HLA-G function
HLA-G isoforms involved
Immune cells involved
References
Inhibition of NK- and T-cell-mediated cell lysis
Primarily HLA-G1 and HLA-G5
Also shown for the other alternatively spliced HLA-G isoforms (HLA-G2 to HLA-G4 and HLA-G6); however, an in vivo functionality is controversial
Decidual and peripheral NK cells
CD8+ cytotoxic T cells
Inhibition of an allocytotoxic T lymphocyte response
HLA-G1 and soluble HLA-G (HLA-G5 and/or sHLA-G1)
Peripheral blood mononuclear cells (PBMCs)
Upregulation of inhibitory receptors
HLA-G1 and HLA-G5
NK cells and CD4+ T cells
LeMaoult et al. (2005)
Shift from a proinflammatory Th1 response to a Th2 response
HLA-G1 and soluble HLA-G (HLA-G5 and/or sHLA-G1)
CD4+ T cells
Induction of CD4+ T cell anergy/long-term unresponsiveness
HLA-G1
CD4+ T cells
LeMaoult et al. (2004)
Possible induction of FoxP3-regulatory T cells
HLA-G5 (and HLA-G1?)
CD4+CD25+FoxP3+ Tregs
Dendritic cells
Dendritic cell induction and immunosuppression
CD4+ and CD8+ T cells
8.3.3 HLA-G Isoforms and Gene Polymorphism
HLA-G potentially exists in four membrane-bound isoforms, HLA-G1, HLA-G2, HLA-G3, and HLA-G4, and three soluble isoforms, HLA-G5, HLA-G6, and HLA-G7, all generated by alternative splicing of HLA-G mRNA (Ishitani and Geraghty 1992; Fujii et al. 1994; Hviid et al. 1998). The secreted soluble HLA-G isoforms are generated by the retention of intron 4 that includes a stop codon (Fujii et al. 1994; Hviid et al. 1998). The most important isoforms are the full-length membrane-bound isoform HLA-G1 and the full-length secreted isoform HLA-G5. Soluble HLA-G1 (sHLA-G1) is generated by the shedding of membrane-bound HLA-G1 molecules (Park et al. 2004).
In contrast to the extremely polymorphic HLA class Ia genes, the HLA-G gene is almost monomorphic. HLA-G has a low polymorphism in the coding regions (Hviid 2006; Dahl and Hviid 2012). According to the WHO Nomenclature Committee for Factors of the HLA System and the International Immunogenetics Information System (IMGT)/HLA Database, 18 HLA-G alleles have been described at the protein level; two of these are so-called null alleles coding for—at least some—nonfunctional protein isoforms. Table 8.2 shows a comparison between the numbers of reported alleles for some of the HLA class Ia genes and HLA-G. In short, only very few amino acid substitutions have been observed in the HLA-G protein. However, quite many single nucleotide polymorphisms (SNPs) have been described in the 5′-upstream regulatory region (5′URR) and in the 3′-untranslated region (3′UTR) of the HLA-G gene (Hviid et al. 1999; Ober et al. 2003; Castelli et al. 2011). In particular, a 14 bp insertion/deletion polymorphism in the 3′UTR has been widely studied in relation to HLA-G mRNA alternative splicing and HLA-G expression levels. This polymorphism has been associated with the risk of developing preeclampsia, recurrent spontaneous abortion, and the success of assisted reproduction, although not all studies show such associations (Harrison et al. 1993; Hviid et al. 2002, 2004b; Hylenius et al. 2004; Larsen et al. 2010; Iversen et al. 2008).
Table 8.2
A comparison between the total number of DNA alleles and protein alleles of some of the classical HLA class Ia genes and the nonclassical HLA class Ib gene, HLA-G, according to the official WHO Nomenclature Committee for Factors of the HLA System and the International Immunogenetics Information System [IMGT/HLA Database (October 2014)]. HLA class Ia gene loci are among the most polymorphic genes in the human genome, whereas HLA-G at the protein level is nearly monomorphic
Gene locus | HLA-A | HLA-B | HLA-G |
---|---|---|---|
Class Ia | Class Ib | ||
Alleles (DNA) | 2,946 | 3,693 | 50 |
Proteins | 2,077 | 2,741 | 18 |
Null alleles | 138 | 122 | 2 |
8.4 HLA Class Ib Molecules During Pregnancy
Follicular fluid has been shown to contain sHLA-G, as well as the matured cumulus–oocyte complex (Rizzo et al. 2009b). A range of studies have reported the expression of HLA-G mRNA and protein in blastocysts (Jurisicova et al. 1996; Yao et al. 2005; Verloes et al. 2011). Furthermore, consensus from a rather large number of studies is that the media from 2- to 3-day-old embryo cultures from in vitro fertilization treatments is positive for sHLA-G in approximately 30–40 % of the cases, although not supported by all studies (Fuzzi et al. 2002; Van Lierop et al. 2002; Sher et al. 2004; Noci et al. 2005; Yao et al. 2005; Sageshima et al. 2007; Vercammen et al. 2008; Kotze and Kruger 2013). Interestingly, a considerable number of independent studies have observed that the pregnancy rate in women who have embryos transferred from cultures, where sHLA-G is detected, is significantly higher than that in women, who have embryos transferred from sHLA-G-negative cultures (Fuzzi et al. 2002; Sher et al. 2004; Noci et al. 2005; Yie et al. 2005; Vercammen et al. 2008; Kotze and Kruger 2013). However, the source of the sHLA-G, or the detection assays, is controversial because it does not seem plausible that the two- to eight-cell blastocyst should be capable of producing the amounts of sHLA-G measured (Sargent et al. 2007). At least some of the sHLA-G might originate from follicular fluid adhering to the oocyte. One study has observed a significant association between an increased cleavage rate and detection of sHLA-G, and another study reported that downregulation of HLA-G attenuates the cleavage rate in human triploid embryos, which to some extent may explain the higher chance of pregnancy associated with positive detection of HLA-G (Yie et al. 2005; Sun et al. 2011). Additionally, the study by Yie et al. has also reported that although pregnancy and live births were observed in sHLA-G-negative IVF cycles, the rate of spontaneous abortions was higher in the HLA-G-negative group (25 %) versus the HLA-G-positive group (11 %) (Yie et al. 2005). Together, all these different studies support a role for HLA-G very early in pregnancy and even at the time of implantation.
Another interesting observation is a study of sHLA-G in maternal blood during early pregnancy after IVF treatment (Pfeiffer et al. 2000). The concentration of sHLA-G in serum samples from 20 women experiencing early spontaneous abortion was significantly reduced during the first 9 weeks of gestation, compared with those of 37 women with intact pregnancies. Even the mean preovulatory sHLA-G serum levels in the 20 women were significantly lower than the mean level in the women with successful pregnancies. These results are supported by another study by Sipak-Szmigiel et al. (2007); however, larger studies are needed to reproduce these initial findings. Soluble HLA-G concentrations in the maternal peripheral blood are two to five times higher than the levels in nonpregnant women and in men; it peaks at the end of the first trimester and the beginning of the second (Alegre et al. 2007; Rizzo et al. 2009a; Darmochwal-Kolarz et al. 2012). Almost all studies have reported significantly reduced sHLA-G levels in maternal blood in cases of preeclampsia in all three trimesters (Hackmon et al. 2007; Steinborn et al. 2007; Rizzo et al. 2009a; Bortolotti et al. 2012).
8.4.1 Expression of HLA Molecules in the Placenta
During pregnancy, HLA-G is expressed by the trophoblast cells in the placenta, especially the extravillous trophoblast cells that invade the uterine wall and the spiral arteries (Kovats et al. 1990; Le Bouteiller and Blaschitz 1999; Morales et al. 2003; Proll et al. 1999; Ishitani et al. 2003) (Fig. 8.1). It is in this feto-maternal contact zone that the HLA-G-expressing trophoblast cells, both as membrane-bound HLA-G and sHLA-G, are in intimate contact with the maternal immune cells. The leukocyte population in the decidua contains approximately 10 % T cells, 20 % macrophages, and 70 % NK cells (Loke et al. 1995). Therefore, the CD16−/lowCD56high NK cells represent the largest population of lymphocytes in the placenta, constituting 50–90 % of all resident leukocytes according to different studies (Bulmer et al. 1991; Koopman et al. 2003).
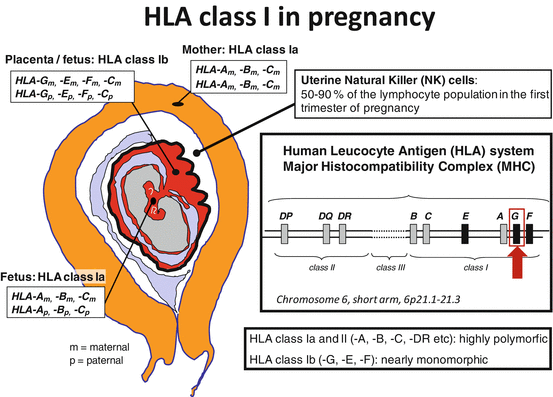
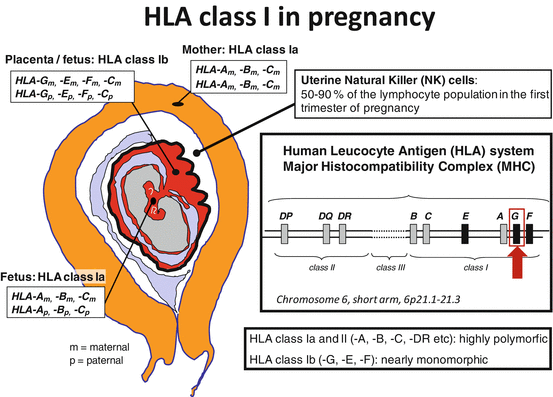
Fig. 8.1
Expression of human leukocyte antigen (HLA) molecules at the feto-maternal contact zone during pregnancy. The fetus inherits one HLA haplotype from the mother and one from the father; thereby, the fetus is semi-allogenic for the mother. The very polymorphic classical HLA class Ia and II molecules are not expressed by the trophoblast cells in the placenta, except for HLA-C. HLA class Ib proteins (HLA-E, HLA-F, and HLA-G) are expressed on the extravillous and invasive trophoblast cells, and they interact with specific receptors on uterine immune cells, especially natural killer (NK) cells. In this way, the trophoblast cells escape NK-cell-mediated lysis, and regulatory T cells may also be induced with the involvement of dendritic cells [Figure modified from (Hviid 2006)]
The extravillous trophoblast cells also express the other two HLA class Ib molecules, HLA-E and HLA-F, and may be the only cells in the body that do so (Ishitani et al. 2003) (Fig. 8.1). The trophoblast cells also express HLA-C at an apparently low level but not HLA-A or HLA-B or HLA class II molecules (King et al. 2000). Again, almost all studies have shown a significantly reduced expression of HLA-G mRNA and protein in the placenta in cases of preeclampsia compared to control pregnancies (Hara et al. 1996; Goldman-Wohl et al. 2000; Yie et al. 2004; Zhu et al. 2012).
8.5 HLA-G Expression in the Male Reproductive System
Most of the studies on HLA-G expression and function in reproduction have focused entirely on the female reproductive system, on pregnancy, and on certain pregnancy complications. However, two early studies of HLA class Ib gene expression in male gametogenic cells have been conducted (Guillaudeux et al. 1996; Fiszer et al. 1997). Guillaudeux et al. detected low levels of HLA class Ib mRNA in both spermatocytes and spermatids; three different alternatively spliced HLA-G mRNA isoforms were detected corresponding to HLA-G1, HLA-G2, and HLA-G3 (Guillaudeux et al. 1996). On the other hand, the same study did not observe any production of detectable HLA class I proteins in spermatogenic cells. These findings are partly in contrast to a study by Fiszer et al. that investigated HLA class Ib mRNA expression in male gametogenic cells from testicular tissue (Fiszer et al. 1997). Considerable levels of HLA-E mRNA were observed, very low levels of HLA-F, and no expression of HLA-G mRNA, even with RT-PCR techniques. HLA-E protein was observed on cells of the adluminal compartment within the seminiferous tubules. A new study by Yao et al. investigated HLA-G mRNA expression in testicular tissue with Johnson scores of 2–9 (Yao et al. 2014). The Johnson scoring system is a method of evaluating the quality of spermatogenesis in testicular biopsies. The HLA-G mRNA levels were significantly higher in testicular tissues with spermatocytes than those with only Sertoli cells and/or spermatogonia. Interestingly, the expression of HLA-G mRNA increased with higher Johnson score of the testicular tissue indicating an important role for HLA-G in spermatogenesis. In this study, HLA-G mRNA expression was also detected in ejaculated sperm. Investigation of HLA-G protein expression was not performed. Interestingly, by using siRNA techniques, it was found in the same study that silencing of the HLA-G gene impaired embryonic development indicating an important role for HLA-G in early pregnancy.
Langat et al. were the first to report the expression of HLA-G mRNA and protein in the normal human prostate (Langat et al. 2006). It was possible to detect mRNA for HLA-G1, HLA-G2, HLA-G5, and HLA-G6. However, only HLA-G5 protein was detectable. The HLA-G5 protein was prominent in the cytoplasm of tubuloglandular epithelia and in glandular secretions. In cases of prostatic adenocarcinomas, the HLA-G5 protein was detectable mainly in secretions.
Given this background—many studies of HLA-G in the female reproductive cycle and during pregnancy, and only a few published studies of specific issues regarding HLA-G in the male reproductive system—we decided to perform a systematic study of HLA-G protein expression in the male reproductive organs (Larsen et al. 2011). Immunohistochemical staining with the use of four different anti-HLA-G monoclonal antibodies (mAbs), two specific for all HLA-G isoforms and two specific for the soluble isoforms HLA-G5 and HLA-G6, was performed on paraffin-embedded tissue samples. Normal testis, testis with atrophy, prostate with hyperplasia, normal epididymis, normal ductus deferens, and normal seminal vesicle were studied. We detected HLA-G protein expression in normal testis in some of the Sertoli cells and in epididymal tissue. The ductuli efferentes stained very strongly for HLA-G. There was a weak expression of HLA-G in hyperplastic prostatic tissue. Only mAbs against the soluble HLA-G isoforms stained positive, suggesting that soluble HLA-G5 is the predominantly expressed HLA-G protein isoform in the male reproductive organs. This is consistent with the findings of Langat et al. in the prostate (Langat et al. 2006). The seminal vesicle was negative for HLA-G protein expression (Larsen et al. 2011). Cells in seminal samples that were immobilized in a plasma–thrombin gel and paraffin embedded all stained negative for HLA-G indicating that leukocytes in the semen do not seem to contribute to sHLA-G in the seminal plasma.
However, based on Western blotting techniques and a sHLA-G ELISA, we detected sHLA-G in seminal plasma samples and in sperm samples. At least some of this sHLA-G was the HLA-G5 isoform (Larsen et al. 2011; Dahl et al. 2014). In a pilot study, we observed highly varied amounts of sHLA-G in seminal plasma samples from different men. This was also the case when the sHLA-G concentration was standardized to total protein concentration in the seminal plasma sample (Larsen et al. 2011). A very large variation in sHLA-G levels in seminal plasma samples was confirmed in a follow-up study of the male partners of 54 unselected couples attending a fertility clinic (Dahl et al. 2014).
It is possible that HLA-G in the testis might have a functional role serving as an immunosuppressive factor, thereby avoiding recognition of “self” sperm cells considered as autoantigens for the immune system. In this way, HLA-G might be a local factor among several that maintains the testes as an immune-privileged site. In support of this, the Sertoli cells seem to be immunoprotective, and they seem to locate HLA-G as described above (Mital et al. 2010; Larsen et al. 2011).
Interestingly, it has been reported that the rhesus monkey carries a nonclassical MHC class I gene named Mamu-AG (Ryan et al. 2002). The expression of Mamu-AG is very similar to HLA-G, and it is a putative homolog of HLA-G. Mamu-AG shares a number of features of HLA-G: generation of alternatively spliced mRNA isoforms, relatively low level of polymorphism, and a high level of expression at the feto-maternal interface (Ryan et al. 2002). However, Mamu-AG is also expressed as a soluble isoform, Mamu-AG5, in the rhesus monkey testis; it is generated by a premature stop codon in intron 4, just as in the case of HLA-G. The Sertoli cells were positive for Mamu-AG in immunostaining experiments. Semen or seminal plasma was not investigated, but late-stage primary and secondary spermatocytes and spermatids were positive for Mamu-AG5, while mature sperm was negative (Ryan et al. 2002). These similar observations across different species support a possible important role of MHC class Ib molecules in the male reproductive system and that they may serve a function in semen even before conception, at conception, and in very early stages of pregnancy.
8.6 HLA-G Genetics Influence HLA-G Protein Concentrations in Seminal Plasma
Several studies have shown significant associations between specific HLA-G genotypes, alleles and haplotypes, and different levels of soluble HLA-G in the blood from nonpregnant donors (Hviid et al. 2004a; Chen et al. 2008; Di Cristofaro et al. 2013; Martelli-Palomino et al. 2013). Therefore, we investigated whether soluble HLA-G levels in seminal plasma samples were associated with the HLA-G genotype of the men. We studied the concentration of sHLA-G in seminal plasma samples and the HLA-G 14 bp ins/del genotype in 40 men, half of them with reduced semen quality (Dahl et al. 2014). The concentration of sHLA-G in the seminal plasma samples was significantly associated with the HLA-G 14 bp ins/del genotype of the men. The del 14 bp/del 14 bp genotype showed the highest level of sHLA-G, and the ins 14 bp/ins 14 bp genotype showed the lowest level. These findings are exactly the same as reported for sHLA-G in blood plasma, or serum, in relation to the HLA-G 14 bp genotype (Hviid et al. 2004a; Chen et al. 2008). Measurements of total protein concentration in the seminal plasma samples were also performed to compensate for semen sample concentration. The same significant differences were observed, when the sHLA-G concentration in the seminal samples was corrected by the total protein concentration expressed as the ratio of sHLA-G to total protein. Furthermore, the same pattern was observed for the total amount of sHLA-G protein in the seminal sample obtained by multiplying the volume of semen with the sHLA-G concentration (Dahl et al. 2014). In conclusion, HLA-G genetics of the man clearly influences the amount of sHLA-G in his semen.
8.7 Immunomodulatory Factors in Seminal Fluid Influence the Female Immune Response
Several studies have indicated that repeated exposure to semen in animal models and in humans, respectively, improves reproductive success (Robertson et al. 2003; Robertson 2005). In mice, it seems that “uterine priming” with semen can promote implantation and fetal growth in subsequent pregnancies, even in a partner-specific manner (Beer et al. 1975). It seems that seminal fluid elicits an inflammation-like response in the female genital tract. Thereby, immune adaptations that can advance conception and pregnancy may be activated (Sharkey et al. 2012). In humans, live birthrates in couples undergoing IVF are significantly improved, when women are exposed to semen at the time of embryo transfer (Tremellen et al. 2000). In fertile women, immune cells and immune factors have been studied in cervix biopsies 12 h after unprotected vaginal coitus, vaginal coitus with the use of condom, or no coitus. After unprotected coitus, seminal fluid induced the recruitment of leukocytes and changes in cytokine and chemokine expression in the cervix and vagina (Sharkey et al. 2012).
8.7.1 HLA-G in Seminal Plasma Might Have Implications for Pregnancy Success
Several factors in seminal plasma may be involved in the modulation of the inflammatory response in the cervix and in the uterus. Two candidates for induction of tolerance to seminal antigens are transforming growth factor-β (TGF-β) and prostaglandin E2, which can be detected at high concentrations within mammalian semen (Robertson et al. 2003; Robertson 2005). However, our studies also indicate sHLA-G as a possible tolerance-inducible and “priming” factor in human seminal fluid (Fig. 8.2). Regulatory T cells (Tregs) and tolerogenic dendritic cells of the woman are most likely to be important in this immunomodulation (Robertson et al. 2013). Interestingly, soluble HLA-G5 may be involved in the induction of CD4+CD25highFoxP3+ Tregs (Selmani et al. 2008), and HLA-G seems to be implicated as a key regulator of tolerogenic dendritic cells (Ristich et al. 2005; Gregori et al. 2010; Amodio et al. 2013).
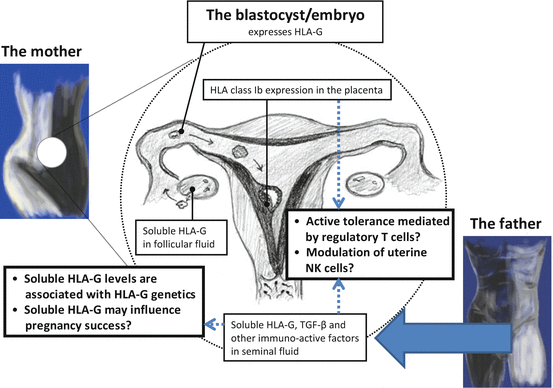
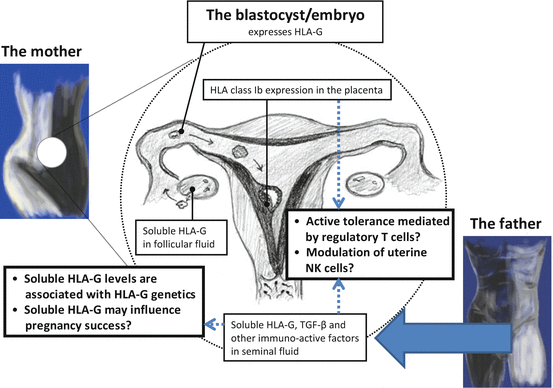
Fig. 8.2
Human leukocyte antigen-G is expressed in almost all of the phases of the reproductive cycle. Therefore, a central and important role for HLA-G in reproduction may be postulated. As shown, HLA-G is present in maternal blood, in follicular fluid, and in seminal plasma prior to implantation. After fertilization, membrane-bound HLA-G and secreted soluble HLA-G are expressed by the extravillous trophoblast cells in the placenta. The expression of HLA-G in the reproductive system during the reproductive cycle may modulate the local immune cells in the female reproductive organs toward immune tolerance of the semi-allogenic embryo. HLA-G gene polymorphisms influence HLA-G protein expression. Aberrant expression or reduced levels of HLA-G may influence pregnancy success and may modulate the risk of certain pregnancy complications, which seem to include immune maladaptation, such as preeclampsia [Figure modified from (Nilsson et al. 2014)]
In a pilot study of 54 unselected couples attending a fertility clinic, a trend for higher seminal plasma levels of sHLA-G per total protein and total sHLA-G in cases with reduced semen quality was observed when the female partner became pregnant after ART, compared with those couples, where no pregnancy was achieved (Dahl et al. 2014). Therefore, the amount of sHLA-G that the woman is exposed to before and at conception, especially in the genital tract, may influence the chance of obtaining a pregnancy. Most of the female partners to the males in the subgroup with reduced semen quality had normal fertility according to the results of the standard medical examination for female factors influencing fertility. It can be speculated that following successful ART procedures, these women might have been able to provide an optimal immunological response to high levels of sHLA-G in the semen of the partner (Dahl et al. 2014).
In conclusion, repeated female exposure to semen and paternal factors therein may be important for the success of pregnancy. One of these factors might be sHLA-G generating a state of local and maybe specific immunomodulation in the woman.
8.8 A Possible Importance of Seminal sHLA-G in Relation to the Risk of Developing Preeclampsia
Preeclampsia can be a very serious pregnancy complication, and it occurs in 2–8 % of all pregnancies. In the second half of pregnancy, the woman develops hypertension and proteinuria, which can be complicated by activation of the coagulation system and disseminated intravascular coagulation. Preeclampsia is a leading cause of maternal and fetal morbidity and mortality. The fetus and especially the placenta are central to the development of the syndrome, and in most cases, the symptoms disappear rapidly after delivery (Redman and Sargent 2005; Ahmed and Ramma 2014). Preeclampsia has been named the “disease of theories.” However, a popular hypothesis for the etiology and pathogenesis of preeclampsia involves immune maladaptation in the early phases of pregnancy and placentation (Dekker and Sibai 1998; Saito et al. 2007). Experimental evidence exists for abnormal immunomodulation in the pregnant woman with preeclampsia when compared with uncomplicated pregnancies. This involves in cases of preeclampsia compared to controls: reduced fractions of regulatory FoxP3+ T cells and CD4+HLA-G+ T cells in peripheral blood (Toldi et al. 2008; Santner-Nanan et al. 2009; Hsu et al. 2014), an apparently skewing of the immune response from T helper 2 (Th2) response toward a proinflammatory Th1 response (Darmochwal-Kolarz et al. 1999), reduced levels of sHLA-G in maternal blood (Hackmon et al. 2007; Steinborn et al. 2007; Rizzo et al. 2009a; Darmochwal-Kolarz et al. 2012), and aberrant expression of HLA-G in placentas (Goldman-Wohl et al. 2000; Yie et al. 2004; Zhu et al. 2012). In addition, several other immune parameters have been reported to be abnormal in cases of preeclampsia.
8.8.1 Epidemiological Observations Support Immune Maladaptation as a Possibly Important Factor in Developing Preeclampsia
It can be speculated that an abnormal exposure to immunomodulatory and tolerogenic factors in semen, either by reduced exposure or low concentration of these factors, might influence the fate of the pregnancy and especially the risk of developing preeclampsia. This has led to a theory of inadequate fetal, or paternal, tolerance induction in cases of preeclampsia, and this might already be of importance before conception involving a mechanism of immunological “priming” of the woman before or at conception. Several epidemiological observations support this and the hypothesis of immune maladaptation as an important factor in the development of preeclampsia: (1) preeclampsia is much more frequent in primipara/primigravida; (2) preeclampsia is more frequent in women with some of the autoimmune diseases, e.g., type 1 diabetes; and (3) there might be a higher risk of developing preeclampsia in a subsequent pregnancy for multipara, who changes partner. However, this may simply be attributed to a higher risk of preeclampsia as a consequence of longer duration to the next pregnancy according to one large study (Skjaerven et al. 2002). Furthermore, the use of donor sperm instead of partner (homologous) sperm in intrauterine insemination treatments seems to increase the risk of developing preeclampsia indicating a partner-specific dimension in a possible immunological “priming” of the woman (Gonzalez-Comadran et al. 2014). Finally, the sexual relationship with the father before preeclampsia seems to influence the risk of developing preeclampsia. A short sexual relationship with the father increases the risk of preeclampsia (Kho et al. 2009), and the use of barrier methods (condom and pessary) as contraception increases the risk of preeclampsia in a subsequent pregnancy (Einarsson et al. 2003). A recent study of 258 preeclampsia cases and 182 normotensive controls has confirmed that the risk of developing preeclampsia decreases significantly with increasing vaginal exposure to paternal semen (Saftlas et al. 2014). HLA typing for mother–offspring pairs, both cases and controls, was also performed for HLA-A, HLA-B, HLA-C, HLA-DRB1, and HLA-DQB1. The authors observed that HLA-A matching (or sharing), HLA class Ia matching, and combined HLA class Ia and II matching were associated with increased odds of preeclampsia (Triche et al. 2014). Very interestingly, the association with preeclampsia was influenced by prior vaginal exposure to paternal seminal fluid. For women with low semen exposure, the effects of HLA class Ia matching were amplified. With moderate to high semen exposure, HLA class II matching effects were predominant.
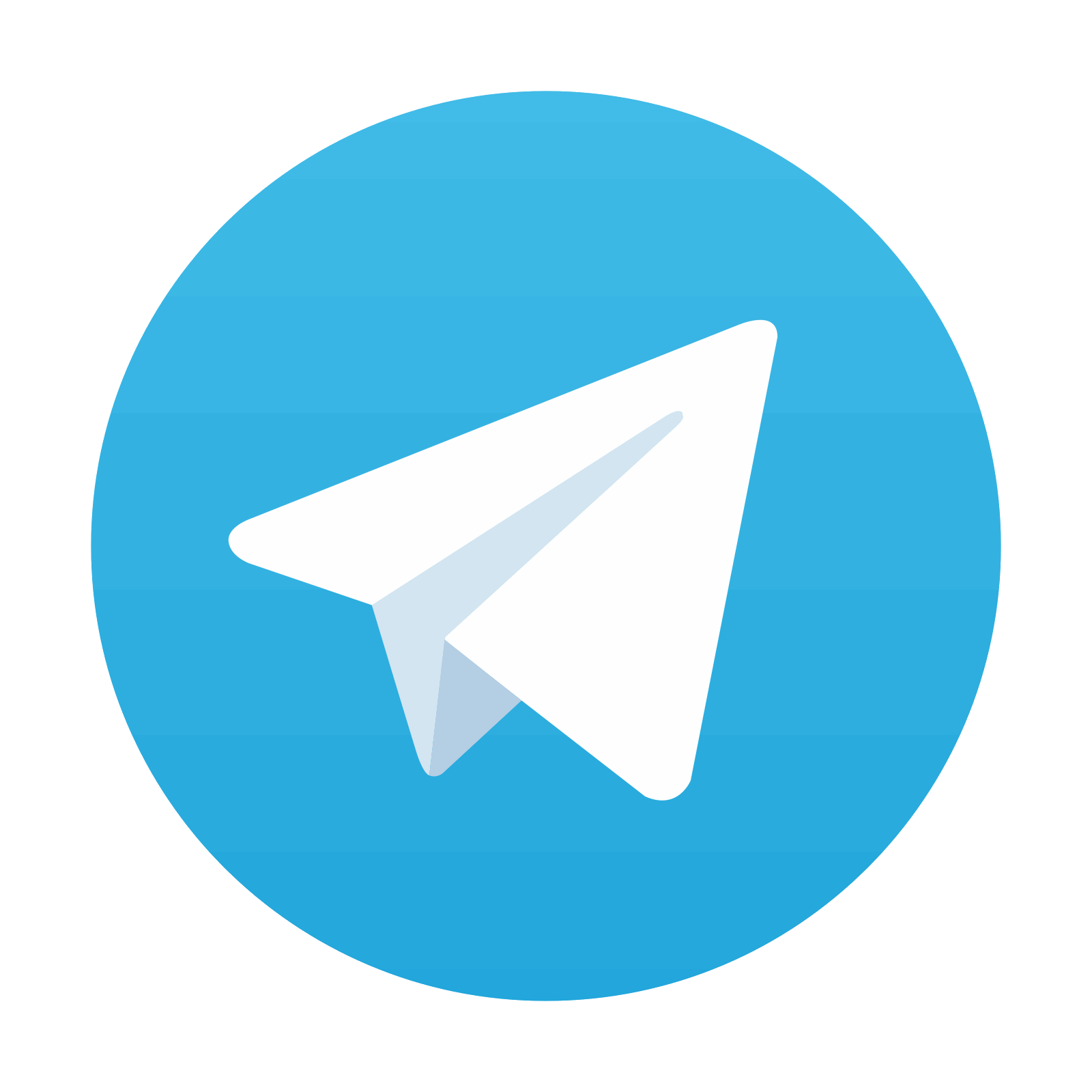
Stay updated, free articles. Join our Telegram channel

Full access? Get Clinical Tree
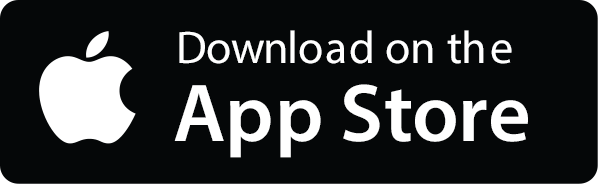
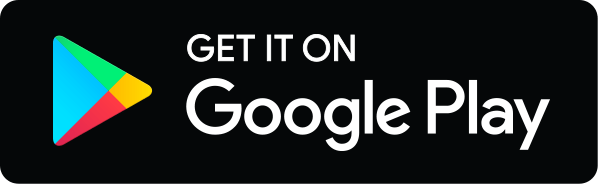