This chapter reviews recent studies from this group on adaptive immune responses to rotavirus (RV) and correlates of protection against disease. The main findings are that both circulating memory RV-specific B and T cells preferentially express intestinal homing receptors, and that both types of lymphocyte populations are enriched in cells with peculiar functions: while B cells are enriched in an innate immunity subset, T cells have a poor functional profile. These characteristics are probably due to the fact that these lymphocytes are activated and proliferate in a tolerogenic intestinal microenvironment (the predominant site of RV replication), making RV infection an excellent model to study lymphocytes from the gut-associated lymphoid tissue. This knowledge may also be useful to define better correlates of protection against the disease.
Human Acquired Immunity to Rotavirus Disease and Correlates of Protection
Abstract
Keywords
1. Introduction
2. Rotavirus-specific T cells
2.1. Rotavirus-Specific CD4 T Cells

Fresh PBMC from healthy adults were cultured with tetanus toxoid, influenza virus antigen, and RV antigens in the presence of anti-CD28 and anti-CD49d monoclonal antibodies (MAbs) for 10 h; brefeldin A was added for the last 5 h of incubation. (A) After that, frequencies of live CD4 T cells producing one, two or three cytokines (IL-2, IFN-γ, and/or TNF-α) were evaluated by intracellular cytokine staining and flow cytometry (n = 13). (B) Frequencies of cytokine producing CD4 T cells in response to each antigen with a naïve (CCR7+ CD45RA+), central memory (CCR7+ CD45RA−), effector memory (CCR7− CD45RA−), and terminal effector memory (CCR7− CD45RA+) are represented (n = 3–5). (Source: Data were taken from publication Parra et al., 2014b.)
2.2. Rotavirus-Specific CD8 T Cells
2.3. Epitopes Recognized by Rotavirus-Specific T Cells
2.4. Markers of Intestinal Homing on Rotavirus-Specific T Cells

RV- and influenza-specific CD4 T cells from eight healthy adults were identified using tetramers coupled with specific peptides and simultaneously stained with antibodies against α4β7 and CCR9. (Source: Data were taken from publication Parra et al., 2014a.)
2.5. Intestinal Human Rotavirus-Specific T Cells
2.6. In Vitro Models to Study Human Rotavirus-Specific T Cells
3. Rotavirus-specific B cells

Summary of the median frequencies of seven subsets of total and RV-specific mBc assessed by multiparametric flow cytometry from 10 healthy adults. Donuts denote the median frequencies of CD19+ B cells (%) subsets. * Statistically significant differences (p < 0.05, two-tailed Wilcoxon test) between total and RV-specific mBc subsets. (Source: Data were taken from publication Herrera et al., 2014.)
4. Correlates of protection
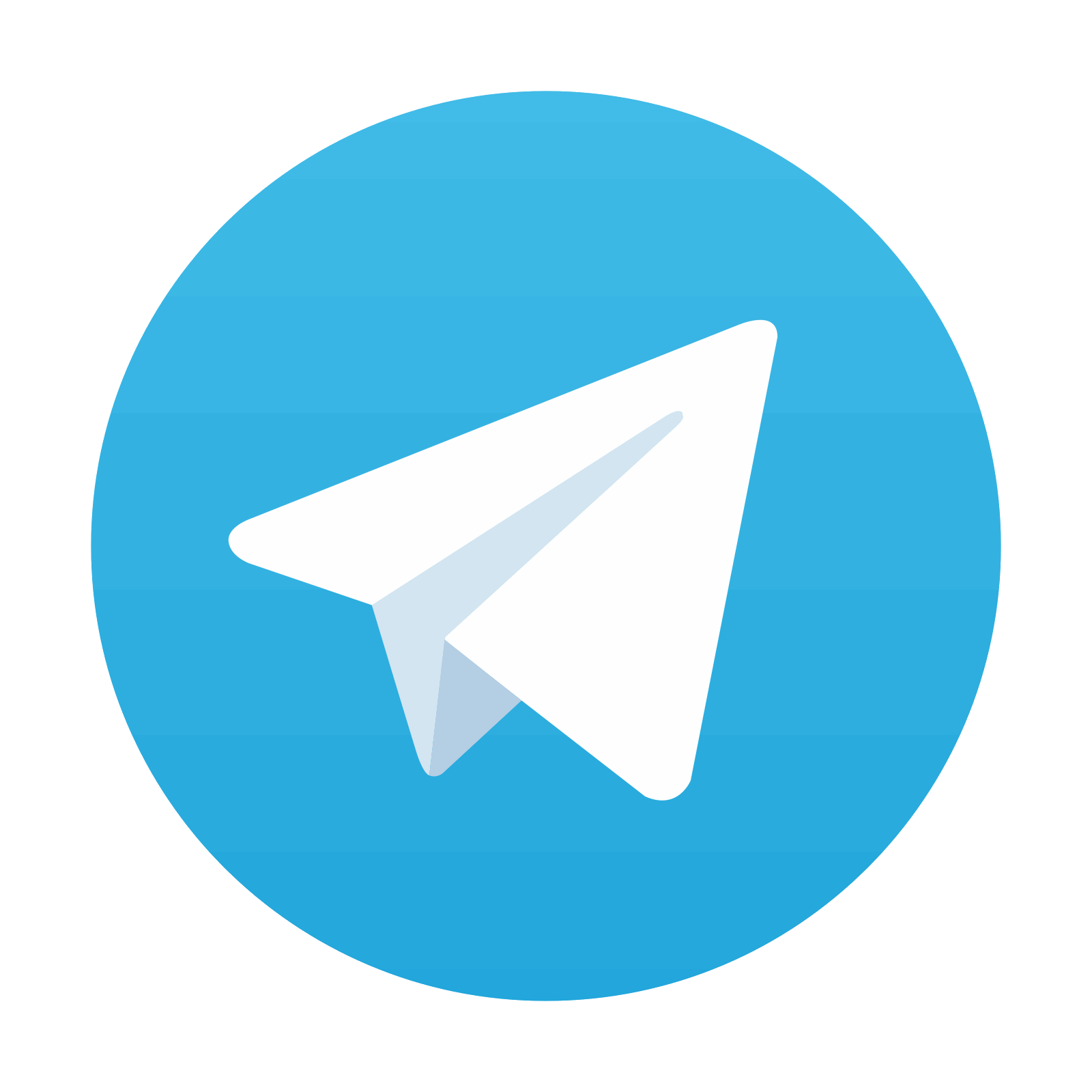
Stay updated, free articles. Join our Telegram channel

Full access? Get Clinical Tree
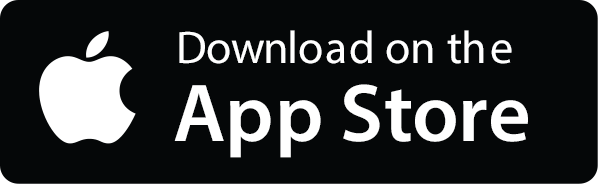
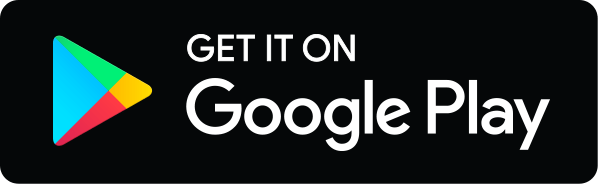