18
Glycolysis & the Oxidation of Pyruvate
David A. Bender, PhD & Peter A. Mayes, PhD, DSc
OBJECTIVES
After studying this chapter, you should be able to:
Describe the pathway of glycolysis and its control and explain how glycolysis can operate under anaerobic conditions.
Describe the reaction of pyruvate dehydrogenase and its regulation.
Explain how inhibition of pyruvate metabolism leads to lactic acidosis
BIOMEDICAL IMPORTANCE
Most tissues have at least some requirement for glucose. In the brain, the requirement is substantial, and even in prolonged fasting the brain can meet no more than about 20% of its energy needs from ketone bodies. Glycolysis, the major pathway for glucose metabolism, occurs in the cytosol of all cells. It is unique, in that it can function either aerobically or anaerobically, depending on the availability of oxygen and the electron transport chain. Erythrocytes, which lack mitochondria, are completely reliant on glucose as their metabolic fuel, and metabolize it by anaerobic glycolysis. However, to oxidize glucose beyond pyruvate (the end product of glycolysis) requires both oxygen and mitochondrial enzyme systems: the pyruvate dehydrogenase complex, the citric acid cycle (Chapter 17), and the respiratory chain (Chapter 13).
Glycolysis is both the principal route for glucose metabolism and also the main pathway for the metabolism of fructose, galactose, and other dietary carbohydrates. The ability of glycolysis to provide ATP in the absence of oxygen is especially important, because this allows skeletal muscle to perform at very high levels of work output when oxygen supply is insufficient, and it allows tissues to survive anoxic episodes. However, heart muscle, which is adapted for aerobic performance, has relatively low glycolytic activity and poor survival under conditions of ischemia. Diseases in which enzymes of glycolysis (eg, pyruvate kinase) are deficient are mainly seen as hemolytic anemias or, if the defect affects skeletal muscle (eg, phosphofructokinase), as fatigue. In fast-growing cancer cells, glycolysis proceeds at a high rate, forming large amounts of pyruvate, which is reduced to lactate and exported. This produces a relatively acidic local environment in the tumor, which may have implications for cancer therapy. The lactate is used for gluconeogenesis in the liver (Chapter 20), an energy-expensive process, which is responsible for much of the hypermetabolism seen in cancer cachexia. Lactic acidosis results from various causes, including impaired activity of pyruvate dehydrogenase, especially in thiamin (vitamin B t) deficiency.
GLYCOLYSIS CAN FUNCTION UNDER ANAEROBIC CONDITIONS
Early in the investigations of glycolysis it was realized that fermentation in yeast was similar to the breakdown of glycogen in muscle. It was noted that when a muscle contracts in an anaerobic medium, that is, one from which oxygen is excluded, glycogen disappears and lactate appears. When oxygen is admitted, aerobic recovery takes place and lactate is no longer produced. However, if contraction occurs under aerobic conditions, lactate does not accumulate and pyruvate is the major end product of glycolysis. Pyruvate is oxidized further to CO2 and water (Figure 18–1). When oxygen is in short supply, mitochondrial reoxidation of NADH formed during glycolysis is impaired, and NADH is reoxidized by reducing pyruvate to lactate, so permitting glycolysis to proceed (Figure 18–1). While glycolysis can occur under anaerobic conditions, this has a price, for it limits the amount of ATP formed per mole of glucose oxidized, so that much more glucose must be metabolized under anaerobic than aerobic conditions. In yeast and some other microorganisms, pyruvate formed in anaerobic glycolysis is not reduced to lactate, but is decarboxylated and reduced to ethanol.
FIGURE 18–1 Summary of glycolysis. , blocked by anaerobic conditions or by absence of mitochondria containing key respiratory enzymes, as in erythrocytes.
THE REACTIONS OF GLYCOLYSIS CONSTITUTE THE MAIN PATHWAY OF GLUCOSE UTILIZATION
The overall equation for glycolysis from glucose to lactate is as follows:
All of the enzymes of glycolysis (Figure 18–2) are found in the cytosol. Glucose enters glycolysis by phosphorylation to glucose 6-phosphate, catalyzed by hexokinase, using ATP as the phosphate donor. Under physiologic conditions, the phosphorylation of glucose to glucose 6-phosphate can be regarded as irreversible. Hexokinase is inhibited allosterically by its product, glucose 6-phosphate.
FIGURE 18–2 The pathway of glycolysis. (,
, inhibition.) *Carbons 1-3 of fructose bisphosphate form dihydroxyacetone phosphate, and carbons 4-6 form glyceraldehyde 3-phosphate. The term “bis-,” as in bisphosphate, indicates that the phosphate groups are separated, whereas the term “di-,” as in adenosine diphosphate, indicates that they are joined.
In tissues other than the liver (and pancreatic β-islet cells), the availability of glucose for glycolysis (or glycogen synthesis in muscle, Chapter 19, and lipogenesis in adipose tissue, Chapter 23) is controlled by transport into the cell, which in turn is regulated by insulin. Hexokinase has a high affinity (low Km) for glucose, and in the liver it is saturated under normal conditions, and so acts at a constant rate to provide glucose 6-phosphate to meet the liver’s needs. Liver cells also contain an isoenzyme of hexokinase, glucokinase, which has a Km very much higher than the normal intracellular concentration of glucose. The function of glucokinase in the liver is to remove glucose from the blood following a meal, providing glucose 6-phosphate in excess of requirements for glycolysis, which is used for glycogen synthesis and lipogenesis. Glucokinase is also found in pancreatic β-islet cells, where it functions to detect high concentrations of glucose; onward metabolites of the glucose 6-phosphate formed stimulate the secretion of insulin.
Glucose 6-phosphate is an important compound at the junction of several metabolic pathways: glycolysis, gluconeogenesis, the pentose phosphate pathway, glycogenesis, and glycogenolysis. In glycolysis, it is converted to fructose 6-phosphate by phosphohexose isomerase, which involves an aldose-ketose isomerization. This reaction is followed by another phosphorylation catalyzed by the enzyme phosphofructokinase (phosphofructokinase-1) forming fructose 1,6-bisphosphate. The phosphofructokinase reaction may be considered to be functionally irreversible under physiologic conditions; it is both inducible and subject to allosteric regulation, and has a major role in regulating the rate of glycolysis. Fructose 1,6-bisphosphate is cleaved by aldolase (fructose 1,6-bisphosphate aldolase) into two triose phosphates, glyceraldehyde 3-phosphate and dihydroxyacetone phosphate, which are interconverted by the enzyme phosphotriose isomerase.
Glycolysis continues with the oxidation of glyceraldehyde 3-phosphate to 1,3-bisphosphoglycerate. The enzyme catalyzing this oxidation, glyceraldehyde 3-phosphate dehydrogenase
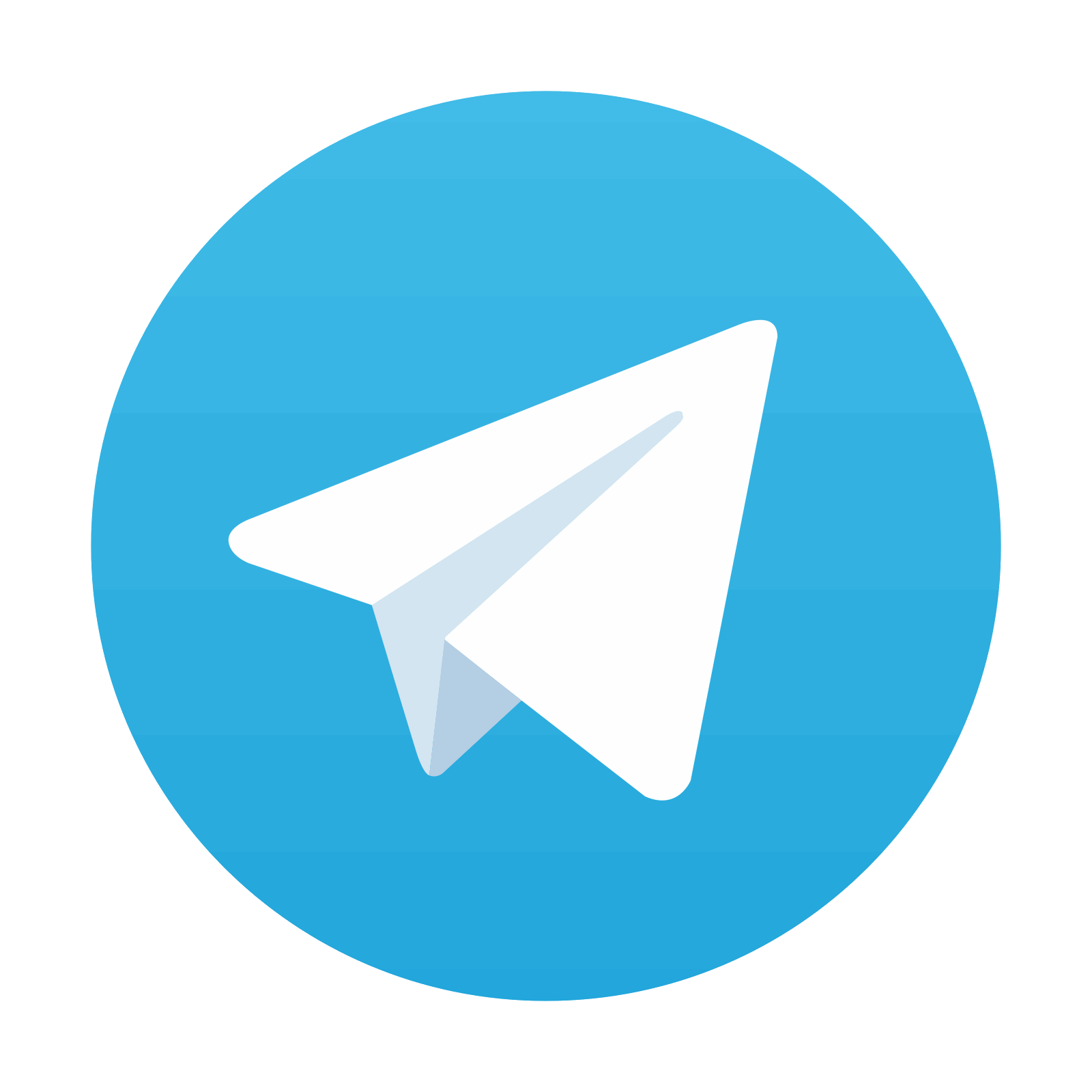
Stay updated, free articles. Join our Telegram channel

Full access? Get Clinical Tree
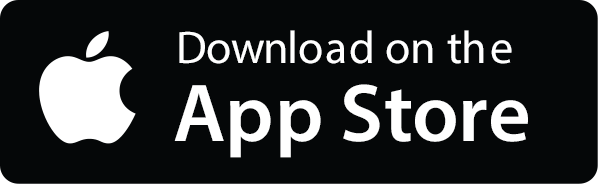
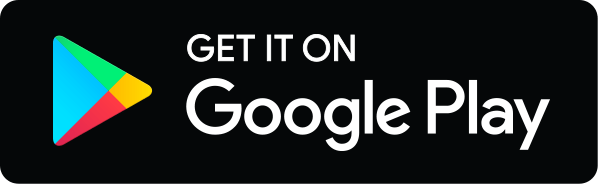