© Springer Science+Business Media New York 2015
Cristina Magi-Galluzzi and Christopher G. Przybycin (eds.)Genitourinary Pathology10.1007/978-1-4939-2044-0_4242. Genetic and Epigenetic Alterations in Testicular Tumors
(1)
Department of Pathology, Cleveland Clinic, Robert J. Tomsich Pathology and Laboratory Medicine Institute, Cleveland, OH, USA
Keywords
TestisGerm cell tumorsGenetic alterationsEpigeneticMethylationChromatin remodelingMicroRNAIntroduction
Testicular germ cell tumors (TGCT) originate from the malignant counterpart of primordial germ cells/gonocytes. Various studies have suggested an inherited predisposition to the development of these tumors, based on the increased risks associated with a positive family history, the higher frequency of bilaterality in familial cases, and the ethnic and racial differences. However, no high-penetrance susceptibility gene has yet been identified, suggesting a genetic architecture in which multiple loci contribute to testicular germ cell tumors susceptibility.
The only recurrent cytogenetic alteration detected in TGCTs is the gain of the short arm of chromosome 12, mostly as isochromosomes.
Candidate gene studies have identified two loci of interest, that is, the Y-chromosome gr/gr deletion and the PDE11A gene, while recent genome-wide association studies have identified at least three genes involved in the KITLG/KIT signaling pathway (KITLG, SPRY4 and BAK1).
Although DNA hypermethylation plays a crucial role in tumorigenesis, aberrant de novo methylation of tumor suppressor genes or tumor-related genes is a rare event in TGCT, particularly in seminomas, although there have been reports on differentially methylated genes among seminomatous and non-seminomatous TGCTs.
MicroRNAs and Piwi-interacting RNAs are increasingly seen as important elements in both gonadal development and spermatogenesis and their pathologies.
Recent expression profiling studies of TGCTs along with advances in embryonic stem-cell research have contributed to our markedly improved understanding of the pathogenesis of testicular cancer. However, many questions remain unanswered and among them probably the most important one concerns the etiology of TGCTs and the relative roles of genetic versus environmental or lifestyle factors.
Genetic Alterations in Testicular Tumors
Though relatively rare, testicular (and extragonadal) germ cell tumors are the most common solid tumors in young men aged 18–35 years and represent the leading cause of cancer-related morbidity and mortality in this group.
Adult male TGTCs arise from malignant transformation of the same precursor, a totipotent germ cell [1], called carcinoma in situ (CIS)/intratubular germ cell neoplasia or testicular intraepithelial neoplasia (ITGCN). The process starts prenatally, is often associated with some degree of gonadal dysgenesis , and involves the acquisition of specific genetic aberrations and subsequent epigenetic alterations [2]. Evidence from morphological, epidemiological, immunohistochemical, and gene expression profiling studies indicates that the ITGCN cell is derived from a gonocyte or primordial germ cell [3]. ITGCN and seminomatous cells are characterized by expression of OCT3/4 and NANOG, all identified as transcription factors related to pluripotency in embryonic stem cells.
Family history of TGCT is one of the strongest and most consistent risk factors for this tumor. Approximately 1.4 % of newly diagnosed TGCT patients report a positive family history of TGCT. Bilateral testicular germ cell tumors are more likely to occur in familial aggregations than in sporadic cases. Studies have estimated that brothers of affected cases have an eight-to tenfold increased relative risk compared with the general population and fathers/sons of TGCT cases have a four- to sixfold higher risk [4]. These high familial risks suggest that inherited susceptibility and/or environmental factors that cluster in families may play a substantial role in the etiology of a significant portion of TGCT cases. Genome-wide screens subsequently provided evidence of a TGCT susceptibility gene on chromosome Xq27 (TGCT1) that might also predispose to cryptorchidism. However, this putative gene has yet to be identified, and other TGCT susceptibility genes probably exist [5]. Although the familial relative risk of TGCT is considerably higher than for most other cancers, the absolute risk is comparatively low (lifetime risk approximately 1⁄230 in Caucasian men). Since no specific high-penetrance susceptibility gene has been identified, it is likely that the combined contribution of multiple common alleles, each conferring modest risk, might underlie familial testicular cancer [6].
ITGCN, seminomas, and all variants of non-seminomatous germ cell tumors (NSGCT) are characterized by marked aneuploidy in nearly all cases as well as specific chromosome gains and losses. The only recurrent cytogenetic alteration is the gain of the short arm of chromosome 12, mostly as isochromosomes [7, 8]. Specifically, isochromosome 12p is the most common alteration (~ 80 %), with duplication of 12p and amplification of shorter stretches of 12p being much less common. While seminomas show high-level amplification of 12p, gain of proximal 17q and loss of 10q have been detected in NSGCTs [9]. Interestingly, ITGCN without adjacent invasive TGCT does not contain isochromosome 12p in most studies, which suggests that isochromosome 12p is not required for the development of ITGCN [10]. It is believed that the gain of 12p is important in tumor progression and occurs subsequent to aneuploidy. This gain appears to be multifunctional in germ cell tumorigenesis on the basis of the observed overexpression of several candidate genes [including KITLG, NANOG (and its pseudogenes), KRAS2, BCAT1, and CCND2] mapped to this region involved in maintenance of pluripotency and oncogenesis.
Further studies are required before we fully understand the role of chromosome 12p in TGCT carcinogenesis.
Compared to chromosomal abnormalities, specific gene mutations in TGCTs are less frequent. The most frequent single genes affected in TGCTs are KIT, K-RAS, N-RAS, and B-RAF [11]. Eight percent of TGCTs have mutations in KIT, 5 % in K-RAS, 3 % in N-RAS, 8 % in MADH4, and 1 % in STK10. Somatic mutations have also been identified in TP53, MET, SNF1LK, and PTEN [12]. Copy number changes are relatively common in TGCT, and point mutations are relatively rare when compared with other cancer types.
Seminomatous and NSGCTs are much more frequent in the testis than in the ovary, which suggests a link to the Y chromosome. This idea is strengthened by the fact that patients with disorders of sex development (DSD) are at risk for development of TGCTs.
The Y chromosome carries a number of testis- and germ cell-specific genes. The region of interest on the Y chromosome is the so-called male-specific Y (MSY) region, which contains high density of genes from nine families, each gene existing in multiple (2–35), near-identical copies [13]. Genes within the MSY are expressed predominantly or exclusively in the testis and are believed to contribute to the development and proliferation of germ cells.
Candidate association studies in sporadic TGCT have detected a Y chromosome 1.6-Mb deletion (designated gr⁄gr), previously implicated in spermatogenic failure and subfertility, in 3.0 % of TGCT patients with a family history, 2 % of TGCT cases without a family history, and 1.3 % of unaffected male controls, demonstrating that the deletion confers an approximately twofold risk of TGCT [14]. The association between gr⁄gr and TGCT is stronger for seminomas than for NSGCTs. However, because of its low frequency and modest risk, this microdeletion accounts for approximately 0.5 % of the excess familial risk of TGCT.
Genetic susceptibility conditioning familial TGCTs has been established, confirming mutations or single nucleotide polymorphisms (SNPs) affecting some genes involved in normal germ cell differentiation such as KITLG, SPRY4, PDE11A, and BAK1 [15].
Genome-wide association studies (GWAS) [16–18] utilizing TGCT samples from the UK have recently identified eight SNPs at six loci: 12q21 (KITLG), 5q31 (SPRY4), 6p21 (BAK1), 5p15 (TERT-CLPTM1L), 9p24 (DMRT1), and 12p13 (ATF7IP). The loci at 5q31, 9p24, and 12q21 were independently reported with consistent effect by Kanetsky et al. in their GWAS comprising cases of TGCT from the USA [19]. KITLG, SPRY4, and BAK1 are all involved in the KITLG⁄KIT signaling pathway. KITLG⁄ KIT system regulates survival, proliferation, and migration of germ cells [20], and germ line homozygous null mutations of either gene in mice cause infertility due to failure of progenitor germ cell development. SPRY4 is an inhibitor of the mitogen-activated protein kinase pathway that is activated by the KITLG⁄KIT pathway. BAK1 encodes a protein that promotes apoptosis by binding to and antagonizing the apoptosis repressor activity of BCL2 and other antiapoptotic proteins. BAK1 expression in TCGT is repressed by the KITLG⁄KIT pathway, and the interaction of BAK1 with antiapoptotic proteins is implicated in germ-cell apoptosis that occurs in response to the blockade of KITLG⁄KIT pathway [21]. TERT and ATF7IP relate to a pathway of telomerase regulation: TERT encodes telomerase while ATF7IP regulates expression of TERT and its partner TERC. DMRT1 relates to a pathway of sex determination [18].
Since frequencies of risk alleles at 12q21 are much lower in the African population than in the Caucasian, the 12q21 locus may, in part, account for the different frequency of TGCT observed between ethnic groups [22].
Although these SNPs may be biologically significant, these six loci together with gr/gr deletion account only for approximately 15 % of the excess familial risk of TGCT. The remaining 85 % of genetic predisposition is yet unexplained and requires further investigation [18].
Epigenetics of Testicular Tumors
The term “epigenetics” refers to heritable changes in gene function that occur without any change in the DNA sequence. Epigenetics is regulation of gene expression and can be essentially transmitted to daughter cells formed after meiosis or mitosis [23] and possibly also through generations. Environmental factors can affect epigenetic processes. So far, three main epigenetic mechanisms are known: DNA methylation , chromatin remodeling , and microRNA regulation . Of those, DNA methylation is the best known and most thoroughly studied epigenetic mechanism [24].
Methylation
DNA methylation , a key component of the epigenome involved in regulating gene expression, is initially acquired in the germ line at millions of sites across the genome. Hypermethylation of CpG islands (sequences rich in CpG) associated with silencing of tumor suppressor genes or tumor-related genes is a common hallmark of human cancer. TGCTs have distinctive DNA methylation profiles that differ from those of somatic tissue-derived cancers or somatic tissues [25]. TGCTs exhibit greater degree of hypomethylation compared to other cancers [26].
TGCT methylation patterns are similar to those exhibited by primordial germ cells [27]. Seminomas are basically devoid of DNA methylation and NSGCTs in general have methylation levels comparable with other tumor tissues. In general, DNA methylation seems to increase with differentiation, and among NSGCTs, undifferentiated embryonal carcinomas harbor the lowest levels of DNA promoter hypermethylation, whereas well-differentiated teratomas display the highest [28].
So far, a limited number of tumor suppressor genes have been found inactivated by DNA promoter hypermethylation in more than a minor percentage of TGCTs, including MGMT, SCGB3A1, RASSF1A, HIC1, and PRSS21. RASSF1A methylation has been detected in 40 % of seminomas and 83 % of NSCGT components. These findings are consistent with a multistep model in which RASSF1A methylation occurs early in TGCT tumorigenesis and additional epigenetic events characterize progression from seminoma to NSGCT [29]. PRSS21 (testisin gene at 16p13), a serine protease abundantly expressed only in normal testes, is thought to be a tumor suppressor gene silenced by aberrant methylation in TGCTs [30]. CpG sites in the 5′ untranslated region proved to be relevant to testisin gene silencing when methylated. It has been demonstrated that the median normalized index of methylation is 8.6 times higher in TGCTs than in normal testicular samples, and significantly higher in NSGCTs than in seminomas.
LINE1 and Alu are two major DNA repetitive elements, which consist of interspersed and tandem repeats. LINE1 is a long group of interspersed nucleotide elements that constitutes at least 18 % of the human genome. The Alu repetitive element is the most abundant short interspersed nucleotide element in the human genome and accounts for about 10 % of the entire genome [24]. Alu and LINE1 elements are normally heavily methylated and contain much of the CpG methylation found in normal human tissues. However, both LINE1 and Alu repeats are extensively unmethylated in seminomas, whereas in NSGCTs the LINE1 sequence is extensively unmethylated, but Alu elements are methylated, confirming a difference in degree of methylation between seminomatous and NSGCT.
Imprinting defects, DNA hypomethylation of testis/cancer associated genes, and presence of unmethylated XIST (X inactive specific transcript) are frequent in TGCTs [28]. TGCTs contain supernumerical X chromosomes in a hypomethylated state at the 5′ end. XIST is expressed exclusively from the inactive form of the X chromosome and is thought to be involved in the inactivation process of female X chromosomes. XIST gene expression has been found in seminomas (83 %) but less frequently in NSGCTs (25 %) [31].
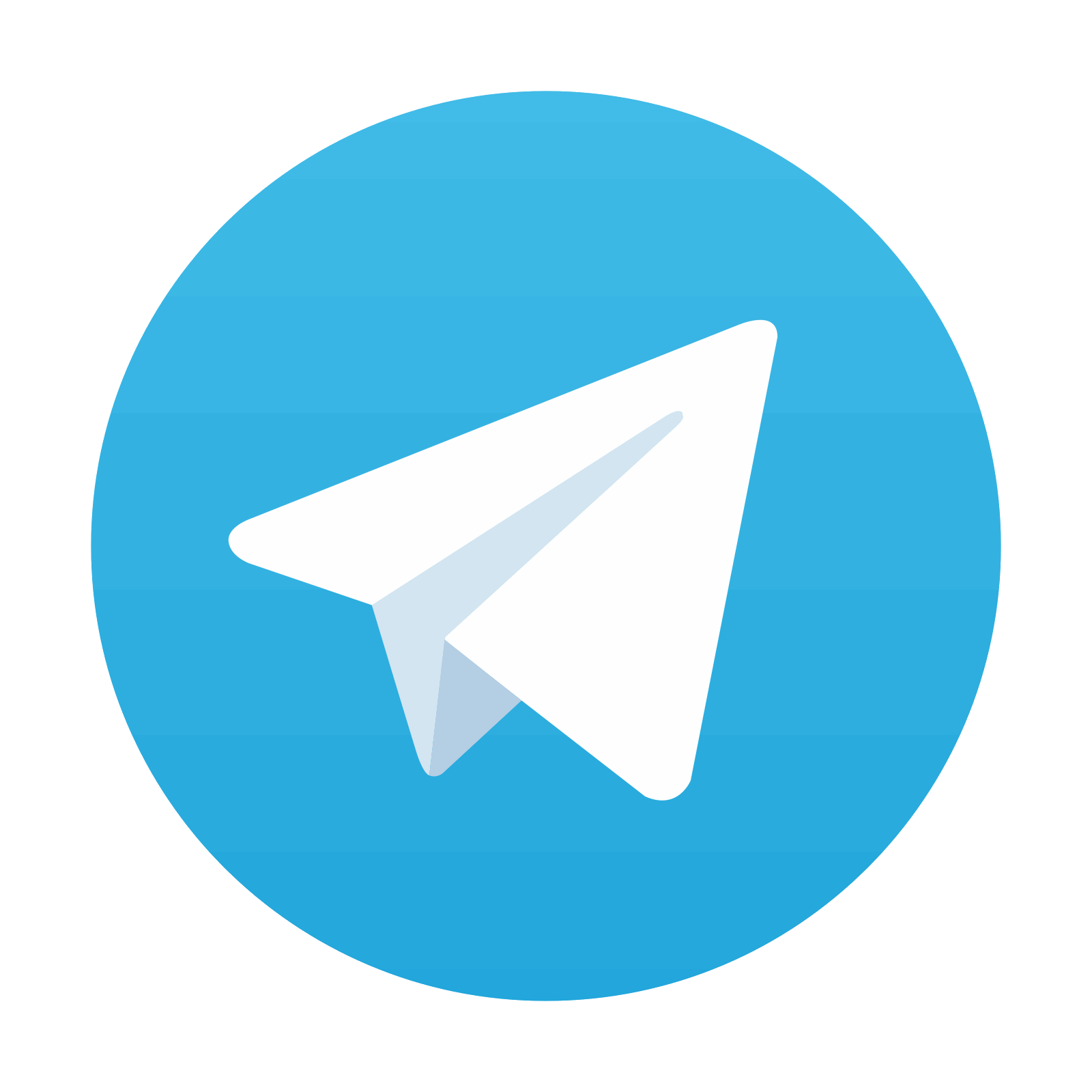
Stay updated, free articles. Join our Telegram channel

Full access? Get Clinical Tree
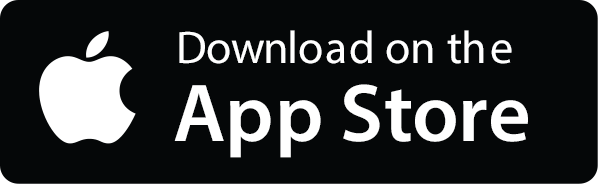
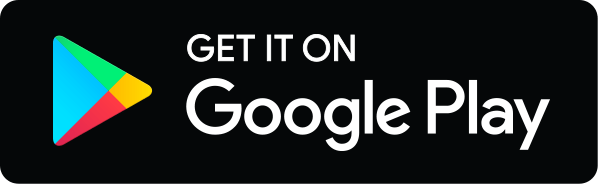