OBJECTIVES
After studying this chapter, you should be able to:
Understand the importance of maintaining homeostasis of body calcium and phosphate concentrations, and how this is accomplished.
Describe the body pools of calcium, their rates of turnover, and the organs that play central roles in regulating movement of calcium between stores.
Delineate the mechanisms of calcium and phosphate absorption and excretion.
Identify the major hormones and other factors that regulate calcium and phosphate homeostasis and their sites of synthesis as well as targets of their action.
Define the basic anatomy of bone.
Delineate cells and their functions in bone formation and resorption.
INTRODUCTION
Calcium is an essential intracellular signaling molecule and also plays a variety of extracellular functions, thus the control of body calcium concentrations is vitally important. The components of the system that maintains calcium homeostasis include cell types that sense changes in extracellular calcium and release calcium-regulating hormones, and the targets of these hormones, including the kidneys, bones, and intestine, that respond with changes in calcium mobilization, excretion, or uptake. Three hormones are primarily concerned with the regulation of calcium homeostasis. Parathyroid hormone (PTH) is secreted by the parathyroid glands. Its main action is to mobilize calcium from bone and increase urinary phosphate excretion. 1,25-Dihydroxycholecalciferol is a steroid hormone formed from vitamin D by successive hydroxylations in the liver and kidneys. Its primary action is to increase calcium absorption from the intestine. Calcitonin, a calcium-lowering hormone that in mammals is secreted primarily by cells in the thyroid gland, inhibits bone resorption. Although the role of calcitonin seems to be relatively minor, all three hormones probably operate in concert to maintain the constancy of the calcium level in the body fluids. Phosphate homeostasis is likewise critical to normal body function, particularly given its inclusion in adenosine triphosphate (ATP), its role as a biologic buffer, and its role as a modifier of proteins, thereby altering their functions. Many of the systems that regulate calcium homeostasis also contribute to that of phosphate, albeit sometimes in a reciprocal manner, and thus will also be discussed in this chapter.
CALCIUM & PHOSPHORUS METABOLISM
The body of a young adult human contains about 1100 g (27.5 moles) of calcium. Ninety-nine percent of the calcium is in the skeleton. Plasma calcium, normally at a concentration of around 10 mg/dL (5 mEq/L, 2.5 mmol/L), is partly bound to protein and partly diffusible (Table 21–1). The distribution of calcium inside the cells and the role of Ca2+ as a second messenger molecule are discussed in Chapter 2.
It is the free, ionized calcium (Ca2+) in the body fluids that is a vital second messenger and is necessary for blood coagulation, muscle contraction, and nerve function. A decrease in extracellular Ca2+ exerts a net excitatory effect on nerve and muscle cells in vivo (see Chapters 4 and 5). The result is hypocalcemic tetany, which is characterized by extensive spasms of skeletal muscle, involving especially the muscles of the extremities and the larynx. Laryngospasm can become so severe that the airway is obstructed and fatal asphyxia is produced. Ca2+ also plays an important role in blood clotting (see Chapter 31), but in vivo, fatal tetany would occur before compromising the clotting reaction.
Because the extent of Ca2+ binding by plasma proteins is proportional to the plasma protein level, it is important to know the plasma protein level when evaluating the total plasma calcium. Other electrolytes and pH also affect the free Ca2+ level. Thus, for example, symptoms of tetany appear at higher total calcium levels if the patient hyperventilates, thereby increasing plasma pH. Plasma proteins are more ionized when the pH is high, providing more protein anions to bind with Ca2+.
The calcium in bone is of two types: a readily exchangeable reservoir and a much larger pool of stable calcium that is only slowly exchangeable. Two independent but interacting homeostatic systems affect the calcium in bone. One is the system that regulates plasma Ca2+, providing for the movement of about 500 mmol of Ca2+ per day into and out of the readily exchangeable pool in the bone (Figure 21–1). The other system involves bone remodeling by the constant interplay of bone resorption and deposition (see following text). However, the Ca2+ interchange between plasma and this stable pool of bone calcium is only about 7.5 mmol/d.
Ca2+ is transported across the brush border of intestinal epithelial cells via channels known as transient receptor potential vanilloid type 6 (TRPV6) and binds to an intracellular protein known as calbindin-D9k. Calbindin-D9k sequesters the absorbed calcium so that it does not disturb epithelial signaling processes that involve calcium. The absorbed Ca2+ is thereby delivered to the basolateral membrane of the epithelial cell, from where it can be transported into the bloodstream by either a Na+/Ca2+ exchanger (NCX1) or a Ca2+-dependent ATPase. Nevertheless, it should be noted that recent studies indicate that some intestinal Ca2+ uptake persists even in the absence of TRPV6 and calbindin-D9k, suggesting that additional pathways are likely also involved in this critical process. The overall transport process is regulated by 1,25-dihydroxycholecalciferol (see below). As Ca2+ uptake rises, moreover, 1,25-dihydroxycholecalciferol levels fall in response to increased plasma Ca2+.
Plasma Ca2+ is filtered in the kidneys, but 98–99% of the filtered Ca2+ is reabsorbed. About 60% of the reabsorption occurs in the proximal tubules and the remainder in the ascending limb of the loop of Henle and the distal tubule. Distal tubular reabsorption depends on the TRPV5 channel, which is related to TRPV6 discussed previously, and whose expression is regulated by PTH.
Phosphate is found in ATP, cyclic adenosine monophosphate (cAMP), 2,3-diphosphoglycerate, many proteins, and other vital compounds in the body. Phosphorylation and dephosphorylation of proteins are involved in the regulation of cell function (see Chapter 2). Therefore, it is not surprising that, like calcium, phosphate metabolism is closely regulated. Total body phosphorus is 500–800 g (16.1–25.8 moles), 85–90% of which is in the skeleton. Total plasma phosphorus is about 12 mg/dL, with two-thirds of this total in organic compounds and the remaining inorganic phosphorus (Pi) mostly in PO43–, HPO42–, and H2PO4–. The amount of phosphorus normally entering bone is about 3 mg (97 μmol)/kg/d, with an equal amount leaving via reabsorption.
Pi in the plasma is filtered in the glomeruli, and 85–90% of the filtered Pi is reabsorbed. Active transport in the proximal tubule accounts for most of the reabsorption and involves two related sodium-dependent Pi cotransporters, NaPi-IIa and NaPi-IIc. NaPi-IIa is powerfully inhibited by PTH, which causes its internalization and degradation and thus a reduction in renal Pi reabsorption (see below).
Pi is absorbed in the duodenum and small intestine. Uptake occurs by a transporter related to those in the kidney, NaPi-IIb, that takes advantage of the low intracellular Na+ concentration established by the Na, K ATPase on the basolateral membrane of intestinal epithelial cells to load Pi against its concentration gradient. However, the pathway by which Pi exits into the bloodstream is not known. Many stimuli that increase Ca2+ absorption, including 1,25-dihydroxycholecalciferol, also increase Pi absorption via increased NaPi-IIb expression and/or its insertion into the enterocyte apical membrane.
VITAMIN D & THE HYDROXYCHOLECALCIFEROLS
The active transport of Ca2+ and PO43− from the intestine is increased by a metabolite of vitamin D. The term “vitamin D” is used to refer to a group of closely related sterols produced by the action of ultraviolet light on certain provitamins (Figure 21–2). Vitamin D3, which is also called cholecalciferol, is produced in the skin of mammals from 7-dehydrocholesterol by the action of sunlight. The reaction involves the rapid formation of previtamin D3, which is then converted more slowly to vitamin D3. Vitamin D3 and its hydroxylated derivatives are transported in the plasma bound to a globulin, vitamin D-binding protein (DBP). Vitamin D3 is also ingested in the diet.
Vitamin D3 is metabolized by enzymes that are members of the cytochrome P450 (CYP) superfamily (see Chapters 1 and 28). In the liver, vitamin D3 is converted to 25-hydroxycholecalciferol (calcidiol, 25-OHD3). The 25-hydroxycholecalciferol is converted in the cells of the proximal tubules of the kidneys to the more active metabolite 1,25-dihydroxycholecalciferol, which is also called calcitriol or 1,25-(OH)2D3. 1,25-Dihydroxycholecalciferol is also made in the placenta, in keratinocytes in the skin, and in macrophages. The normal plasma level of 25-hydroxycholecalciferol is about 30 ng/mL, and that of 1,25-dihydroxycholecalciferol is about 0.03 ng/mL (approximately 100 pmol/L). The less active metabolite 24,25-dihydroxycholecalciferol is also formed in the kidneys (Figure 21–2).
1,25-Dihydroxycholecalciferol stimulates the expression of a number of gene products involved in Ca2+ transport and handling via its receptor, which acts as a transcriptional regulator in its ligand-bound form. One group is the family of calbindin-D proteins. These are members of the troponin C superfamily of Ca2+-binding proteins that also includes calmodulin (see Chapter 2). Calbindin-Ds are found in human intestine, brain, and kidneys. In the intestinal epithelium and many other tissues, two calbindins are induced: calbindin-D9K and calbindin-D28K, with molecular weights of 9000 and 28,000, respectively. 1,25-Dihydroxycholecalciferol also increases the number of Ca2+–ATPase and TRPV6 molecules in the intestinal cells, and thus, the overall capacity for absorption of dietary calcium.
In addition to increasing Ca2+ absorption from the intestine, 1,25-dihydroxycholecalciferol facilitates Ca2+ reabsorption in the kidneys via increased TRPV5 expression in the proximal tubules, increases the synthetic activity of osteoblasts, and is necessary for normal calcification of matrix (Clinical Box 21–1). The stimulation of osteoblasts brings about a secondary increase in the activity of osteoclasts (see below).
The formation of 25-hydroxycholecalciferol does not appear to be stringently regulated. However, the formation of 1,25-dihydroxycholecalciferol in the kidneys, which is catalyzed by the renal 1α-hydroxylase, is regulated in a feedback manner by plasma Ca2+ and PO43+ (Figure 21–3). When the plasma Ca2+ level is high, little 1,25-dihydroxycholecalciferol is produced, and the kidneys produce the relatively inactive metabolite 24,25-dihydroxycholecalciferol instead. This effect of Ca2+ on production of 1,25-dihydroxycholecalciferol is the mechanism that brings about adaptation of Ca2+ absorption from the intestine (see previous text). Conversely, expression of 1α-hydroxylase is stimulated by PTH, and when the plasma Ca2+ level is low, PTH secretion is increased. The production of 1,25-dihydroxycholecalciferol is also increased by low plasma PO43– levels and inhibited by high plasma PO43– levels, by a direct inhibitory effect of PO43– on the 1α-hydroxylase. Additional control of 1,25-dihydroxycholecalciferol formation results from a direct negative feedback effect of the metabolite on 1α-hydroxylase, a positive feedback action on the formation of 24,25-dihydroxycholecalciferol, and a direct action on the parathyroid gland to inhibit PTH expression.
FIGURE 21–3
Effects of PTH and 1,25-dihydroxycholecalciferol on whole body calcium homeostasis. A reduction in plasma calcium stimulates parathyroid hormone secretion. PTH in turn causes calcium conservation and production of 1,25-dihydroxycholecalciferol in the kidneys, the latter of which increases calcium uptake in the intestine. PTH also releases calcium from the readily exchangeable pool in the bone. All of these actions act to restore normal plasma calcium. (Reproduced with permission from Widmaier EP, Raff H, Strang KT: Vander’s Human Physiology, 10th ed. New York, NY: McGraw-Hill; 2006.)
An “anti-aging” protein called α-Klotho (named after Klotho, a daughter of Zeus in Greek mythology who spins the thread of life) has also recently been discovered to play important roles in calcium and phosphate homeostasis, in part by reciprocal effects on 1,25-dihydroxycholecalciferol levels. Mice deficient in α-Klotho displayed accelerated aging, decreased bone mineral density, calcifications, and hypercalcemia and hyperphosphatemia. α-Klotho plays an important role in stabilizing the membrane localization of proteins important in calcium and phosphate (re)absorption, such as TRPV5 and Na, K ATPase. Likewise, it enhances the activity of another factor, fibroblast growth factor 23 (FGF23), at its receptor. FGF23 thereby decreases renal NaPi-IIa and NaPi-IIc expression and inhibits the production of 1α-hydroxylase, reducing levels of 1,25-dihydroxycholecalciferol (Clinical Box 21–1).
CLINICAL BOX 21–1 Rickets & Osteomalacia
Vitamin D deficiency causes defective calcification of bone matrix and the disease called rickets in children and osteomalacia in adults. Even though 1,25-dihydroxycholecalciferol is necessary for normal mineralization of bone matrix, the main defect in this condition is failure to deliver adequate amounts of Ca2+ and PO43– to the sites of mineralization. The full-blown condition in children is characterized by weakness and bowing of weight-bearing bones, dental defects, and hypocalcemia. In adults, the condition is less obvious. It used to be most commonly due to inadequate exposure to the sun in smoggy cities, but now it is more commonly due to inadequate intake of the provitamins on which the sun acts in the skin. These cases respond to administration of vitamin D. The condition can also be caused by inactivating mutations of the gene for renal 1α-hydroxylase, or in severe kidney or liver diseases, in which case there is no response to vitamin D but a normal response to 1,25-dihydroxycholecalciferol (type I vitamin D–resistant rickets). In rare instances, it can be due to inactivating mutations of the gene for the 1,25-dihydroxycholecalciferol receptor (type II vitamin D–resistant rickets), in which case there is a deficient response to both vitamin D and 1,25-dihydroxycholecalciferol.
THERAPEUTIC HIGHLIGHTSTreatment of these conditions depends on the underlying biochemical basis, as indicated above. Routine supplementation of milk with vitamin D has greatly reduced the occurrence of rickets in Western countries, but the condition remains among the most common childhood diseases in developing countries. Orthopedic surgery may be necessary in severely affected children.
THE PARATHYROID GLANDS
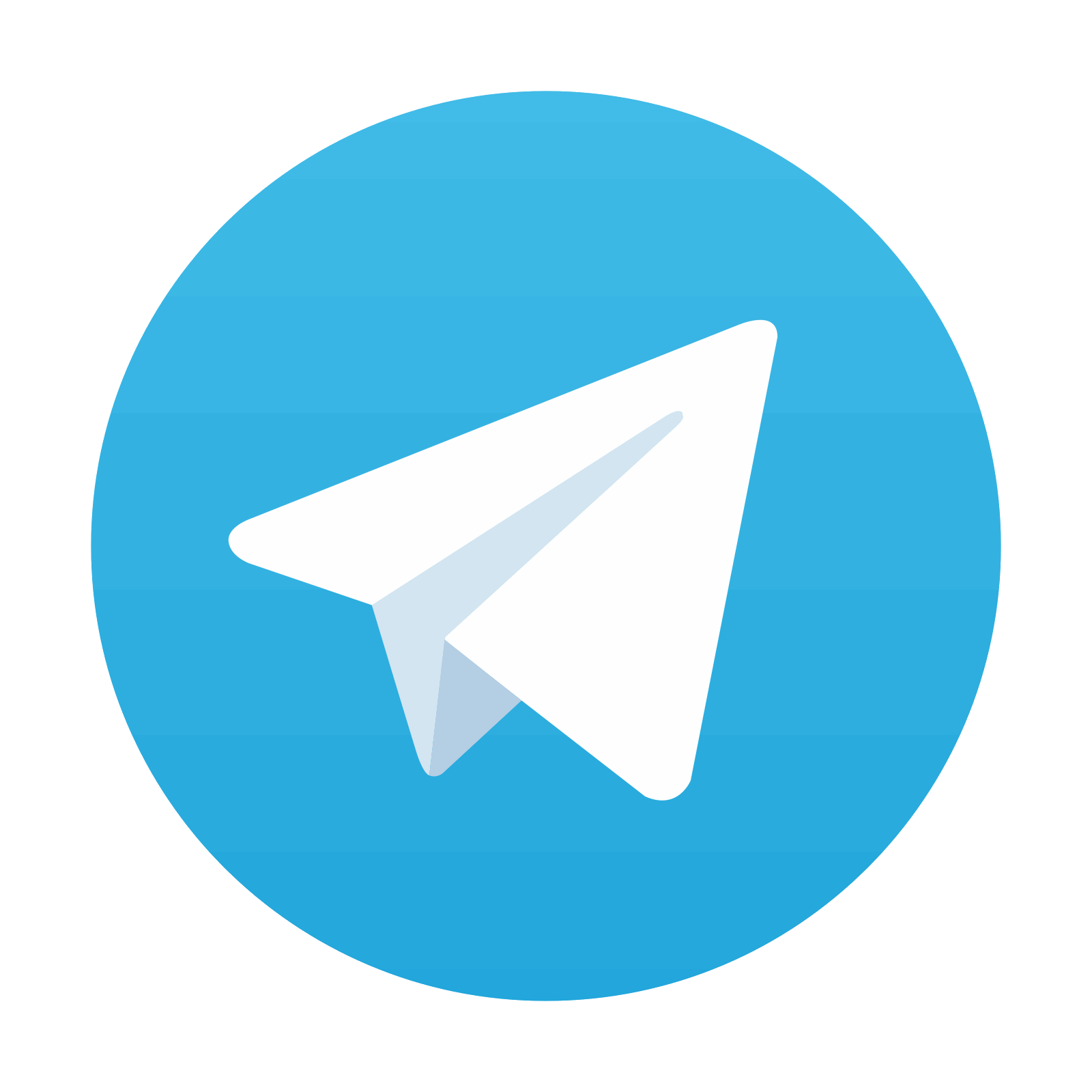
Stay updated, free articles. Join our Telegram channel

Full access? Get Clinical Tree
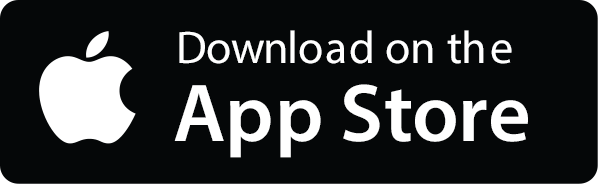
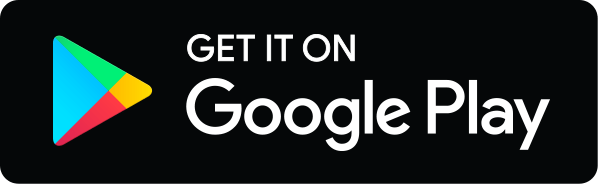