25
Hematopoietic Agents
This chapter will be most useful after having a basic understanding of the material in Chapter 37, Hematopoietic Agents: Growth Factors, Minerals, and Vitamins in Goodman & Gilman’s The Pharmacological Basis of Therapeutics, 12th Edition. In addition to the material presented here, the 12th Edition contains:
• A description of hematopoietic cell growth and differentiation and the role of hematopoietic growth factors in these processes
• A discussion of iron metabolism and storage in the body
• A discussion of factors that cause iron deficiency anemia
• A discussion of vitamin deficiencies that can lead to anemia
• Table 37-3 Iron Requirements for Pregnancy
• Table 37-2 The Body Content of Iron
• Table 37-5 Average Response to Oral Iron
• The molecular structures of vitamin B12 and folic acid, and their congeners
LEARNING OBJECTIVES
Understand the mechanisms of action of drugs, minerals, and vitamins used to stimulate hematopoiesis.
Know the untoward effects of drugs used to stimulate hematopoiesis.
Know the clinical application of hematopoietic growth factors and how efficacy of therapy is monitored.
Know how mineral and vitamin deficiencies can lead to specific anemia, how these are diagnosed, and how they are treated with individual agents or combinations of agents.
Know when iron and vitamin supplementation should be used prophylactically.
DRUGS INCLUDED IN THIS CHAPTER
• Copper sulfate (cupric sulfate)
• Cyanocobalamin (vitamin B12; CALOMIST, NASCOBAL)
• Darbepoetin alfa (ARANESP)
• Eltrombopag (PROMACTA)
• Epoetin alfa (recombinant erythropoietin; EPOGEN, PROCRIT, EPREX)
• Ferrous fumarate (HEMCYTE, FEOSTAT, others)
• Ferrous gluconate (FERGON, others)
• Ferrous succinate, aspartate, and other ferrous salts
• Ferrous sulfate (FEOSOL, others)
• Ferumoxytol (FERAHEME)
• Folic acid (pteroylglutamic acid [PteGlu], L-methylfolate)
• Folinic acid (leucovorin calcium, citrovorum factor)
• G-CSF (recombinant G-CSF, filgrastim; NEUPOGEN)
• GM-CSF (recombinant GM-CSF, sargramostim; LEUKINE)
• Hydroxocobalamin (vitamin B12)
• IL-11 (recombinant IL-11; oprelvekin; [NEUMEGA]
• Iron dextran (DEXFERRUM, INFED, IMFERON)
• Iron sucrose (VENOFER)
• Pegylated recombinant G-CSF (pegfilgrastim; NEULASTA)
• Polysaccharide-ferrihydrite complex (NIFEREX, others)
• Pyridoxine
• Riboflavin
• Romiplostim (NPLATE)
• Sodium ferric gluconate (FERRLECIT)
MECHANISMS OF ACTION OF HEMATOPOIETIC AGENTS
FIGURE 25-1 Sites of action of hematopoietic growth factors in the differentiation and maturation of marrow cell lines. A self-sustaining pool of marrow stem cells differentiates under the influence of specific hematopoietic growth factors to form a variety of hematopoietic and lymphopoietic cells. Stem cell factor (SCF), ligand (FL), interleukin-3 (IL-3), and granulocyte-macrophage colony-stimulating factor (GM-CSF), together with cell–cell interactions in the marrow, stimulate stem cells to form a series of burst-forming units (BFU) and colony-forming units (CFU): CFU-GEMM (granulocyte, erythrocyte, monocyte, and megakaryocyte), CFU-GM (granulocyte and macrophage), CFU-Meg (megakaryocyte), BFU-E (erythrocyte), and CFU-E (erythrocyte). After considerable proliferation, further differentiation is stimulated by synergistic interactions with growth factors for each of the major cell lines—granulocyte colony–stimulating factor (G-CSF), monocyte/macrophage-stimulating factor (M-CSF), thrombopoietin, and erythropoietin. Each of these factors also influences the proliferation, maturation, and, in some cases, the function of the derivative cell line (see Table 25-1).
FIGURE 25-2 Cytokine–cell interactions. Macrophages, T cells, B cells, and marrow stem cells interact via several cytokines (IL-1, IL-2, IL-3, IL-4, IFN [interferon]-γ, GM-CSF, and G-CSF) in response to a bacterial or a foreign antigen challenge. See Table 25-1 for the functional activities of these various cytokines.
FIGURE 25-3 Interrelationships and metabolic roles of vitamin B12 and folic acid. See Side Bar INTERACTIONS OF VITAMIN B12 AND FOLATE IN INTRACELLULAR METABOLISM. FIGLU, formiminoglutamic acid, which arises from the catabolism of histidine; TcII, transcobalamin II; CH3H4PteGlu1, methyltetrahydrofolate.
TABLE 25-1 Hematopoietic Growth Factors
ERYTHROPOIETIN (EPO)
• Stimulates proliferation and maturation of committed erythroid progenitors to increase red cell production
STEM CELL FACTOR (SCF, c-kit ligand, Steel factor) and FLT-3 LIGAND (FL)
• Act synergistically with a wide range of other colony-stimulating factors and interleukins to stimulate pluripotent and committed stem cells
• FL also stimulates both dendritic and NK cells (anti-tumor response)
• SCF also stimulates mast cells and melanocytes
INTERLEUKINS
IL-1, IL-3, IL-5, IL-6, IL-9, and IL-11
• Act synergistically with each other and SCF, GM-CSF, G-CSF, and EPO to stimulate BFU-E, CFU-GEMM, CFU-GM, CFU-E, and CFU-Meg growth
• Numerous immunologic roles, including stimulation of B cell and T cell growth
IL-5
• Controls eosinophil survival and differentiation
IL-6
• IL-6 stimulates human myeloma cells to proliferate
• IL-6 and IL-11 stimulate BFU-Meg to increase platelet production
IL-1, IL-2, IL-4, IL-7, and IL-12
• Stimulate growth and function of T cells, B cells, NK cells, and monocytes
• Co-stimulate B, T, and LAK cells
IL-8 and IL-10
• Numerous immunological activities involving B and T cell functions
• IL-8 acts as a chemotactic factor for basophils and neutrophils
GRANULOCYTE-MACROPHAGE COLONY–STIMULATING FACTOR (GM-CSF)
• Acts synergistically with SCF, IL-1, IL-3, and IL-6 to stimulate CFU-GM, and CFU-Meg to increase neutrophil and monocyte production
• With EPO may promote BFU-E formation
• Enhances migration, phagocytosis, superoxide production, and antibody-dependent cell-mediated toxicity of neutrophils, monocytes, and eosinophils
• Prevents alveolar proteinosis
GRANULOCYTE COLONY–STIMULATING FACTOR (G-CSF)
• Stimulates CFU-G to increase neutrophil production
• Enhances phagocytic and cytotoxic activities of neutrophils
MONOCYTE/MACROPHAGE COLONY–STIMULATING FACTOR (M-CSF, CSF-1)
• Stimulates CFU-M to increase monocyte precursors
• Activates and enhances function of monocyte/macrophages
MACROPHAGE COLONY–STIMULATING FACTOR (M-CSF)
• Stimulates CFU-M to increase monocyte/macrophage precursors
• Acts in concert with tissues and other growth factors to determine the proliferation, differentiation, and survival of a range of cells of the mononuclear phagocyte system
THROMBOPOIETIN (TPO, Mpl ligand)
• Stimulates the self-renewal and expansion of hematopoietic stem cells
• Stimulates stem cell differentiation into megakaryocyte progenitors
• Selectively stimulates megakaryocytopoiesis to increase platelet production
• Acts synergistically with other growth factors, especially IL-6 and IL-11
BFU, burst-forming unit; CFU, colony-forming unit; E, erythrocyte; G, granulocyte; M, macrophage; Meg, megakaryocyte; NK cells, natural killer cells; LAK cells, lymphokine-activated killer cells.
A 64-year-old woman with chronic kidney disease has a low hematocrit and is started on therapy with epoetin alfa.
a. What is the mechanism of action of epoetin alfa?
Recombinant human erythropoietin (epoetin alfa) is nearly identical to the endogenous hormone except that the carbohydrate modification pattern of epoetin alfa differs slightly from the native protein; this difference apparently does not alter kinetics, potency, or immunoreactivity of the drug.
The endogenous protein is heavily glycosylated and expressed primarily in peritubular interstitial cells of the kidney. After secretion, erythropoietin binds to a receptor on the surface of committed erythroid progenitors in the marrow and is internalized. With anemia or hypoxemia, synthesis rapidly increases by 100-fold or more, serum erythropoietin levels rise, and marrow progenitor cell survival, proliferation, and maturation are dramatically stimulated (see Figure 25-1). This finely tuned feedback loop can be disrupted by kidney disease, marrow damage, or a deficiency in iron or an essential vitamin. With an infection or an inflammatory state, erythropoietin secretion, iron delivery, and progenitor proliferation all are suppressed by inflammatory cytokines. There is a clear dose–response relationship between the epoetin alfa dose and the rise in hematocrit in anephric patients, with eradication of their anemia at higher doses.
More recently, a novel erythropoiesis-stimulating protein, darbepoetin alfa, has been approved for clinical use in patients with indications similar to those for epoetin alfa. It is a genetically modified form of erythropoietin in which 4 amino acids have been mutated such that additional carbohydrate side chains are added during its synthesis, prolonging the circulatory survival of the drug up to 24 to 26 hours.
b. What are other indications for erythropoiesis-stimulating agents (ESAs)?
Epoetin alfa also is effective in the treatment of anemia associated with surgery, AIDS, cancer chemotherapy, prematurity, and certain chronic inflammatory conditions. Darbepoetin alfa also has been approved for use in patients with anemia associated with chronic kidney disease and is under review for several other indications.
Highly competitive athletes have used epoetin alfa to increase their hemoglobin levels (“blood doping”) and improve performance. Unfortunately, this misuse of the drug has been implicated in the deaths of several athletes and is strongly discouraged.
c. What are the therapeutic considerations to optimize therapy with epoetin alfa?
During erythropoietin therapy, absolute or functional iron deficiency may develop. Functional iron deficiency (ie, normal ferritin levels but low transferrin saturation) presumably results from the inability to mobilize iron stores rapidly enough to support the increased erythropoiesis. Virtually all patients eventually will require supplemental iron to increase or maintain transferrin saturation to levels that will adequately support stimulated erythropoiesis.
Resistance to epoetin alfa therapy is common in patients who develop an inflammatory illness or become iron deficient, so close monitoring of general health and iron status is essential. Less common causes of resistance include occult blood loss, folic acid deficiency, carnitine deficiency, inadequate dialysis, aluminum toxicity, and osteitis fibrosa cystica secondary to hyperparathyroidism.
d. What are the possible toxicities and hazards of therapy with epoetin alfa?
The most common side effect of epoetin alfa therapy is aggravation of hypertension, which occurs in 20 to 30% of patients and most often is associated with a rapid rise in hematocrit. Blood pressure usually can be controlled either by increasing antihypertensive therapy, ultrafiltration in dialysis patients, or by reducing the epoetin alfa dose to slow the hematocrit response. ESAs should not be used in patients with preexisting uncontrolled hypertension.
Headache, tachycardia, edema, shortness of breath, nausea, vomiting, diarrhea, injection site stinging, and flu-like symptoms (eg, arthralgias and myalgias) also have been reported in conjunction with epoetin alfa therapy.
During hemodialysis, patients receiving epoetin alfa or darbepoetin may require increased anticoagulation. Serious thromboembolic events have been reported, including migratory thrombophlebitis, microvascular thrombosis, pulmonary embolism, and thrombosis of the retinal artery and temporal and renal veins. The risk of thrombotic events, including vascular access thromboses, was higher in adults with ischemic heart disease or congestive heart failure receiving epoetin alfa therapy with the goal of reaching a normal hematocrit (42%) than in those with a lower-target hematocrit of 30%. The higher risk of cardiovascular events from erythropoietic therapies may be associated with higher hemoglobin or higher rates of rise in hemoglobin. The hemoglobin level should be managed to avoid exceeding a target level of 12 g/dL.
ESA use is associated with increased rates of cancer recurrence and decreased on-study survival in patients in whom the drugs are administered for cancer-induced or for chemotherapy-induced anemia. The cause(s) of this effect is presently unclear, but some studies suggest that tumor cells bearing the erythropoietin receptor are more likely to be affected by the use of ESAs.
A 42-year-old man with leukemia will undergo autologous peripheral blood stem cell transplant following high-dose chemotherapy.
a. What is the rationale for using myeloid growth factors in this procedure?
The myeloid growth factors (see Figure 25-1 and Table 25-1) are glycoproteins that stimulate the proliferation and differentiation of 1 or more myeloid cell lines. They also enhance the function of mature granulocytes and monocytes. Recombinant forms of several growth factors have been produced, including granulocyte-macrophage colony-stimulating factor (GM-CSF), granulocyte colony-stimulating factor (G-CSF), interleukin-3 (IL-3), macrophage colony-stimulating factor (M-CSF; also known as colony-stimulating factor, CSF-1), and stem cell factor (SCF).
Recombinant human GM-CSF (sargramostim) and recombinant human G-CSF (filgrastim) are used in patients undergoing autologous bone marrow and peripheral blood stem cell transplant following high-dose chemotherapy to stimulate the proliferation, differentiation, and function of myeloid cell lines that are required to fight infection. Sargramostim and filgrastim shorten the duration of severe neutropenia and reduce transplant morbidity secondary to bacterial and fungal infections without a change in long-term survival or risk of inducing an early relapse of the malignant process.
b. What is the difference in the effects of GM-CSF versus G-CSF?
GM-CSF is capable of stimulating the proliferation, differentiation, and function of a number of the myeloid cell lineages (see Figure 25-1). It acts synergistically with other growth factors, including erythropoietin, at the level of the burst-forming unit (BFU). GM-CSF stimulates the colony-forming units (CFUs) CFU-GEMM, CFU-GM, CFU-M, CFU-E, and CFU-Meg to increase cell production. It also enhances the migration, phagocytosis, superoxide production, and antibody-dependent cell-mediated toxicity of neutrophils, monocytes, and eosinophils.
The activity of G-CSF is restricted to neutrophils and their progenitors, stimulating their proliferation, differentiation, and function. It acts primarily on the CFU-G, although it also can play a synergistic role with IL-3 and GM-CSF in stimulating other cell lines. G-CSF enhances phagocytic and cytotoxic activities of neutrophils. Unlike GM-CSF, G-CSF has little effect on monocytes, macrophages, and eosinophils, and reduces inflammation by inhibiting IL-1, tumor necrosis factor, and interferon γ. G-CSF also mobilizes primitive hematopoietic cells, including hematopoietic stem cells, from the marrow into the peripheral blood. This observation has virtually transformed the practice of stem cell transplantation, such that more than 90% of all such procedures today use G-CSF–mobilized peripheral blood stem cells (PBSCs) as the donor product.
c. What are other indications for G-CSF?
G-CSF also is effective in the treatment of severe congenital neutropenias. In patients with cyclic neutropenia, G-CSF therapy will increase the level of neutrophils and shorten the length of the cycle sufficiently to prevent recurrent bacterial infections. Filgrastim therapy can improve neutrophil counts in some patients with myelodysplasia or marrow damage (moderately severe aplastic anemia or tumor infiltration of the marrow). The neutropenia of AIDS patients receiving zidovudine also can be partially or completely reversed. Filgrastim is routinely used in patients undergoing peripheral blood stem cell (PBSC) collection for stem cell transplantation. It promotes the release of CD34+ progenitor cells from the marrow, reducing the number of collections necessary for transplant. Moreover, filgrastim-mobilized PBSCs appear more capable of rapid engraftment. PBSC-transplanted patients require fewer days of platelet and red blood cell transfusions and a shorter duration of hospitalization than do patients receiving autologous bone marrow transplants.
INTERACTIONS OF VITAMIN B12 AND FOLATE IN INTRACELLULAR METABOLISM (See Figure 25-3)
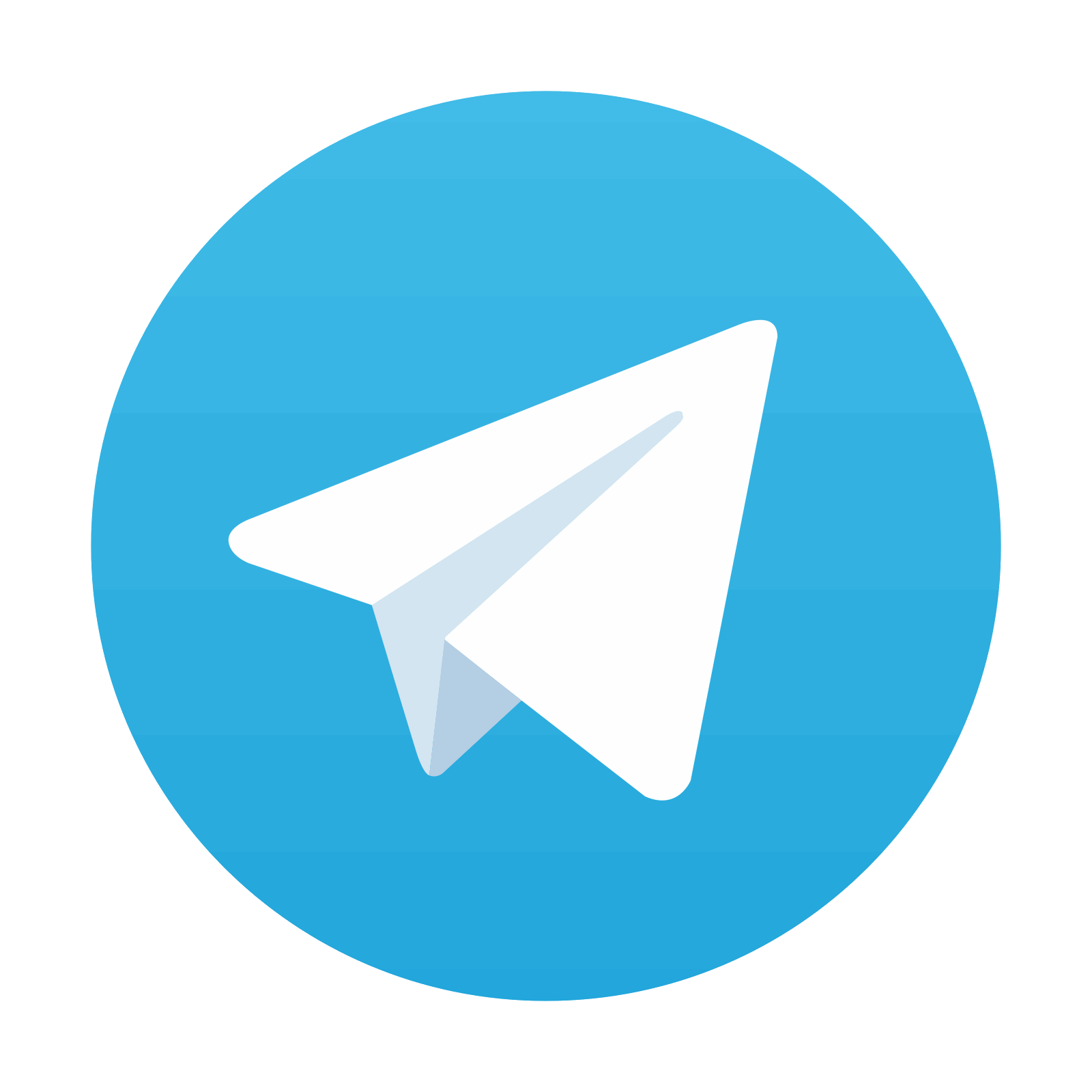
Stay updated, free articles. Join our Telegram channel

Full access? Get Clinical Tree
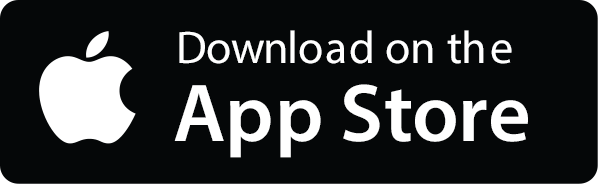
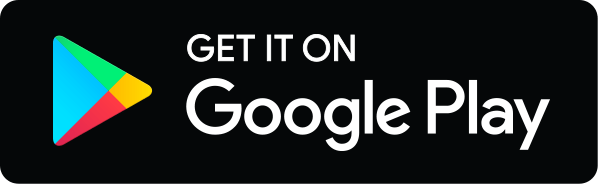