pouch, an evagination from the roof of the pharynx (see Figure 18–1). Sympathetic nerve fibers reach the anterior lobe from its capsule, and parasympathetic fibers reach it from the petrosal nerves, but few, if any, nerve fibers pass to it from the hypothalamus. However, the portal hypophysial vessels form a direct vascular link between the hypothalamus and the anterior pituitary. Arterial twigs from the carotid arteries and circle of Willis form a network of fenestrated capillaries called the primary plexus on the ventral surface of the hypothalamus (Figure 17–1). Capillary loops also penetrate the median eminence. The capillaries drain into the sinusoidal portal hypophysial vessels that carry blood down the pituitary stalk to the capillaries of the anterior pituitary. This system begins and ends in capillaries without going through the heart and is therefore a true portal system. In birds and some mammals, including humans, there is no other anterior hypophysial arterial supply other than capsular vessels and anastomotic connections from the capillaries of the posterior pituitary. The median eminence is generally defined as the portion of the ventral hypothalamus from which the portal vessels arise. This region is outside the blood–brain barrier (see Chapter 33).
The major functions of the hypothalamus are summarized in Table 17–1. Some are fairly clear-cut visceral reflexes, and others include complex behavioral and emotional reactions; however, all involve a particular response to a particular stimulus. It is important to keep this in mind in considering hypothalamic function.
Function | Afferents from | Integrating Areas |
---|---|---|
Temperature regulation | Temperature receptors in the skin, deep tissues, spinal cord, hypothalamus, and other parts of the brain | Anterior hypothalamus, response to heat; posterior hypothalamus, response to cold |
Neuroendocrine control of: | ||
Catecholamines Vasopressin Oxytocin Thyroid-stimulating hormone (thyrotropin, TSH) via TRH Adrenocorticotropic hormone (ACTH) and β-lipotropin (β-LPH) via CRH Follicle-stimulating hormone (FSH) and luteinizing hormone (LH) via GnRH Prolactin via PIH and PRH Growth hormone via somatostatin and GRH |
Limbic areas concerned with emotion Osmoreceptors, “volume receptors,” others Touch receptors in breast, uterus, genitalia Temperature receptors in infants, perhaps others Limbic system (emotional stimuli); reticular formation (“systemic” stimuli); hypothalamic and anterior pituitary cells sensitive to circulating blood cortisol level; suprachiasmatic nuclei (diurnal rhythm) Hypothalamic cells sensitive to estrogens, eyes, touch receptors in skin and genitalia of reflex ovulating species Touch receptors in breasts, other unknown receptors Unknown receptors |
Dorsal and posterior hypothalamus Supraoptic and paraventricular nuclei Supraoptic and paraventricular nuclei Paraventricular nuclei and neighboring areas Paraventricular nuclei Preoptic area; other areas Arcuate nucleus; other areas (hypothalamus inhibits secretion) Periventricular nucleus, arcuate nucleus |
“Appetitive” behavior: | ||
Thirst Hunger Sexual behavior |
Osmoreceptors, probably located in the organum vasculosum of the lamina terminalis; angiotensin II uptake in the subfornical organ Glucostat cells sensitive to rate of glucose utilization; leptin receptors; receptors for other polypeptides Cells sensitive to circulating estrogen, androgen, and others |
Lateral superior hypothalamus Ventromedial, arcuate, and paraventricular nuclei; lateral hypothalamus Anterior ventral hypothalamus plus, in the male, piriform cortex |
Defensive reactions (fear, rage) | Sense organs and neocortex, paths unknown | Diffuse, in limbic system and hypothalamus |
Control of body rhythms | Retina via retinohypothalamic fibers | Suprachiasmatic nuclei |
Many years ago, Sherrington called the hypothalamus “the head ganglion of the autonomic system.” Stimulation of the hypothalamus produces autonomic responses, but the hypothalamus does not seem to be concerned with the regulation of visceral function per se. Rather, the autonomic responses triggered in the hypothalamus are part of more complex phenomena such as eating, and emotions such as rage. For example, stimulation of various parts of the hypothalamus, especially the lateral areas, produces diffuse sympathetic discharge and increased adrenal medullary secretion—the mass sympathetic discharge seen in animals exposed to stress (the flight or fight reaction; see Chapter 13).
It has been claimed that separate hypothalamic areas control epinephrine and norepinephrine secretion. Differential secretion of one or the other of these adrenal medullary catecholamines does occur in certain situations (see Chapter 20), but the selective increases are small.
Body weight depends on the balance between caloric intake and utilization of calories. Obesity results when the former exceeds the latter. The hypothalamus and related parts of the brain play a key role in the regulation of food intake. Obesity is considered in detail in Chapter 26, and the relation of obesity to diabetes mellitus is discussed in Chapter 24.
Hypothalamic regulation of sleep and circadian rhythms are discussed in Chapter 14.
Another appetitive mechanism under hypothalamic control is thirst. Drinking is regulated by plasma osmolality and extracellular fluid (ECF) volume in much the same fashion as vasopressin secretion (see Chapter 38). Water intake is increased by increased effective osmotic pressure of the plasma (Figure 17–3), by decreases in ECF volume, and by psychological and other factors. Osmolality acts via osmoreceptors, receptors that sense the osmolality of the body fluids. These osmoreceptors are located in the anterior hypothalamus.
FIGURE 17–3
Relation of plasma osmolality to thirst in healthy adult humans during infusion of hypertonic saline. The intensity of thirst is measured on a special analog scale. (Reproduced with permission from Thompson CJ et al: The osmotic thresholds for thirst and vasopressin release are similar in healthy humans. Clin Sci Lond 1986; Dec; 71(6):651–656.)
Decreases in ECF volume also stimulate thirst by a pathway independent of that mediating thirst in response to increased plasma osmolality (Figure 17–4). Thus, hemorrhage causes increased drinking even if there is no change in the osmolality of the plasma. The effect of ECF volume depletion on thirst is mediated in part via the renin–angiotensin system (see Chapter 38). Renin secretion is increased by hypovolemia and results in an increase in circulating angiotensin II. The angiotensin II acts on the subfornical organ, a specialized receptor area in the diencephalon (see Figure 33–7), to stimulate the neural areas concerned with thirst. Some evidences suggest that it acts on the organum vasculosum of the lamina terminalis (OVLT) as well. These areas are highly permeable and are two of the circumventricular organs located outside the blood–brain barrier (see Chapter 33). However, drugs that block the action of angiotensin II do not completely block the thirst response to hypovolemia, and it appears that the baroreceptors in the heart and blood vessels are also involved.
The intake of liquids is increased during eating (prandial drinking). The increase has been called a learned or habit response, but it has not been investigated in detail. One factor is an increase in plasma osmolality that occurs as food is absorbed. Another may be an action of one or more gastrointestinal hormones on the hypothalamus.
When the sensation of thirst is obtunded, either by direct damage to the diencephalon or by depressed or altered states of consciousness, patients stop drinking adequate amounts of fluid. Dehydration results if appropriate measures are not instituted to maintain water balance. If the protein intake is high, the products of protein metabolism cause an osmotic diuresis (see Chapter 38), and the amounts of water required to maintain hydration are large. Most cases of hypernatremia are actually due to simple dehydration in patients with psychoses or hypothalamic disease who do not or cannot increase their water intake when their thirst mechanism is stimulated. Lesions of the anterior communicating artery can also obtund thirst because branches of this artery supply the hypothalamic areas concerned with thirst.
A number of other well-established factors contribute to the regulation of water intake. Psychological and social factors are important. Dryness of the pharyngeal mucous membrane causes a sensation of thirst. Patients in whom fluid intake must be restricted sometimes get appreciable relief of thirst by sucking ice chips or a wet cloth.
Dehydrated dogs, cats, camels, and some other animals rapidly drink just enough water to make up their water deficit. They stop drinking before the water is absorbed (while their plasma is still hypertonic), so some kind of pharyngeal gastrointestinal “metering” must be involved. Some evidence suggests that humans have a similar metering ability, though it is not well developed.
CONTROL OF POSTERIOR PITUITARY SECRETION
In most mammals, the hormones secreted by the posterior pituitary gland are arginine vasopressin (AVP) and oxytocin. In hippopotami and most pigs, arginine in the vasopressin molecule is replaced by lysine to form lysine vasopressin. The posterior pituitaries of some species of pigs and marsupials contain a mixture of arginine and lysine vasopressin. The posterior lobe hormones are nanopeptides with a disulfide ring at one end (Figure 17–5).
The hormones of the posterior pituitary gland are synthesized in the cell bodies of the magnocellular neurons in the supraoptic and paraventricular nuclei and transported down the axons of these neurons to their endings in the posterior lobe, where they are secreted in response to electrical activity in the endings. Some of the neurons make oxytocin and others make vasopressin, and oxytocin-containing and vasopressin-containing cells are found in both nuclei.
Oxytocin and vasopressin are typical neural hormones, that is, hormones secreted into the circulation by nerve cells. This type of neural regulation is compared with other types in Figure 17–6. The term neurosecretion was originally coined to describe the secretion of hormones by neurons, but the term is somewhat misleading because it appears that all neurons secrete chemical messengers (see Chapter 7).
FIGURE 17–6
Neural control mechanisms. In the two situations on the left, neurotransmitters act at nerve endings on muscle; in the two in the middle, neurotransmitters regulate the secretion of endocrine glands; and in the two on the right, neurons secrete hormones into the hypophysial portal or general circulation.
Like other peptide hormones, the posterior lobe hormones are synthesized as part of larger precursor molecules. Vasopressin and oxytocin each have a characteristic neurophysin associated with them in the granules in the neurons that secrete them—neurophysin I in the case of oxytocin and neurophysin II in the case of vasopressin. The neurophysins were originally thought to be binding polypeptides, but it now appears that they are simply parts of the precursor molecules. The precursor for AVP, prepropressophysin, contains a 19-amino-acid residue leader sequence followed by AVP, neurophysin II, and a glycopeptide (Figure 17–7). Prepro-oxyphysin, the precursor for oxytocin, is a similar but smaller molecule that lacks the glycopeptide.

Full access? Get Clinical Tree
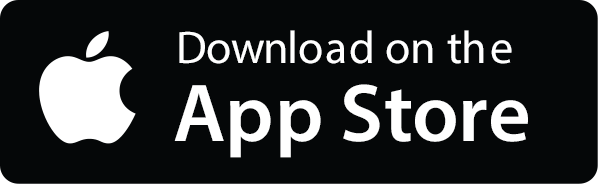
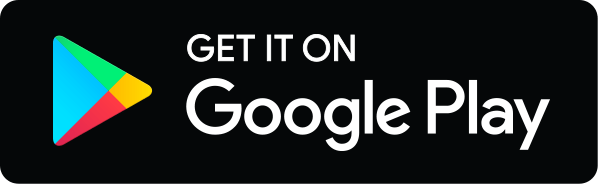