Figure 6-1. Primary hemostasis is achieved initially with a platelet aggregation as illustrated. Note that platelet adhesion, shape change, granule release, followed by recruitment and the hemostatic plug at the area of subendothelial collagen (binds to GpVI) exposure are the initial events for thrombus formation. Platelets can also be activated by PDI with de-encryption of TF.
The intrinsic pathway of blood coagulation requires activation of factor XI to XIa. This may occur by both the contact activation system through activation of factor XII, plasma prekallikrein, and high–molecular-weight kininogen and, more important, through thrombin with negatively charged surfaces.19 Factor XIa activates factor XI autocatalytically and also catalyzes the conversion of factor IX to IXa. After activation, factor VIIIa dissociates from vWF and assembles with factors IXa and X. Factor IXa, factor X, ionized calcium, and thrombin-activated factor VIII (VIIIa) then assemble on the platelet surface in a complex called the Xase complex to catalyze the activation of factor X to Xa. Factor Xa then shunts into the prothrombinase complex for further amplification of thrombin formation.
The importance of a mechanism of factor XI activation independent of the contact activation system is apparent because patients deficient in those factors of the contact activation system, including factor XI, bleed, whereas patients deficient in factor XII, prekallikrein, and high–molecular-weight kininogen do not usually bleed.20 The contact activation system is the most important coagulation process involved in extracorporeal bypass circuits, including cardiopulmonary bypass and extracorporeal membrane oxygenation.
Figure 6-2. The classical pathway showing the interface between the intrinsic pathway, extrinsic pathway, and the common pathway is illustrated with the ultimate production of thrombin. This catalyzes fibrin from fibrinogen, and then cross-linking the fibrin to form a stable clot.
NATURAL ANTICOAGULANT MECHANISMS
1 At the same time that thrombin forms, natural anticoagulant mechanisms oppose further thrombin formation and help to localize thrombin activity to areas of vascular injury. Just as thrombin generation is key to coagulation, antithrombin (AT) is the central anticoagulant protein (Fig. 6-3). This glycoprotein of 70-kD molecular weight binds to thrombin, preventing the removal of FPA and FPB from fibrinogen, prevents the activation of factors V and VIII, and inhibits the activation and aggregation of platelets. In addition, AT directly inhibits factors IXa, Xa, and XIa.
A second natural anticoagulant is activated protein C (APC), which inactivates factors Va21,22 and VIIIa, thus reducing the Xase and prothrombinase complex acceleration of the rate of thrombin formation. In the circulation, protein C is activated on endothelial cell surfaces by thrombin complexed with one of its receptors, thrombomodulin (TM).23–25 The formation of this thrombin–TM complex accelerates the activation of protein C compared with thrombin alone. Thrombin, at the same time, by binding to TM, loses its platelet-activating activity as well as its enzymatic activity for fibrinogen and factor V. Protein S is a cofactor for APC.
Another innate anticoagulant is tissue factor pathway inhibitor (TFPI). The protein binds to TF–VIIa complex, inhibiting the activation of factor X to Xa and the formation of the prothrombinase complex.26 A fourth natural anticoagulant is heparin cofactor II.27 Its concentration in plasma is estimated to be significantly less than that of AT, and its action is implicated primarily in the regulation of thrombin formation in extravascular tissues. Finally, thrombin is inactivated when it becomes incorporated into the clot.
FIBRINOLYSIS
In addition to natural anticoagulants such as protein C and S, physiologic clot formation is balanced by a contained process of clot lysis, which prevents thrombus formation from proceeding outside of the injured area (Fig. 6-4). The central fibrinolytic enzyme is plasmin, a serine protease generated by the proteolytic cleavage of the proenzyme, plasminogen. Its main substrates include fibrin, fibrinogen, and other coagulation factors. Plasminogen, tissue plasminogen activator (tPA), and α2-antiplasmin (α2-AP) become incorporated into the fibrin clot as it forms. Plasminogen activators are serine proteases that activate plasminogen, by cleavage of a single arginine–valine peptide bond, to the enzyme plasmin. Plasminogen activation provides localized proteolytic activity.28–30 In fact, thrombin promotes tPA release from endothelial cells as well as the production of plasminogen activator inhibitor (PAI-1) from endothelial cells.31,32
Figure 6-3. Antithrombin is a primary anticoagulant. Note antithrombin complexes with IIa to inhibit fibrin polymerization, as well as factor Xa, and an inactivating factor Va and VIIIa.
Figure 6-4. Hemostasis with thrombus production is a tight and intricate process that is locally confined. Balancing thrombus production is tissue plasmin activator and urokinase plasminogen activator which activates plasmin and causes thrombolysis. These are balanced by plasminogen activator inhibitor-1 and alpha-2-antiplasmin. Free plasmin is complexed rapidly. Fibrin degradation products, such as D-dimer are produced.
The major endogenous plasminogen activators include tPA and urokinase, and intrinsic factors, such as factor XII, prekallikrein, and high–molecular-weight kininogen. These later factors of the contact system are more important in clot lysis than thrombus formation. These enzymes may also liberate bradykinin from high–molecular-weight kininogen, resulting in an increase in vascular permeability, prostacyclin (PGI2) liberation, and tPA secretion. Finally, APC has been found to proteolytically inactivate the inhibitor to tPA, thus promoting tPA activity and fibrinolysis.33
Fibrin, when digested by plasmin, yields one molecule of fragment E and two molecules of fragment D. In physiologic clot formation, fragment D is released in dimeric form (D-dimer),20,34 and is a marker for fibrinolysis of formed clot. An elevated D-dimer level after treatment of DVT is one biomarker that has been found to accurately predict an ongoing risk of recurrent VTE.35
Two primary inhibitors of plasmin are important. First, α2-AP is released by endothelial cells and complexes with plasmin. In physiologic fibrinolysis, α2-AP is bound to fibrin and excess plasmin is readily inactivated. In plasma, PAI-1 is the primary inhibitor of plasminogen activators. It is secreted in an active form from liver and endothelial cells and stabilized by binding to Vn. PAI-1 levels are elevated by hyperlipidemia, and PAI-1 elevation appears to synergize with factor V Leiden genetic abnormalities.36
In summary, coagulation is an ongoing process of thrombus formation, inhibition of thrombus formation, and thrombus dissolution. The central mediators are TF, platelets, thrombin, and plasmin. Abnormalities in coagulation occur when one process – thrombus formation, thrombus inhibition, or fibrinolysis – overcomes the others and dominates the delicate balance.
ENDOTHELIUM AND HEMOSTASIS
2 Through its ability to express procoagulants and anticoagulant factors, vasoconstrictors and vasodilators, as well as key cell adhesion molecules and cytokines, the endothelial cell is a key regulator of hemostasis (Fig. 6-5).37 Vascular endothelium maintains a vasodilatory and local fibrinolytic state in which coagulation, platelet adhesion and activation, and leukocyte activation are suppressed.
Vasodilatory endothelial products include adenosine, nitric oxide (NO), and PGI2. A nonthrombogenic endothelial surface is maintained by four main mechanisms including; endothelial production of TM and subsequent activation of protein C, endothelial expression of surface heparan- and dermatan sulfate, constitutive expression of TFPI by endothelium (which is markedly accelerated in response to heparin), and local production of tPA and urokinase plasminogen activator (uPA).37,38 Finally, the elaboration of NO and interleukin (IL)-10 by endothelium inhibits leukocyte adhesion and activation.
During states of endothelial disturbances such as injury, a prothrombotic and proinflammatory state of vasoconstriction is driven by the endothelial surface. Endothelial release of platelet-activating factor (PAF) and endothelin-1 promotes vasoconstriction.38 Endothelial cells increase production of vWF, TF, PAI-1, and factor V to augment thrombosis with exposure to prothrombotic stimuli. Finally, in response to endothelial injury, endothelial cells are activated, resulting in increased surface expression of cell adhesion molecules, promoting leukocyte adhesion and activation.
THROMBOSIS, INFLAMMATION, AND RESOLUTION
3 After VT, an acute to chronic inflammatory response occurs in the vein wall and thrombus progressing from thrombus amplification, to organization, and vein recanalization (often at the expense of vein wall fibrosis and vein valvular damage). Initially, there is an increase in neutrophils (PMN) in the vein wall followed by monocytes/macrophages. Cytokines, chemokines, and inflammatory factors (e.g., tumor necrosis factor [TNF]) facilitate inflammation. The ultimate response of the vein wall depends on proinflammatory and anti-inflammatory mediator balance at the interface between the leukocyte, activated platelet, and endothelium.39
Cell Adhesion Molecules
Selectins (P- and E-selectin) have been found to be intimately involved in this process (Fig. 6-6).40 Selectins are the first upregulated glycoproteins on activated platelets (alpha granules) and endothelial cells (Weibel–Palade bodies). They mediate the adhesion of leukocytes, platelets, and even cancer cells in inflammation and thrombosis. The P-selectin receptor is P-selectin glycoprotein ligand-1 (PSGL-1). P-selectin:PSGL-1 interactions trigger the release of procoagulant microparticles (MPs), that support fibrin formation, thrombus growth, and increase monocyte TF expression.
MPs <1 micron fragments may be central for P-selectin’s effect. It is believed that with initial thrombosis, P-selectin upregulation leads to MP formation. These procoagulant MPs, which may express TF, are then recruited into the area of developing thrombosis, amplifying the process. Consistently, P-selectin gene deficiency, results in less MP formation, and less thrombosis.41 In an experimental study, the generation of procoagulant activity was shown to be dependent on P-selectin:PSGL-1 interactions related to MP formation.42 The procoagulant nature of these MP was demonstrated by their ability to normalize bleeding in factor VIII–deficient mice.
Figure 6-5. The endothelium is a primary interface allowing both anticoagulant functions in the resting state, with prostacyclin, NO, plasminogen activators, and thrombomodulin. Procoagulant proteins are expressed on activated endothelium including selectins, procoagulant proteins, such as the von Willebrand factor, TF, as well as PAI-1.
E-selectin, upregulated after P-selectin, is an important regulator of thrombus formation and fibrin content in a mouse VT model.43,44 Endotoxin-induced TF-mediated coagulation is enhanced in humans carrying the S128R E-selectin allele,45 and patients homozygous for the S128R E-selectin allele have an increased risk for VTE recurrence, highlighting the importance of E-selectin in DVT.46 E-selectin has been shown to be efficient at raising the affinity and avidity of 2 (CD18) integrins which support neutrophil trafficking to sites of acute inflammation and recruit platelets and red blood cells.47
PSGL-1 has greatest affinity for P-selectin, and lesser affinity for E-selectin and L-selectin. The role of P-selectin in VT has been suggested by the study of a mouse with high fourfold higher circulating levels of P-selectin than wild type.48 These mice are hypercoagulable based on clotting tests, and a receptor antagonist against the P-selectin receptor (rPSGL-Ig) reverses the hypercoagulability. Consistently, wild-type mice administered soluble P-selectin (sP-sel) become hypercoagulable. In models of VT, P-selectin inhibition given prophylactically decreases thrombosis in a dose-dependent fashion, and can treat established VT as effectively as heparin without anticoagulation.49,50
Figure 6-6. The interaction between stasis injury and procoagulant syndromes are represented by Virchow triad. Endothelial and vascular injuries cause leukocytes and platelets to express P- and E-selectin and the PSGL-1 receptor. Microparticles are released which express TF. This stimulates the coagulation pathway, fibrin production, and thrombus amplification.
sP-sel is released from activated platelets and endothelial cells and levels rise significantly during pathologic conditions.51–53 sP-sel has been studied as a biomarker for DVT,51,54–56 combining Sp-sel with Wells score (clinical pretest probability of DVT) establishes the diagnosis of DVT. A sP-sel (≥90 ng/mL) combined with Wells score (≥2), showed a better PPV (91%) than D-dimer (≥500 ng/mL) combined with Wells score (≥2), which was 69%.57
Other factors of importance to venous thrombogenesis include vWF, a factor that stabilizes procoagulant factor VIII to promote the initiation and formation of stable thrombus at the site of vascular inflammation and TF. Using a ferric chloride model of vein wall injury, it has been shown that occlusive thrombus formation is dependent on vWF.58 Regarding TF, mouse models using gene-targeting and bone marrow transplantation technologies found that TF in the vessel wall is more imporant than TF from leukocytes for thrombus formation when there is no flow in the experimental model.59 TF has been linked to circulating procoagulant MPs, particularly in cancer patients, cancer is associated with high levels of DVT, and TF activity is increased in cells treated with chemotherapeutic agents.60 In addition, cancer patients with VTE were found to have elevated levels of microparticle TF compared to cancer patients without VTE.61,62
Leukocytes and Thrombosis
Inflammatory cells are important to the process of thrombus recanalization and organization (Fig. 6-7).40,63 Thrombus resolution resembles wound healing, and involves both profibrotic growth factors, collagen deposition, matrix metalloproteinase (MMP) expression, and activation.64,65 As the thrombus resolves, a number of proinflammatory factors are released into the local environment, including interleukin-1 beta (IL-1β) and tumor necrosis factor-alpha (TNF-α).66 The cellular sources of these different mediators are probably leukocytes and smooth muscle–like cells within the resolving thrombus and adjacent vein wall. The fact that leukocytes invade the thrombus in a specific sequence suggests their importance in the normal thrombus resolution.66
The first cell type in the thrombus is the PMN. Although PMNs may cause vein wall injury, they are essential for early thrombus resolution by promoting both fibrinolysis as well as collagenolysis.67,68 In a rat model of stasis VT, neutropenia was associated with larger thrombi at 2 and 7 days, and was correlated with increased thrombus fibrosis and significantly lower thrombus levels of both uPA and MMP-9.69 Conversely, in a nonstasis VT model, PMNs may promote thrombosis.14 It is likely the timing of PMN influx and the amount of perithrombus blood flow that determines prothrombotic or prothrombolytic activities. Through processes that also involve the initial activation of leukocytes and platelets, PMNs form neutrophil endothelial traps (NETs), which are extracellular fragments of DNA containing histones and antimicrobial proteins.14,70 NETs provide a scaffold for thrombus formation and are prothrombotic.71
In order to investigate if plasma DNA is a surrogate for NETs in patients with DVT, and to determine correlations with other biomarkers of DVT studied in our laboratory, patients presenting to our diagnostic vascular laboratory were investigated.72 Patients were divided into positive for DVT by ultrasound, leg pain but negative for DVT by ultrasound, and control volunteers. Circulating DNA was significantly elevated in DVT patients, compared with controls. Of interest was a significant positive correlation of circulating DNA with C-reactive protein, D-dimer, vWF, and the Wells score (all p <0.01).
The monocyte/macrophage is likely the most important cell for later DVT resolution.73 Monocyte influx into the thrombus peaks at day 8 after thrombogenesis, and correlates with elevated MCP-1 levels, which has been associated with DVT resolution.74 Targeted deletion of CC receptor-2 (CCR-2 KO) in the mouse model of stasis thrombosis was associated with late impairment of thrombus resolution, probably via impaired MMP-2 and MMP-9 activities.75 Indeed, precedent exists in humans that the monocytic activation state may predict long-term DVT resolution.76
Thrombi have been found to contain increasing amounts of both tPA and uPA activities as they resolve, and this activity is expressed by invading monocytes.77,78 In a recent study, mice genetically deleted for uPA had impaired thrombus resolution with reduced monocyte infiltration at the margins of the thrombus, with few neovascular channels present.79 Mice gene deleted for tPA, however, were not similarly affected, suggesting that uPA, not tPA, is responsible for this activity.
Figure 6-7. The proposed resolution mechanism involves both early thrombolysis with a large distending clot and then, over time, a fibrotic thrombus that resembles scar tissue as produced. Note proinflammatory factors, as well as neutrophils (releasing NETs), platelets, and MMP are present early with subsequent vein wall injury related to collagenolysis and elastinolysis. Later, vascular smooth muscle cell proliferation appears to occur, with thrombus resolution with increased profibrotic growth factor such as IL-13 and TGF-β. This promotes collagen accumulation. Both PAI-1 and CCR7 are protective against fibrosis.
The local venous environment is by definition hypoxic, hypoxia-inducible factor-1 alpha (HIF-1α). A major angiogenic growth factor is HIF-1α. Experimental data in a stasis model of VT suggest that thrombosis stimulates increased vein wall HIF-1α, and that by exogenous stimulation of HIF-1α expression, thrombus recanalization was increased and associated with accelerated VT resolution.80 Thus, thrombus resolution is in part dependent on neovascularization. The effect of mechanical stretch, such as by a VT, may also stimulate HIF-1α as well as MMP-2 and MMP-9, both leading to reduced vein contractility.81
Recent data linking inflammation to fibrosis demonstrate that inhibition of the inflammatory response can decrease vein wall fibrosis. In a rat model of stasis DVT, treated with either low–molecular-weight heparin (LMWH) or an oral inhibitor to P-selectin 2 days after establishment of thrombosis, inhibition of P-selectin significantly decreased vein wall injury (independent of thrombus size).82 The mechanism accounting for this protective effect is not yet known, but probably does not involve leukocyte blockade, because no differences in influx of monocytes into the vein wall were observed.
Loss of venous endothelium likely also contributes to the vein wall fibrosis, as well as the predisposition to recurrent thrombosis. An experimental model of DVT showed lower expression of homeostatic endothelial genes such as NO and TM than in controls, which correlated with loss of vWF positive cell luminal staining.83 Other investigators have found that prolonged venous stasis is associated with decreased plasminogen activators, probably related to loss of endothelium.84
Associated with the early biomechanical injury from DVT is an elevation of profibrotic mediators, including transforming growth factor-beta (TGF-b), interleukin-13 (IL-13), IL-6, and monocyte chemotactic protein-1 (MCP-1).82,85,86 These mediators are present within the vein wall and thrombus and may drive the fibrotic response. Although exogenous MCP-1 may hasten DVT resolution, it promotes organ fibrosis in vivo. The profibrotic growth factor TGF-b is also present in the thrombus and is activated with normal thrombolysis.87 TGF-b may be a key mechanism promoting vein wall fibrosis.
Late fibrosis has been observed in our mouse model of DVT, which demonstrated a significant increase in vein wall collagen after stasis thrombogenesis.88–90 Based on experimental studies, elastinolysis and collagenolysis seem to occur early, as measured by an increase in vein wall stiffness, persisting through 14 days, and are accompanied by elevated MMP-2 and MMP-9 activities.85,89 Correlating with this increase in fibrosis is altered procollagen I and III gene expression, as well as an increase in MMP-2 and MMP-9 gene expression and activity. Genetic deletion of MMP-2 or MMP-2/9 is associated with decreased midterm vein wall fibrosis, possibly by modulating vein wall elastin/collagen metabolism as well as monocyte influx.64,65 It has been demonstrated that decreasing inflammation with a selectin inhibitor or with neutralizing IL-6 can also decrease vein wall fibrosis.43,91,92 Moreover, the thrombus size itself does not drive the vein wall injury response; rather the mechanism of thrombosis seems more important.85,93 Lastly, PAI-1 overexpression is associated with decreased vein wall fibrosis, in part by decreased MMP-2/9 activity.90
ARTERIAL VERSUS VENOUS THROMBOSIS
Thrombosis in the arterial system occurs somewhat differently than in the venous system (Fig. 6-8). The elements required for the initiation of DVT were described by Virchow as stasis, endothelial injury, and hypercoagulability of the blood. In the arterial circulation, endothelial vascular injury (whether acute or chronic) is key to thrombosis. This is most clearly demonstrated by the typical atherosclerotic plaque. In advanced lesions, the lipid core of the plaque is rich in inflammatory cells, cholesterol crystals, and TF (generated by activated macrophages within the plaque).94 Plaque ulceration exposes highly thrombogenic lipid to the bloodstream, activating coagulation, platelet aggregation, and leading to the deposition of clot.95 Platelet deposition occurs at the apex of stenosis, the point of maximal shear force. The blood flow velocity itself regulates how quickly both thrombi form and dissolve.96
A platelet-rich thrombus is observed in arterial thrombus while in contrast, venous blood stasis and changes in its composition (leading to hypercoagulability) incite the formation of thrombus from local procoagulant events, including small endothelial disruptions at venous confluences, saccules, and valve pockets.11 Hypercoagulable states have classically been highly associated with VTE, but do play a role in cardiovascular disease as well.97,98 Moreover, HMG-CoA reductase inhibitors (statin) not only decrease atherothrombotic events, but also incident VTE,99 suggesting some common pathogenic pathways.
PROCOAGULANT STATES
Acquired Procoagulant States
4 Most thrombotic clinical episodes have an identifiable cause, although environment risks and genetic predispositions to thrombosis may account for many of the VTE that manifest clinically.11 Risk factors for arterial thrombosis are primarily related to atherosclerosis, and are detailed elsewhere.
The most common risk factors for VTE are prior DVT, malignancy, immobility, intravenous catheters, increased age, major surgery, trauma, infections such as pneumonia and urinary tract infection, and certain chemotherapies (Table 6-1).100–102 Certain medications such as oral contraceptives and hormonal replacement therapies also increase the risk of VTE.
Of primary importance to surgeons is how best to estimate perioperative VTE risk, and apply appropriate prophylaxis. This can be done with a screening form, such as that devised by Caprini et al.103 and Pannucci et al.104 Essentially, this is a focused assessment of VTE risks related to current illnesses and history that may not be fully covered in the routine history and physical. The recommendations are also well detailed in the routinely updated American College of Chest Physicians VTE evidence-based guidelines.105 The higher the risk, the more intensive the prophylaxis, is the paradigm. For example, an outpatient hernia patient may require no prophylaxis outside of early ambulation where as a hip replacement in an obese patient would be best treated with anticoagulation as well as sequential compression devices for the lower extremities. However, all patients should be assessed for VTE risk.
Malignancy
Cancer represents an independent risk factor for the development of VTE, with a sixfold increased risk in cancer patients. Importantly, cancer patients undergoing surgical procedures have twice the risk of development of postoperative DVT.106 Highest rates of VTE are seen in pancreas, stomach, and lung cancer. The malignancy itself activates a procoagulant phenotype by expression of TF by malignant cells, release of TF-bearing MPs, and increased activation of neutrophil extracellular traps – the downstream effects of which have been outlined above.107
Despite considerable evidence supporting the use of prophylactic anticoagulation in cancer patients, many do not receive optimal pharmacoprophylaxis for DVT while inpatient.108 An extended duration of therapy – 28 days – was found to be associated with a decreased incidence of DVT at the end of the study period; consequently, current guidelines recommend extended thromboprophylaxis in cancer patients after an operative intervention.108
Figure 6-8. Depicted in A is the typical atherosclerotic thrombotic nidus, which includes rupture of a plaque, composed of smooth muscle, foam cells, and leukocytes. Platelets are the primary intermediary in arterial thrombosis, as well as TF. In figure B, increased coagulability, as well as vessel wall changes with procoagulant TF expression promotes thrombosis.
Inflammatory Bowel Disease
Since the first half of the last century, a link between inflammatory bowel disease (IBD) and VTE has been recognized. While there does appear some correlation between thrombosis and the inflammatory nature of the disease, the link between the inflammatory and coagulation cascades does not tell the whole story. There are several possible mechanisms for this increased tendency toward thromboembolic events under active investigation, including spontaneous platelet activation through the CD40–CD40L pathway.109
A recent retrospective cohort study of the National Surgical Quality Improvement Program database demonstrated that the presence of IBD is an independent risk factor for postoperative VTE with an odds ratio of 2.110 Interestingly, there appears to be an increased tendency to form clot in unusual locations, such as cerebral and hepatic veins. Currently, guidelines recommend only standard pharmacologic thromboprophylaxis for patients with IBD.
Trauma
As with other acquired prothrombotic states, the systemic inflammatory milieu is associated with VTE in trauma patients. Activation of inflammatory pathways through TNF-α with increased circulating TF and procoagulant MP induces a prothrombotic state.111 Incidence of VTE in trauma patients is around 2%, and this may be higher in patients with other risk factors, such as old age, obesity, and prolonged immobility after bone fractures.112,113 LMWH with application of pneumatic compression devices has been shown to be the preferred thromboprophylaxis in trauma patients, and this is reflected in anticoagulation guidelines.105 However, optimal dosing regiments are not well defined.
Table 6-1 Acquired Hypercoagulable States
Lupus Anticoagulant/Antiphospholipid Syndrome (Antiphospholipid Antibody)
Antiphospholipid antibody syndrome is a particularly virulent hypercoagulable state that results in both arterial thrombosis and VT and consists of an elevated antiphospholipid antibody titer in association with thrombosis, recurrent fetal loss, thrombocytopenia, and livedo reticularis. Strokes, myocardial infarction, visceral infarction, and extremity gangrene may also occur. Although the lupus anticoagulant has been noted often in patients with systemic lupus erythematosus (SLE), it does occur in patients without SLE. It may also be induced in patients by medications, cancer, and certain infections.114
A number of possible thrombotic mechanisms have been suggested, including inhibition of PGI2 synthesis or release from endothelial cells,115 inhibition of APC by thrombin/TM,116 elevated PAI-1 levels,117 platelet activation,118 endothelial cell activation,119 and interference with the endothelial cell–associated annexin V anticoagulant activity.120 Increased TF expression on monocytes and low free protein S plasma levels have also been found with the antiphospholipid syndrome and a history of thrombosis.121,122
At least one-third of patients with lupus anticoagulants have a history of one or more thrombotic events, 70% or more being VTE.123 Graft thrombosis has been observed in 27% to 50% of patients positive for antiphospholipid antibody.124,125
For the diagnosis of antiphospholipid syndrome, both clinical and laboratory criteria are necessary.
For the clinical criteria, ≥1 must be present:
1. ≥1 clinical episode of, objectively confirmed, arterial, venous, or small vessel thrombosis.
2. Pregnancy morbidity: ≥1 unexplained fetal death @ ≥10 weeks EGA; ≥1 premature birth (≤34th week of gestation) due to eclampsia, severe preeclampsia, or placental insufficiency; ≥3 unexplained consecutive spontaneous abortions @ <10 weeks EGA.126
For the laboratory criteria, ≥1 must be present:
1. LA (+) ≥2 occasions, at least 12 weeks apart, according to ISTH guidelines (prolonged PL-based clotting assay, lack of correction with 1:1 mix, and correction with excess PL).
2. ACLA and/or anti-β2 glycoprotein-I antibody (medium or high immunoglobulin G (IgG) and/or IgM isotype titer ≥2 occasions, at least 12 weeks apart using standardized ELISA assays).
The prolongation in the aPTT is strictly a laboratory phenomenon. The dilute Russell viper venom time confirms the presence of a lupus anticoagulant.
There is imperfect agreement between diagnostic tests for this abnormality. Approximately 80% of patients with a prolonged aPTT will have a positive antiphospholipid antibody, but only 10% to 50% of patients with a positive antiphospholipid antibody will have a prolonged aPTT.127
Heparin followed by warfarin has been recommended for the treatment of the antiphospholipid syndrome.114,123 For recurrent fetal loss, heparin or LMWH use through pregnancy is recommended. In patients with lupus anticoagulants, heparin is monitored by antifactor Xa levels.
Heparin-Induced Thrombocytopenia and Thrombosis Syndrome
5 Heparin-induced thrombocytopenia (HIT) occurs in 0.6% to 30% of patients in whom heparin is given, although severe thrombocytopenia associated with thrombosis (HITTS) is seen much less frequently.128,129 Approximately one half of HIT patients have thrombosis that is noted clinically or by duplex imaging. In an analysis of 11 prospective studies, the incidence was reported to be 3%, with thrombosis in 0.9%.130 Although earlier morbidity and mortality rates of 61% and 23% had been reported,116 with early diagnosis and appropriate treatment, these rates have declined to 6% and 0%, respectively.131 HIT is caused by a heparin-dependent IgG antibody that, when bound to platelet factor 4 (PF4), induces platelet aggregation in part by inducing MP formation.132,133 The antibody may not be heparin specific, as the degree of sulfonation of the heparin-like compound has been suggested to be critical for this aggregation.134
Both porcine and bovine UFH as well as LMWH have been associated with HIT.135 The syndrome usually begins 3 to 14 days after heparin is begun. Both arterial and venous thromboses have been reported, and even small exposures to heparin (heparin coating on catheters) can cause the syndrome.128,136 VTE associated with HIT is <1% for LMWH, while it is approximately 12% to 13% for UFH with a higher risk for mortality.137
The diagnosis should be suspected when a patient experiences a 50% or greater decline in platelet count, when there is a fall in platelet count below 100,000/mL during heparin therapy, or in any patient who experiences thrombosis during heparin administration.138 The syndrome may be difficult to diagnose as many hospitalized patients have multiple reasons for low platelet counts, and vigilance is important. A platelet count should be checked about every 2 days when on heparin therapy.
The laboratory diagnosis of HIT/HITTS is made by a number of assays. The serotonin release assay was the “gold standard” in the past. An ELISA test detecting the antiheparin antibody in the patient’s plasma directed against the heparin–PF4 complex is now commonly used.128 This assay is less specific but more sensitive and is easier to perform and interpret than the serotonin assay. However, inappropriate testing is common when HIT is suspected. The 4Ts score is a validated measure of pretest probability with a high negative predictive value that, used appropriately, can limit expensive over-testing and unnecessarily subjecting patients to cessation of heparin and alternative anticoagulation.139,140
When the diagnosis is made (clinically), cessation of heparin is mandatory. This includes removing heparin from intravenous catheters and flushes.136 Warfarin should not be administered until an adequate alternative anticoagulant has been started to prevent thrombotic complications. A number of anticoagulants are now available to substitute for patients with this diagnosis. The direct thrombin inhibitor, argatroban, is FDA approved for this indication and shows no cross-reactivity to heparin antibodies.138,141 When using argatroban, it is important to keep in mind that the INR is artificially elevated with this agent. Fondaparinux has also been used for this indication.142
Hypercoagulability Testing: Clinical signs of possible thrombophilia include thrombosis at a young age (<40), unprovoked thrombosis, recurrent thrombosis, thrombosis at unusual locations such as the mesentery, CNS venous sinus, and portal vein, a family history of thrombosis, particularly unprovoked, severe, and in those <50 years of age.143 Testing for hereditary defects in patients with thrombosis with no family history has pros and cons. On the pro side, testing may: improve understanding of pathogenesis of thrombosis, identify and counsel affected family members, and obviate expensive diagnostic testing (e.g., CT scans) looking for a malignancy. On the con side, testing may identify patients with defects whose management would change, with the potential for overaggressive management, insurance implications for the patient arise, and the testing is rather costly.
In order to determine the efficacy of hypercoagulable testing, a multicenter international observational registry on clinical characteristics, treatment patterns, and outcome in consecutive patients with symptomatic, objectively confirmed acute VTE, was done. In this study, 22,847 patients were enrolled and 4,503 tested for thrombophilia. Of the total patient population, 8.4% had with factor V Leiden, 6.8% had PTG20210A, and 3% had activated protein C resistance (APC-R). The authors concluded that for the low incidence and in accordance with the recommendations made by other authors and international bodies, “the undertaking of thrombophilia testing on patients with a first episode of VTE is not advisable.”144
A hypercoagulable screen should include routine coagulation tests such as the aPTT and platelet count, AT activity and antigen assay, protein C antigen and activity levels, protein S antigen level, and mixing studies to identify a lupus anticoagulant (if indicated); APC-R assay and factor V Leiden gene analysis; prothrombin G20210A genetic analysis; homocysteine level; an antiphospholipid antibody screen that includes anticardiolipin antibody; fibrinogen level; FVIII, FIX, and FXI levels and a functional plasminogen assay.
Inherited Procoagulant States
Defects with High Risk for Thrombosis
Antithrombin Deficiency. AT is a serine protease inhibitor (SERPIN) of thrombin, kallikrein, and factors Xa, IXa, VIIa, XIIa, and XIa (Table 6-2). It is synthesized in the liver and has a half-life of 2.8 days. AT deficiency, accounts for approximately 0.5% to 3% of episodes of VTE, the prevalence in the population is 0.2%, and it carries a 20× increased risk for VTE when heterozygous145–147 and may occur at unusual sites such as mesenteric or cerebral veins. Arterial and graft thromboses have also been described in AT deficiency.148,149 It is inherited in an autosomal dominant fashion most cases become apparent by 50 years of age.150 Homozygous patients usually die in utero, whereas heterozygous patients usually demonstrate AT levels <70% of normal. Acquired AT deficiency results from liver disease, malignancy, sepsis, disseminated intravascular coagulation (DIC), malnutrition, and renal disease.148 AT deficiency is divided into type I deficiency (quantitative) and type II deficiency (qualitative).
Table 6-2 Severity and Frequency of VTE due to Hypercoagulable States
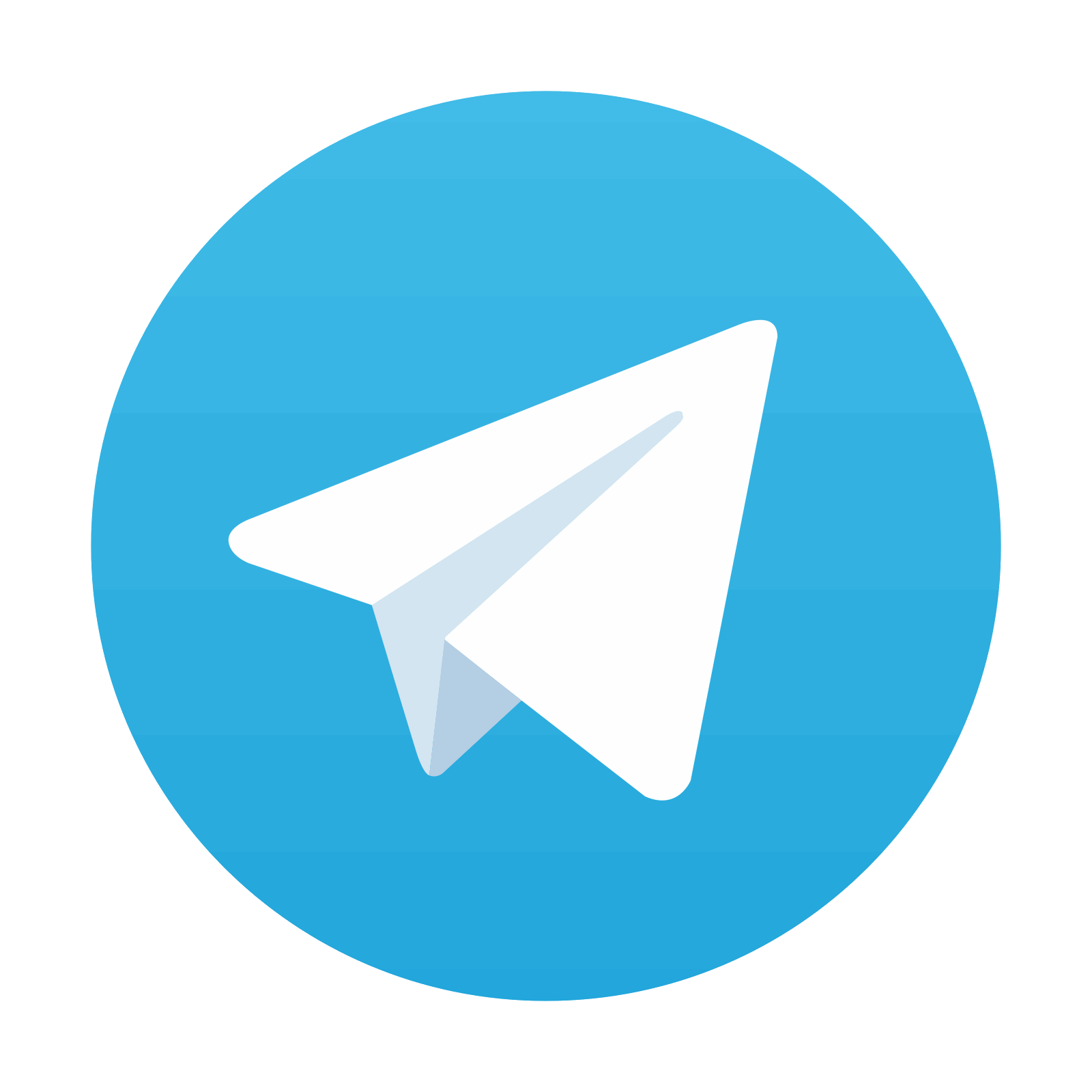
Stay updated, free articles. Join our Telegram channel

Full access? Get Clinical Tree
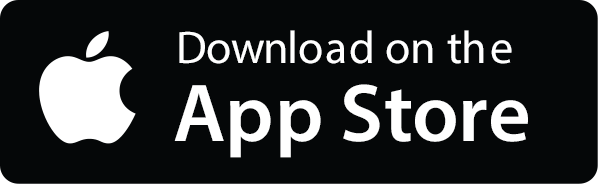
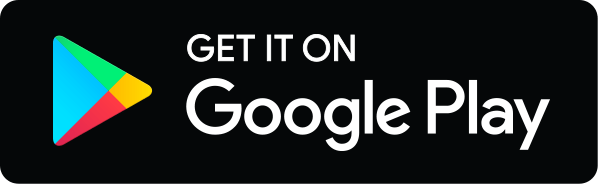