© Springer Science+Business Media New York 2015
Cristina Magi-Galluzzi and Christopher G. Przybycin (eds.)Genitourinary Pathology10.1007/978-1-4939-2044-0_3232. Genetic and Epigenetic Alterations in Renal Cell Carcinoma
(1)
Department of Pathology, New York University Langone Medical Center, New York, NY, USA
Keywords
Renal cell carcinomaSubtypesGeneticsEpigeneticsPrognosisManagementIntroduction
Renal cell carcinoma (RCC), a group of heterogeneous tumors arising from the epithelium of the renal tubules, accounts for greater than 90 % of all malignancies in the adult kidney. Classified according to the 2004 World Health Organization criteria, these tumors have unique clinical and pathological features [1]. Cytogenetic and molecular studies have also demonstrated that each histological subtype demonstrates specific genetic changes. Although different histological subtypes of RCC demonstrate unique pathogenesis and genetic alterations, the impact of histological subtypes on prognosis remains controversial [2]. In view of specific genomic and epigenetic alterations associated with a specific RCC subtype, implementation of genetic and epigenetic markers in the management of RCC might become helpful in the future.
Genetic Alterations and Pathogenesis
Clear cell RCC (CCRCC) is the most common subtype, accounting for 60–75 % of RCCs. The most common and characteristic genetic change in CCRCC is an alteration of the short arm of chromosome 3 (−3p, > 90 %), followed thereafter by changes in other chromosomal regions, including 5q, 6q, 8p, 9p, 10p, and 14q [3]. Biallelic inactivation, such as by loss of one 3p arm and mutations on the second chromosome 3, is a key event in the tumor development of CCRCC. Additional chromosomal aberrations are often associated with tumor progression. At least three regions harboring several different genes on 3p have been implicated, including the von Hippel–Lindau ( VHL) gene on 3p25-26, the FHIT gene on 3p11-12, and the RASSF1Aand DRR1 genes on 3p21-22. VHL functions as a tumor suppressor and plays a critical role in the hypoxia-inducible factor (HIF) pathway. Mutations in the VHL gene lead to increased activation of the HIF pathway and its downstream target genes such as vascular endothelial growth factor ( VEGF), platelet-derived growth factor ( PGDF), epidermal growth factor ( EGF), carbonic anhydrase ( CAIX), Glut-1, and erythropoietin. These genes act in concert to promote deregulated epithelial proliferation and angiogenesis and therefore appear to contribute to the pathogenesis of CCRCC. The majority of CCRCCs are sporadic, with less than 5 % occurring in patients with inherited cancer syndromes such as von Hippel–Lindau disease, tuberous sclerosis, and constitutional chromosome 3 translocation syndrome.
Papillary RCC (PRCC) accounts for 10–15 % of RCCs. Gain of chromosomes 7 and 17 and loss of Y chromosome are the most common cytogenetic changes [4]. Additional gain of chromosomes 3, 12, 16, 20, and other chromosomes is often associated with tumor progression. The responsible genes that underlie several hereditary forms of PRCC have been identified including c-Met gene mutations (7q31) in hereditary papillary renal cell carcinoma syndrome (HPRCC) and fumarate hydratase (FH) mutations (1q42) in hereditary leiomyomatosis/renal cell carcinoma syndrome (HLRCC). Gain-of-function mutations in c-MET result in altered cellular processes related to renal papillary carcinogenesis, although these mutations are uncommon in sporadic PRCC. Fumarate hydratase functions as an enzyme that converts fumarate to malate in the tricarboxylic acid cycle. Recent studies suggest that fumarate hydratase also regulates the stability of hypoxia-inducible factors (HIFs) and may therefore play a role in renal carcinogenesis.
Chromophobe RCC (ChRCC) accounts for 5 % of RCC. It frequently has multiple complex chromosomal losses, including Y, 1, 2, 6, 10, 13, 17, and 21 [5]. Renal oncocytoma, a benign tumor that may bear morphological resemblance to chromophobe RCC, is characterized by alterations involving chromosome 11q, partial or complete losses of chromosomes 1 or 14, or a sex chromosome (Y or X). ChRCC and renal oncocytoma share some cytogenetic similarity, although the former typically demonstrates more complex karyotypic alterations than the latter. Patients with Birt–Hogg–Dube syndrome, the gene for which is mapped to 17p11.2, often develop ChRCC, oncocytoma, and hybrid tumors with features of both ChRCC and oncocytoma.
Recently, several distinct RCCs with chromosomal translocations involving the TFE3 gene at Xp11.2 and TFEB gene at 6p21 have been described [6]. The translocation results in overexpression of fusion proteins that harbor the DNA-binding domains from TFE3 and TFEB, and this overexpression has been hypothesized to function in the pathogenesis of this unique class of RCC.
Epigenetic Alterations in RCC
DNA Methylation
DNA methylation , a covalent chemical modification resulting in addition of a methyl group at the carbon 5 position of the cytosine ring in CpG dinucleotides, is one of the most consistent epigenetic changes occurring in human cancer. Morris et al. [7], in a genomewide methylation analysis, found a significant correlation between tumor suppressor gene SCUBE3 DNA methylation and an increased risk of cancer death or relapse in RCC patients. In addition, aberrant promoter methylation of DLEC1 (a tumor suppressor gene at 3p22) is associated with more advanced tumor stage and higher grade [8]. Methylation of microRNA genes miR-9-1 and miR-9-3 is associated with RCC tumor recurrence and decrease in recurrence-free survival time [9].
DNA Hypomethylation
Histone Modification and Chromatin Remodeling
Little data on histone modification and prognosis of RCC patients have been published. Ellinger et al. [12] evaluated histone H3 lysine 4 mono-methyl (H3K4me1), di-methyl (H3K4me2) and trimethyl (H3K4me3) patterns in renal cell carcinoma (RCC) using a tissue microarray with 193 RCC (including 142 clear cell, 31 papillary, 10 chromophobe, and 10 sarcomatoid RCCs) and 10 oncocytoma specimens. H3K4me3 staining was more intense in papillary RCC, whereas H3K4me1 and H3K4me2 were similar in the diverse RCC subtypes. H3K4me1–3 levels were inversely correlated with Fuhrman grading, pT stage, lymph node involvement, and distant metastasis. Progression-free survival and cancer-specific survival were shorter in patients with low levels of H3K4me1–3 in the univariate analysis, but they did not observe a significant correlation of a single modification in a multivariate model, which also included the established prognostic parameters TNM stage and Fuhrman grade. In comparison, the H3K4me score, which combined staining levels of the H3K4 modifications, was an independent predictor of RCC progression-free survival. Studies from this group also showed that H3 lysine 27(H3K27) methylation levels were inversely correlated with Fuhrman grading and pT stage. Progression-free survival was shorter in patients with lower levels of H3K27me1 and H3K27me3 in the univariate analysis [13].
Recent studies identified several frequent mutations of histone modifying and chromatin remodeling genes in CCRCC. These include PBRM1, a subunit of the PBAF AWI/SNF chromatin remodeling complex [14], ARID1A, a subunit of the BAF AWI/SNF chromatin remodeling complex [15], histodeubiquitinase BAP1 [16, 17], histone demethylase KDM5C [18], and histone methyltransferase SETD2 [19]. Most mutations of these chromatin modulators discovered in CCRCC are loss of function, implicating major roles for epigenetic regulation of additional functional pathways participating in the development and progression of these diseases. Clinical data have shown these mutations are associated with advanced stage, grade, and tumor invasion [20, 21].
MicroRNA Expression
MicroRNAs (miRNAs) , non-coding RNAs regulating gene expression, are frequently aberrantly expressed in human cancers. To date, there are more than 100 publications on microRNA expression and RCC diagnosis, recurrence, and metastasis. Several panels/clusters of miRNAs have been proposed to predict the recurrence and metastatic potentials of RCC. It is interesting to note that as miRNA can be easily detected and quantified in blood, serum assays based on these metastasis-associated miRNAs may be of value. However, further studies in larger patient cohorts are necessary to validate the potential value of microRNA as prognostic biomarkers .
Diagnostic Markers and Applications
Chromosome 3p Alterations
The loss of DNA sequences on chromosome 3p is one of the primary and most frequent events in the pathogenesis of CCRCC [22]. Loss of heterozygosity (LOH) and comparative genetic hybridization (CGH) analyses of CCRCCs have revealed that allelic (interstitial) losses predominantly occur in the chromosome 3p21 region in combination with either 3p25 or 3p13-14, or with both, and these allelic losses are restricted to CCRCC. Chromosome 3p alterations are observed in over 96 % of sporadic and hereditary RCC. Deletion of 3p is the only karyotypic finding in 15 % of non-papillary RCC and in RCC as small as 1 mm. Even the sarcomatoid component of CCRCC retains characteristic 3p alterations. Chromosome 3p alteration is detected in only 8 % of papillary RCC; however, those PRCCs have cytological characteristics of CCRCC. Therefore, loss of 3p is highly specific for CCRCC, and the presence of 3p alteration can provide support for a diagnosis of CCRCC. Detection of 3p changes can be accomplished by conventional cytogenetics , LOH using probes mapped to 3p regions, and CGH.
Quantification of Chromosomes 7, 17
PRCC often demonstrates chromosomal gain, most frequently gain of chromosomes 7 and 17, which are present in 68–75 and 67–80 % of PRCC, respectively [4, 23]. However, trisomy 7 is also a common finding in several other human cancers and in 18–30 % of non-papillary RCC, normal renal cells, and several non-malignant conditions. Therefore, trisomy 7 is, by itself, not specific for PRCC. In contrast, a non-random gain of chromosome 17 is uncommon in other forms of RCC (present in 2.6 % of CCRCC) and other human cancers and, therefore, is a genetic finding fairly specific to PRCC.
Quantification of chromosomes 7 and 17 can be accomplished by cytogenetic study, comparative genomic hybridization (CGH), and fluorescence in situ hybridization (FISH). FISH can be conveniently performed on formalin-fixed, paraffin-embedded tissues using centromeric probes for chromosomes 7 and 17. Brown et al. [24] described a method to isolate intact nuclei from paraffin sections. The majority of the published studies have used tissue sections of 4–8 μm in thickness. To account for the potential nuclear truncation artifact that may affect the chromosomal copy counts on formalin-fixed, paraffin-embedded tissue sections, a normal range is established based on the copy counts of chromosomes 7 and 17 in the adjacent normal kidney tissue.
TFE3, TFEB, and RCC Associated with MITF/TFE Gene Translocation
Some RCCs are associated with chromosomal translocations involving specific genes. RCC associated with Xp11.2 translocation/TFE3 gene fusion is defined by chromosomal translocation involving the TFE3 gene on chromosome Xp11.2, resulting in the overexpression of the TFE3 protein, a member of the MITF/TFE transcriptional factor family [6]. The translocation partner genes include PRCC on 1q21, ASPL on 17q25, PSL on 1p34, NonO on Xq12, and CLTC on 17q23. These carcinomas typically affect children and young adults . Although RCC accounts for less than 5 % of pediatric renal tumors, Xp11.2-associated RCCs make up a significant proportion of these cases. The RCC involving ASPL-TFE3 translocation characteristically presents at an advanced stage and also with lymph node metastasis . The morphology varies slightly with different chromosomal translocations; however, the most distinctive histological feature is the presence of papillary structures lined with clear cells.
Molecular genetic analysis for the chromosomal translocation involving the TFE3 gene provides the most definitive evidence. Immunohistochemical stains for the TFE3 protein, on the other hand, offer a simple, sensitive, and specific assay for the Xp11-translocation RCC on formalin-fixed and paraffin-embedded tissues. As it is tightly regulated in normal tissues, TFE3 is undetectable on routine immunohistochemistry even though it is a ubiquitously expressed nuclear transcriptional factor. TFE3 fusion proteins, in contrast, are overexpressed in Xp11 translocation RCC and can therefore be detected by immunohistochemical staining. Argani et al. [25] reported that the TFE3 protein could be detected by immunohistochemistry in 20/21 (95.2 %) of Xp11.2 translocation RCC confirmed molecularly. The only case that was negative for TFE3 was fixed in Bouin’s fixative. It is known that TFE3 protein is labile and its antigenicity is affected by fixation, with more intense staining at the periphery of the tissue section. However, TFE3 immunostaining is not entirely specific for the Xp11.2 translocation RCC, as positive staining can be detected in 29 % of perivascular epithelioid cell tumor (PECOMA) of soft tissue and gynecological tract, and rarely in other tumors, including adrenal cortical carcinoma, granular cell tumor, bile duct carcinoma, and high-grade myxofibrosarcoma [26].
Another variant of RCC harbors a t(6;11)(p21;q12) translocation that results in overexpression of TFEB transcriptional factor on 6p21, another member of the MITF/TFE family. Similar to Xp11.2 translocation RCC, RCC associated with TFEB also predominantly affects children and young adults. The characteristic morphology includes a biphasic population of larger and smaller epithelioid cells, with the latter typically clustered around hyaline basement membrane material. The diagnosis can be confirmed by the immunohistochemical stain for TFEB protein, which is both sensitive and specific for RCC associated with TFEB, as it is not detectable in other neoplasms and normal tissues. More recently, Malouf et al. [27] found genomic heterogeneity of translocation RCC (TRCC) that included alterations common with clear cell RCC (e.g., 3p loss) and papillary RCC (e.g., trisomy 7 and/or 17). When compared with young patients (< 18 years), adults with TRCC displayed distinct genomic and epigenetic aberrations, exemplified by lower LINE-1 methylation and frequent 17q partial gain, which were consistent with a large-scale dosage effect affecting RCC carcinogenesis. The results show that besides TFE3/TFEB translocations, TRCC shares alterations commonly present in other RCC histological subtypes, and these are associated with patient outcomes.
Mutations in c-Met, Fumarate Hydratase, and Folliculin Genes
Less than 5 % of RCC patients are afflicted with an inherited cancer syndrome. Age of onset in these patients is variable, although most tend to occur at an earlier age. Each of the inherited cancer syndromes predisposes patients to distinct subtypes of RCC [28]. Renal involvement can range from solitary lesions to bilateral and multifocal tumors. Patients may also have characteristic extrarenal manifestations. Family history, early onset, bilateral, and multifocal renal involvement should arouse suspicion for a hereditary renal cancer syndrome. Von Hippel–Lindau disease will be discussed later in this chapter. Three other entities will be mentioned briefly, including hereditary papillary renal cell carcinoma syndrome (HPRCC), hereditary leiomyomatosis/renal cell carcinoma (HLRCC), and Birt–Hogg–Dube syndrome (BHD), and will be covered more extensively in Chap. 29. Molecular assays, including DNA sequencing, are used to detect the germline mutations in these genes in suspected patients, as immunohistochemical assays are not useful in this setting.
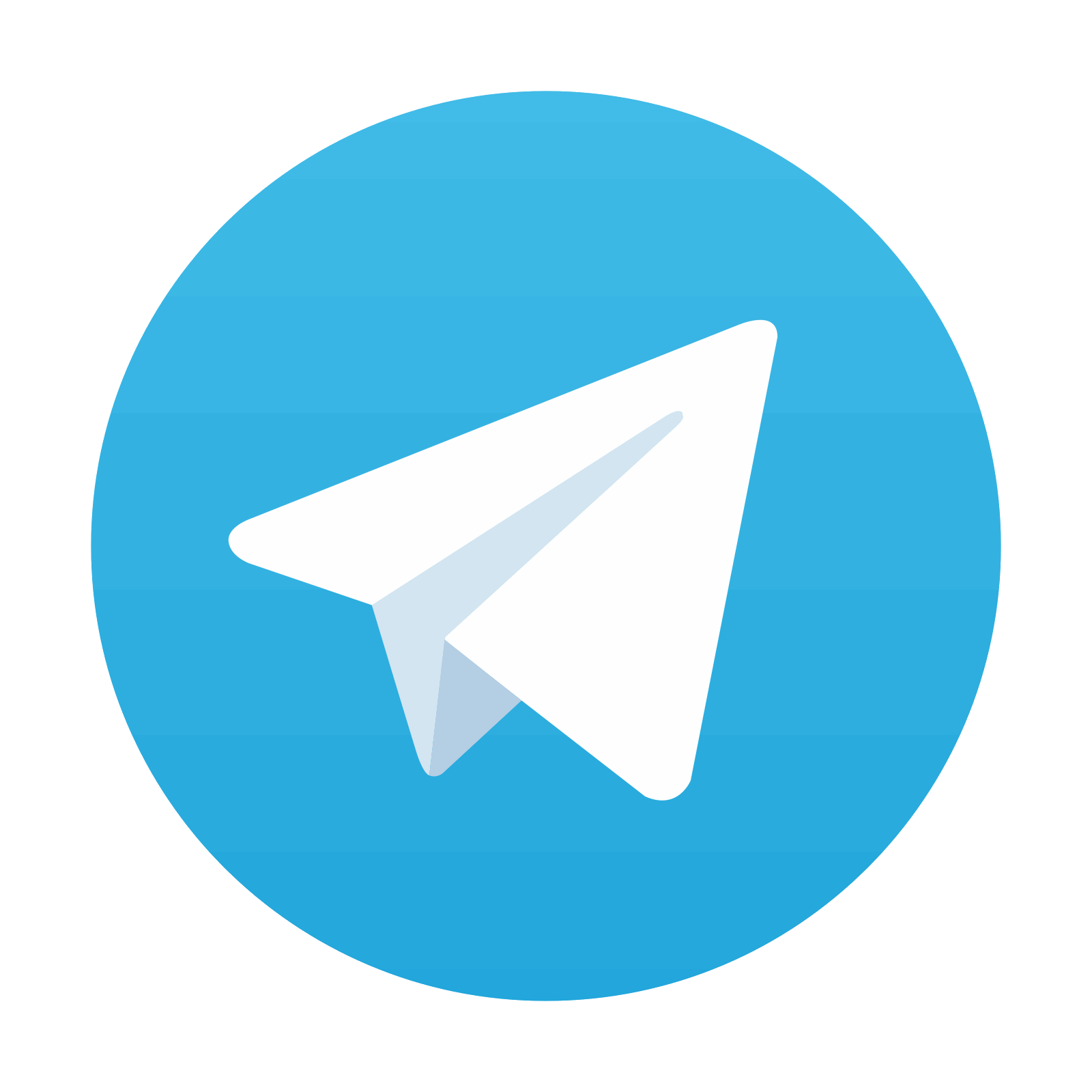
Stay updated, free articles. Join our Telegram channel

Full access? Get Clinical Tree
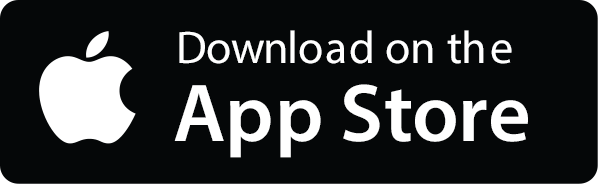
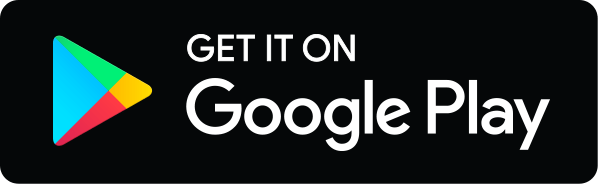