Disease
Gene therapy
Severe combined immunodeficiency (SCID)
Adenosine deaminase (ADA)
Cystic fibrosis
Cystic fibrosis transmembrane regulator (CFTR)
Sickle cell anemia
β-Globin
Hemophilia
Factor IX
Thalassemia
α or β–Globin
Peripheral artery disease
Vascular endothelial growth factor (VEGF)
Familial hyper cholesterolemia
Low-density lipoprotein (LDL) receptor
Lesch–Nyhan syndrome
Hypoxanthine-guanine phosphoribosyl transferase (HGPRT)
4.2 Approaches to Gene Therapy
The two main approaches to gene therapy are:
4.2.1 Somatic Gene Therapy
Somatic cells are the nonreproductive cells of an organism (other than sex cells), e.g., blood cells, bone marrow cells, intestinal cells etc. Somatic cell gene therapy involves the insertion of fully functional and expressible gene into a target somatic cell to correct genetic disease. In somatic gene therapy, the fully functional therapeutic gene is inserted into the target somatic cell of a patient to correct the genetic disease permanently. Somatic gene therapy will not be inherited by the patient’s offspring or later generations and any effects will be restricted to the individual patient only.
4.2.2 Germ Line Gene Therapy
The reproductive (sex) cells, i.e., sperm or eggs of an organism constitute germ cell line. Germ line gene therapy is the introduction of functional genes, which are integrated into genomes. Hence, the change due to germ line therapy is heritable and would be passed to later generations. This approach should be highly effective in treatment of genetic and hereditary disorders, however, for safety, ethical and technical reasons, this therapy is generally not attempted.
4.3 Principles of Gene Therapy
Gene therapy is introducing a functional copy of the gene for curing the defect. If a child or foetus is diagnosed to carry a defective gene leading to disability, one may like to correct it by one of the three methods: (1) by replacing the defective gene by correct gene, (2) by correcting the defective gene through gene targeting or (3) by gene augmentation either through increasing the number of copies of the gene or through a higher level of expression of introduced gene. Maximum progress in the area of gene therapy has been achieved through the gene augmentation method where normal foreign gene sequences for defective gene are introduced involving vector-mediated DNA delivery system.
The steps involved in the gene therapy are as follows:
1.
Identification and characterization of gene.
2.
Cloning of gene.
3.
Choice of vector.
4.
Method of delivery.
5.
Expression of gene.
The primary step is to identify the genetic defect and to clone a functional copy of the gene. The functional gene is then transferred to the patient, which involves choosing a vector with a suitable method of delivery. The vector/gene must be designed to allow proper expression of the gene inside the patient. There are two types of gene therapies:
4.3.1 Ex Vivo Gene Therapy
Ex vivo gene therapy involves the transfer of genes in cultured cells which are then reintroduced into the patient (therapy takes place outside the patient). This technique involves the following steps:
1.
Isolation of cells (selected tissues e.g., bone marrow) with genetic defect from a patient.
2.
Growing the cells in culture.
3.
Introduction of the therapeutic gene to correct the defective gene.
4.
Selection of the genetically corrected cells and their growth.
5.
Transplantation of the modified cells to the patient.
This therapy involves the use of patient’s own cells for culture and genetic correction and then transferred back to the patient. Hence, this therapy does not develop adverse immunological reactions and is quite effective (Fig. 4.1).
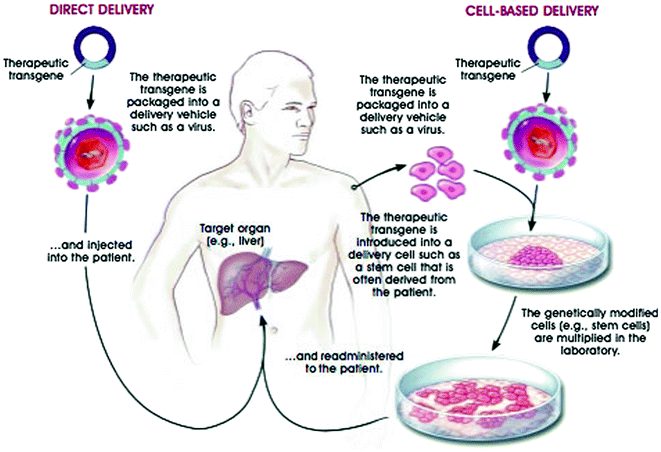
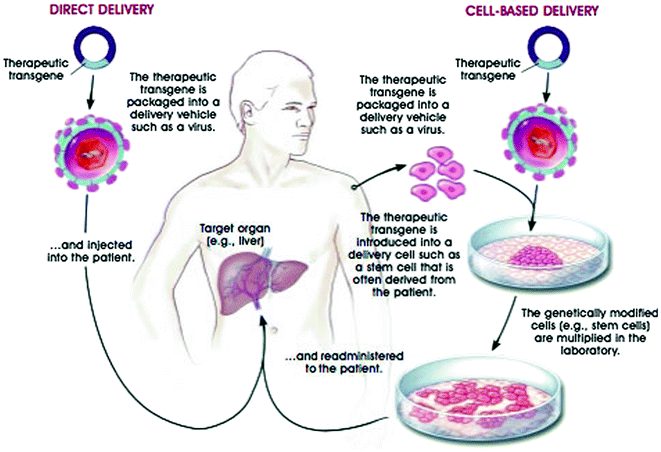
Fig. 4.1
Strategies for delivering therapeutic transgenes into patients; transfer of genes in cultured cells (ex vivo) and direct delivery of genes into the cells (in vivo) © 2001 Terese Winslow
4.3.2 In Vivo Gene Therapy
In vivo gene therapy is the direct delivery of the genes into the cells of the particular tissue.
4.4 Vehicles of Gene Transfer
A gene cannot be directly inserted into a person’s cells. It must be delivered to the cell using a carrier, or “vector” (Table 4.2). Gene transferring systems can be divided into two groups, viral and nonviral vectors. The examples of viral vectors are: (a) Retrovirus, (b) Adenovirus, (c) Adeno-associated virus (AAV), and the examples of nonviral vectors are: (a) Liposomes, (b) Ribozymes, and (c) Oligonucleotides.
Table 4.2
Gene transfer vehicles–vector delivery system include either recombinant viruses or various forms of plasmid DNA
Source | Vector | Genome | Advantages | Disadvantages |
---|---|---|---|---|
Viral | Retrovirus | ssRNA | Efficient transduction | Transduction requires cell division |
Stable gene transfer | Limits on transgene size/content | |||
Relatively safe | ||||
Adenovirus | dsDNA | Highly effective gene transfer | Transient transduction | |
Higher titer | Immunological response | |||
Adeno-associated virus (AAV) | ssDNA | Potential stable transduction | Status of genome not fully elucidated | |
Stable expression | ||||
Nonviral | Plasmid/Liposomes | dsDNA | Absence of viral components | Low transfer and expression |
Potential for targeting |
4.4.1 Viral Vectors in Gene Delivery
4.4.1.1 Recombinant Retrovirus Vectors
The most common class of gene transfer vector is the recombinant retroviruses. Retroviruses contain single-stranded RNA genome of 7–10 kb in size. These genomes are complexed with vector-encoded proteins and surrounded with a lipid bilayer generated when the virus buds from the infected cell. Retrovirus genomes integrate into the target cell chromosomes as double-stranded DNA provirus generated by reverse transcription. This aids the virus genomes to be stably transferred to the targeted cells. Examples of retroviruses used as recombinant vectors include: oncoretroviruses, such as Moloney Murine Leukemia virus (MuLV) associated with oncogenesis in mice; lentiviruses, such as human immunodeficiency virus (HIV) associated with AIDS in humans; and spumaviruses, such as the human foamy virus, that has no known pathogenesis in humans (Buchschacher 2001; Bushman 2007).
The basic retrovirus genome consists of three genes (gag, pol and env) enclosed between two long terminal repeats (LTRs) (Fig. 4.2 ). The LTR sequences are needed for integration of the DNA version of the virus genome into the host cell DNA. Between the upstream or 5′ LTR and the gag gene is the packaging signal (psi Ψ) which is essential for packaging the RNA into the budding virus particle, and the coding sequences for the reverse transcriptase and structural genes needed for the formation of mature virus particle.


Fig. 4.2
Retrovirus genome has packaging signal (Ψ) and gag, pol and env genes
The first step in generating replication-defective recombinant vectors is to introduce the coding sequences separately into cultured cells to generate packaging lines. The therapeutic gene is then combined with the remaining viral sequences, including the LTR and Ψ elements, in bacterial plasmids. This plasmid form of a vector is introduced into the packaging line, where the primary RNA transcripts are incorporated into the empty virus particles generated by the viral structural genes (Fig. 4.3). As a result, these virus particles contain the recombinant vector genomes, but not virus structural genes. After infection of the patient, the RNA inside the retroviral vector is reverse transcribed to give a DNA copy (although the retroviral vector does not carry a copy of the reverse transcriptase gene, a few molecules of reverse transcriptase enzyme are packaged in retrovirus particles). Ideally, the cloned gene, enclosed between the two LTR sequences, is then integrated into host cell DNA (Wolff and Budker 2005).
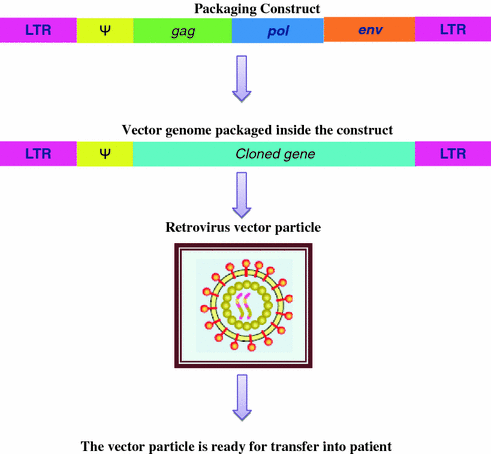
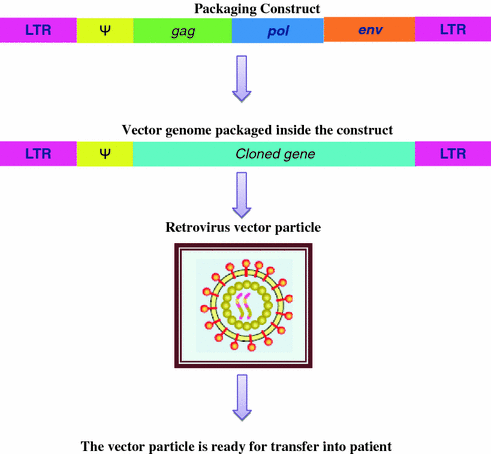
Fig. 4.3
Retrovirus vector system uses two virus constructs. The therapeutic vector carried the cloned gene and packaging signal. When both the constructs are present, the therapeutic vector and the cloned gene is packaged into the capsid (Mandel et al. 2006)
Recombinant retro viral vectors are advantageous because the integration of vectors into the target cell chromosomes is highly stable and do not cause an immune response. This class of vectors is also capable of highly efficient rates of gene transfer, depending on the choice of virus envelope. There are also several disadvantages for this class of recombinant virus vectors, which include a variable rate of gene expression. They can carry only small amounts of DNA (about 8 kb) and cannot infect nondividing cells. These vectors are difficult to deliver in vivo because they are relatively fragile. Besides, there are growing safety concerns about potential problems of mutagenesis (Weidhaas et al. 2000).
4.4.1.2 Recombinant Adenovirus Vectors
Adenoviruses were among the first viruses chosen as vectors for use in human gene therapy and currently the second most common form of gene transfer vectors. Adenoviruses are relatively large 36 kb linear double-stranded DNA viruses that contain many regulatory and structural genes that are expressed at different times during the infection (Fig. 4.4a). These genomes are surrounded by glycoprotein capsids that are generated through lytic life cycle. The virus particle consists of a simple icosahedral shell, or capsid, containing a single linear dsDNA molecule of approximately 36,000 base pairs. A terminal protein protects each end of the DNA. The capsid is made of 240 hexons, each of which is a trimer of the hexon protein. Hexons are named for their 6-fold symmetry and are surrounded by six neighboring hexons. The hexon protein has loops that project outward from the virus surface. Adenoviruses are subdivided into about 50 serotypes by their response to antibody binding. This variation is largely due to variation in the loops of the hexon protein. The adenoviruses share the same receptor as B-group coxsackieviruses. This protein is therefore known as coxsackievirus adenovirus receptor (or CAR), but its normal physiological role is unknown (Wu et al. 2006).
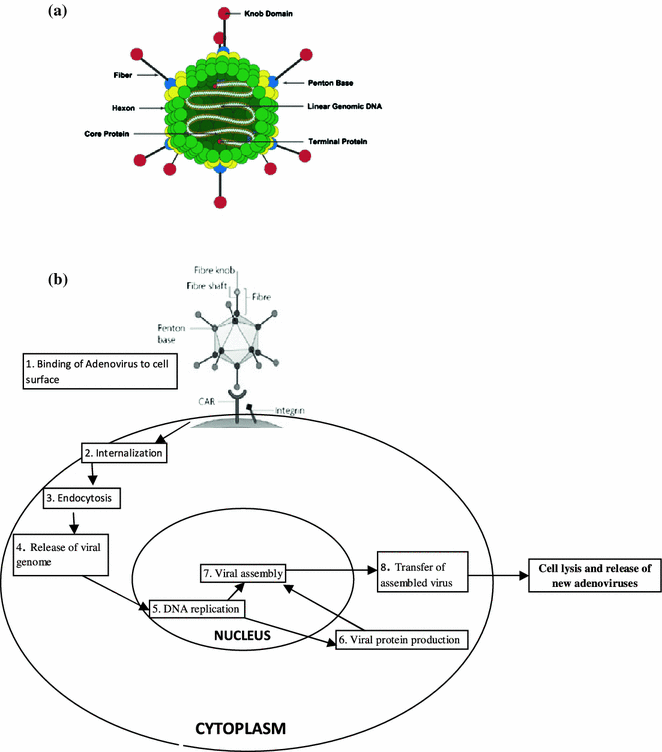
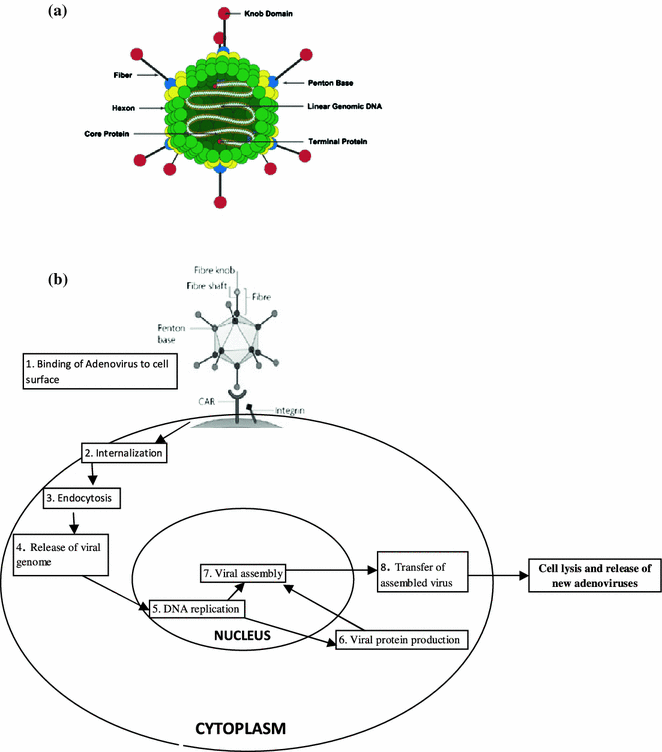
Fig. 4.4
a Adenovirus structure. b Adenovirus enters human cells by recognizing two receptors CAR and integrin. The virus is taken into the cell attached to the receptors and injects its DNA into the nucleus through a nuclear pore
Adenoviruses enter human cells by recognizing two receptors, CAR and integrin. The virus is taken into the cell attached to the receptors and surrounded by a membrane vesicle that dissociated in the cytoplasm. The adenovirus is injected into the nucleus through a nuclear pore. After the fiber tip binds to CAR, the penton base binds to integrins on the host cell surface (integrins are transmembrane proteins involved in adhesion) (Fig. 4.4b). The membrane puckers, move inward and form a vesicle that takes the adenovirus inside the cell. The virus is then released into the cytoplasm and travels toward the nucleus. The virion is disassembled outside the nucleus, and only the DNA (with its terminal proteins) enters the nucleus.
Adenoviruses have several advantages:
1.
They are relatively harmless and cause mild infections of epithelial cells.
2.
They are nononcogenic (i.e., they do not cause tumors).
3.
They are relatively easy to culture and can be produced in large quantities.
4.
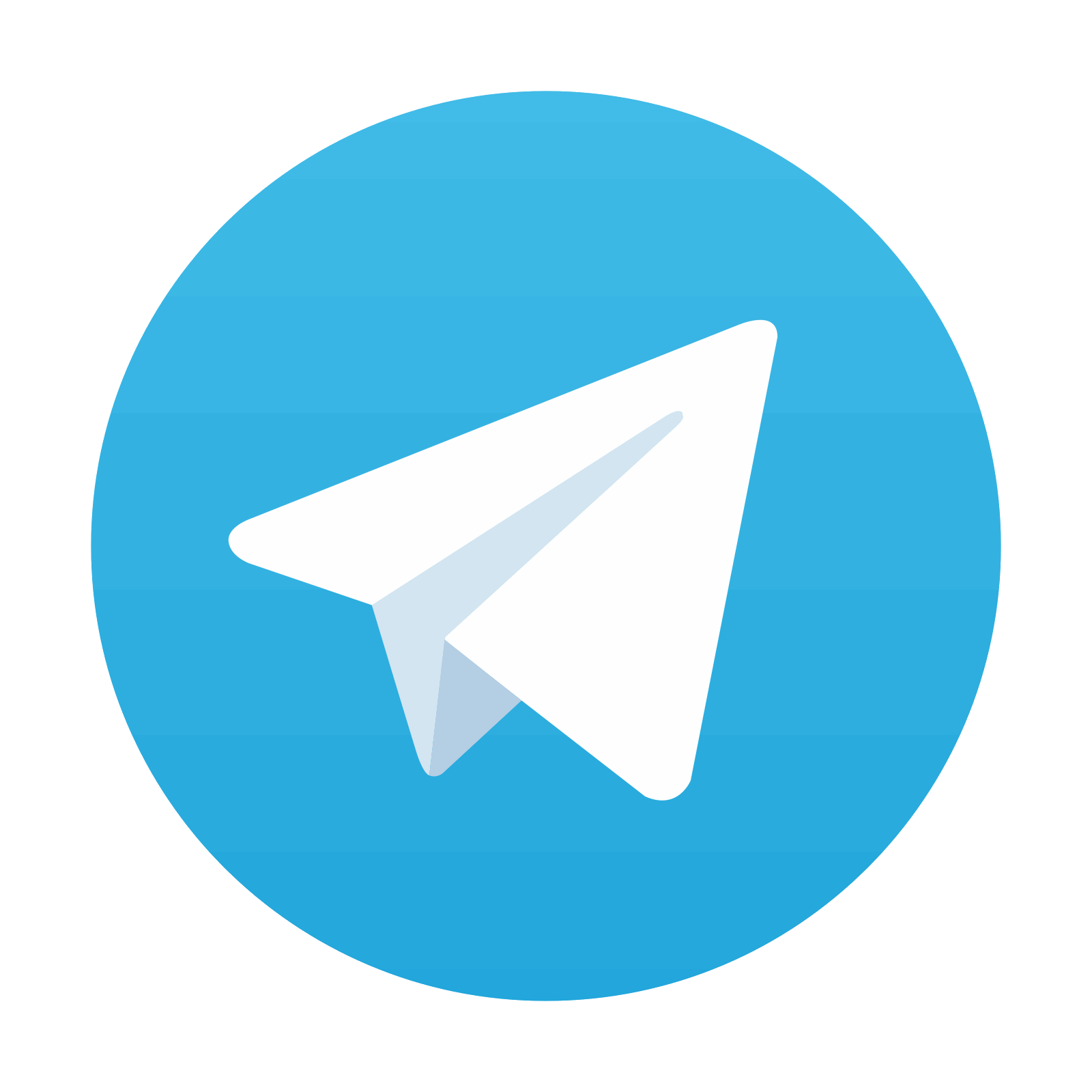
The life cycle and biology of adenovirus are well understood.
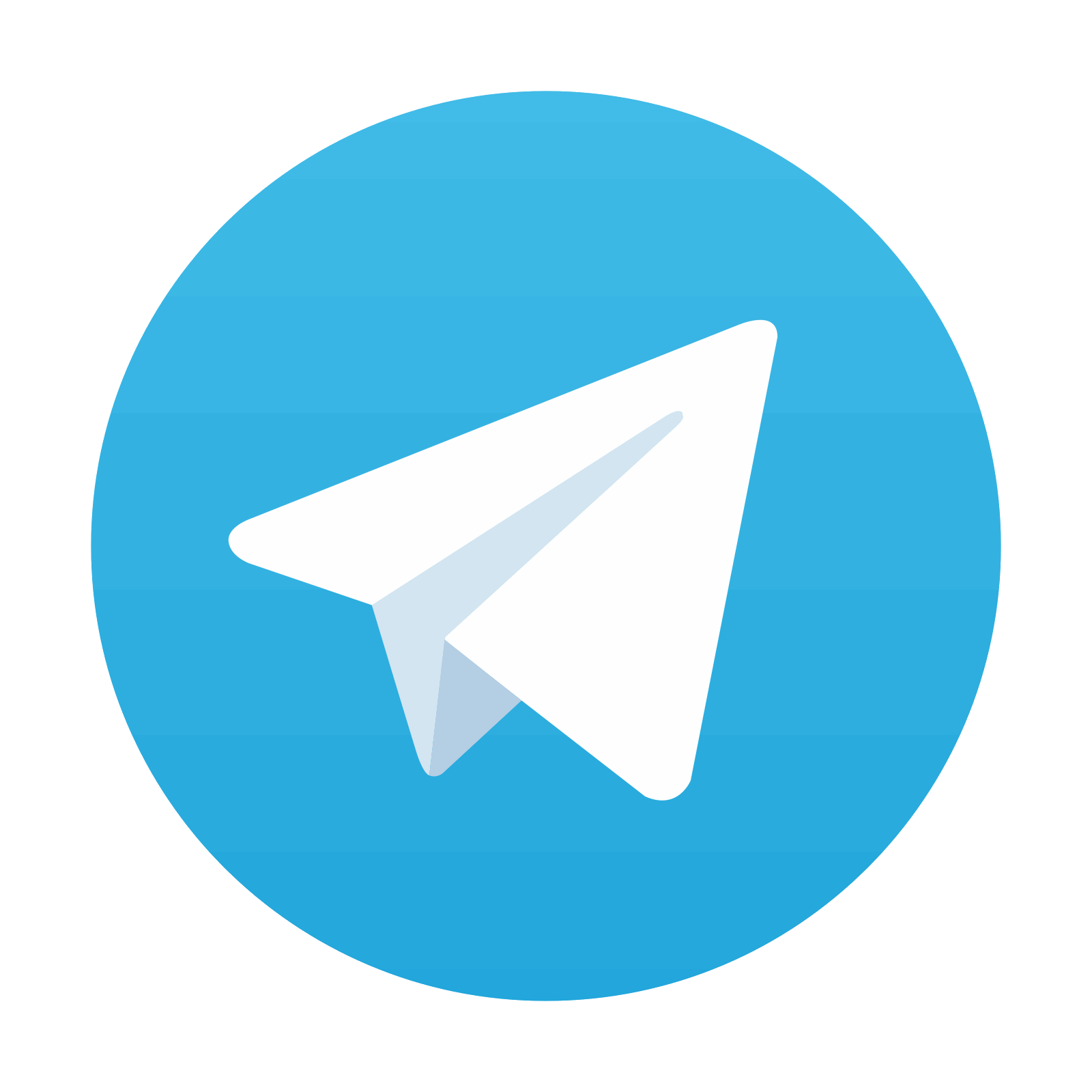
Stay updated, free articles. Join our Telegram channel

Full access? Get Clinical Tree
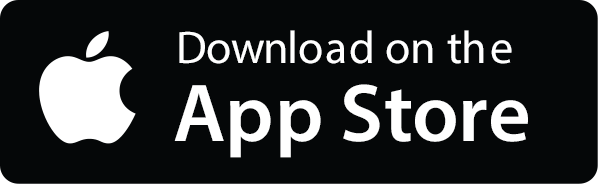
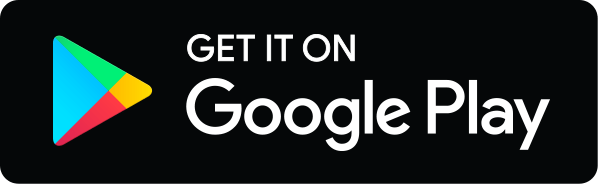
