CONTENTS
16.1.1 Recombinant DNA Technology
16.1.2 Plasmid (Expression Vector)
16.1.3 Polymerase Chain Reaction
16.2 Polymer-Based Delivery Systems
16.2.1 Reducible Polyethylenimine
16.2.2 Poly(Amido Amine) and Poly(Amido Ethylenimine)
16.2.3 Reducible Poly(Disulfide Amine)
16.2.4 Peptide-Based Gene Delivery Systems
16.2.5 Virus-Based Delivery Systems
16.3 Intracellular Organelle–Targeting Gene Delivery Systems
16.3.1 Nuclear-Targeted Gene Delivery Systems
16.3.2 Mitochondrial Targeting
16.4 Disease-Specific Expression Systems
Since it was first reported in 1972, genetic engineering has been rapidly progressing for treating many genetic and acquired diseases including cancer, diabetes, and tissue damage. Genetic engineering represented by recombinant DNA technology is applied to various fields including medicine, agriculture, and stockbreeding. Recently, recombinant DNA technology has been widely used for developing various protein drugs and for gene therapy. Nucleotides, including DNA, small interfering RNA (siRNA), and micro RNA, provide information needed by the cells and control the protein production. Protein not only works in the cell but also controls the organ and whole body functions. The absence or overexpression of specific proteins in the body can lead to various clinical symptoms due to their structural or functional changes. Gene therapy allows delivery of specific nucleotides to control the absence or overexpression of certain proteins responsible for diseases.
Protein drugs produced by recombinant DNA technology, such as granulocyte macrophage colony–stimulating factor (GM-CSF), erythropoietin (EPO), interleukins (ILs), and insulin-like growth factor-1 (IGF-1), represent one of the fastest-growing sectors in the pharmaceutical industry. Although protein drugs have exquisite therapeutic efficacy, their clinical applications are limited by poor oral bioavailability, low pharmacokinetic and pharmacodynamic profiles in the blood, high manufacturing cost, and difficult quality control of bioactivity. These require frequent parenteral administration, which, in turn, reduced patient compliance with increases in treatment cost. These limitations of treating diseases by protein drug can be overcome by gene therapy.
FIGURE 16.1 Scheme of gene delivery systems.
Gene therapy can be used to overexpress or reduce target proteins for treatment or prevention of diseases. DNA-based gene therapy delivers exogenous plasmid DNA, which encodes a specific gene to enhance the expression of therapeutic proteins, and thus, it must be delivered to the nucleus. On the other hand, siRNA reduces protein expression by silencing target mRNA in the cytoplasm (Figure 16.1). Successful clinical application of gene therapy delivering nucleotides to the right target requires overcoming several barriers, such as penetrating the cell membrane, stability in serum, and safety concerns such as uncontrolled gene delivery. DNA and RNA require appropriate delivery vehicles to overcome these barriers. The commonly used delivery vehicles include cationic polymers, peptides, liposomes, and nanoparticles.
16.1.1 RECOMBINANT DNA TECHNOLOGY
Recombinant DNA technology refers to modification, manipulation, and synthesis of DNA expression vectors. A DNA sequence extracted from cells or synthesized in the laboratory is inserted into DNA expression systems, such as plasmid and virus, using restriction enzymes. Preparation of the recombinant DNA expression vector needs to consider the following components (Figure 16.2):
• Template DNA: Target DNAs are prepared by extraction from cell or by artificial synthesis.
• Expression vector: A plasmid or virus is used as an expression vector.
• Polymerase chain reaction (PCR): The PCR is a biochemical technology that is used to amplify a single or a few copies of a piece of DNA. PCR is widely used for DNA cloning for sequencing, DNA-based phylogeny, the diagnosis of hereditary disease, and the detection and diagnosis of infectious disease.
FIGURE 16.2 Recombinant DNA technology.
• Restriction enzymes: Restriction enzymes (restriction endonuclease) cut DNA at or near specific recognition nucleotide sequences known as restriction sites.
16.1.2 PLASMID (EXPRESSION VECTOR)
Gene expression can be regulated in the cell at transcriptional, translational, and posttranslational levels, whereas expression vector can be regulated by transcription factors, promoters, and stimuli response. Plasmid vector expressing a target protein in the cell consists of the following components (Figure 16.3).
• Promoter: It is a region of DNA that initiates transcription of the target gene and located at the 5’ region of the antisense strand. The promoter is recognized by RNA polymerase and binds to transcription factors. Examples are cytomegalovirus, simian virus 40 (SV40), Moloney murine leukemia virus, and Rous sarcoma virus.
• 5′ Untranslated region: All mRNA have a cap structure located between the cap site and initiation codon. The cap structure consists of a 7-methylguanosine linked to the first nucleotide via 5′-5′ triphosphate bridge. It is known to influence mRNA translation efficiency.
• 3′-Untranslated region (3′-UTR): The 3′-UTR is located in mRNA following the termination codon. It has a potential role in mRNA stability and AU-rich motifs.
• Poly(A) tail: The poly(A) consists of adenosine residues and is located at the 3′ end of most eukaryotic mRNA.
• Multiple cloning site (MCS): An MCS is a short segment of DNA, which contains many restriction sites. Target DNA is inserted into the region of the MCS.
• Restriction site: The restriction site contains specific sequences of nucleotides, which are recognized by restriction enzymes.
FIGURE 16.3 Structure of plasmid vector.
To treat diseases, a gene delivery system must overexpress their therapeutic gene in their target cells. Various gene carriers have been engineered to be nontoxic and have a higher transfection efficiency, but some therapeutic genes have harmful side effects when expressed in nontarget or normal tissue (Figure 16.1). For example, a growth factor gene expressed in normal tissue cells may enhance tumor formation. Therefore, careful regulation of gene expression is required to avoid deleterious side effects.
16.1.3 POLYMERASE CHAIN REACTION
Since the development by Kary Mullis in 1983, PCR has become an essential tool in a variety of applications including DNA cloning, functional analysis of genes, diagnosis of hereditary disease, and identification of genetic fingerprints. Target DNAs between 0.1 and 10 kb pairs can be amplified by PCR. These components include the following:
• Template DNA: A DNA vector is contained in the therapeutic DNA region.
• Primers: Complementary primer to the 3′ end of each of the sense and antisense strand of the template DNA. It recognizes the template DNA and has a restriction enzyme site (20–30 mer).
• Taq polymerase: Taq polymerase recognizes and synthesizes new DNA segments complementary to the DNA template using deoxynucleotide triphosphate (dNTP) under high temperature around 75°C.
• dNTP: It supplies nucleotides for synthesis of DNA segments. It consists of adenine (A), guanine (G), thymine (T), and cytosine (C).
To prepare DNA segments, PCR typically undergoes 20–40 repeated temperature changes, called cycles. The cycle consists of three steps, denaturation, annealing, and extension (Figure 16.4).
• Denaturation step: Template DNA is denatured under 94°C–98°C for 20–30 seconds. Sense and antisense are divided due to disrupting the hydrogen bonding between complementary bases.
FIGURE 16.4 Polymerase chain reaction.
• Annealing step: This step progresses under 50°C–65°C for 20–40 seconds allowing annealing of the primers to the single-stranded DNA template.
• Extension step: It is called the elongation step under specific temperature depending on the DNA polymerase. Polymerase works under their optimum temperature at 75°C–80°C. The extension time depends on the DNA polymerase and on the length of the DNA fragment to be amplified.
PCR procedures have been developed for optimizing PCR conditions case by case, because PCR products are affected by various conditions, such as contamination of template DNA, size of template DNA, and primer design. Amplified DNA segments are purified and cloned into the expression vector using restriction enzymes. Constructs are verified by DNA sequence analysis.
Since its discovery, RNA interference (RNAi) has been widely used for functional analysis and for therapeutic purposes. Overexpression of specific proteins may induce diseases such as Parkinson’s disease (PD) and cancer. The siRNA recognizes target mRNA and leads its degradation via the intracellular mechanism of RNAi (Figure 16.1). This process is mediated by specific cytosolic proteins, dicer, RNA-induced silencing complex, and Argonaute. The siRNA molecule is localized in cytoplasm and, through complementary base pairing with the mRNA of the target gene, forms a double-stranded RNA molecule (dsRNA) molecule. The mediator proteins induce RNA silencing by cleaving the dsRNA molecule. For transportation of RNA molecules, a wide variety of nonviral vectors have been developed that induce internalization by endocytosis and subsequent escape from the endosome. These carriers are tightly bound to nucleic acids by electrostatic interactions, but this interaction may diminish the bioactivities of genes because not all genes may be released from the carrier to localize at their target locations. Various carriers showed effective endosomal escape and rapid dissociation in the cytoplasm by the proton-buffering effect and the cytoplasm-sensitive reducible polymers.
Several types of viruses such as retrovirus, adenovirus, adeno-associated virus, and gene poxvirus have been developed for gene delivery. A modified genome is included in the virion structure of viral vectors to deliver DNA. It consists of essential viral sequences and the required transcription unit of the exogenous gene. Although engineered viruses serve as efficient delivery systems for overcoming intracellular barriers, they are more immunogenic than synthetic carriers. Some viral types are difficult to apply as a gene delivery system because of their natural tropism. Recently, various delivery systems have been developed for reducing immunogenicity using capsid engineering or regulated expression cassettes. Viruses were modified for retargeting to new cell types by vector engineering approaches such as pseudotyping or genetically engineered with peptides.
16.2 POLYMER-BASED DELIVERY SYSTEMS
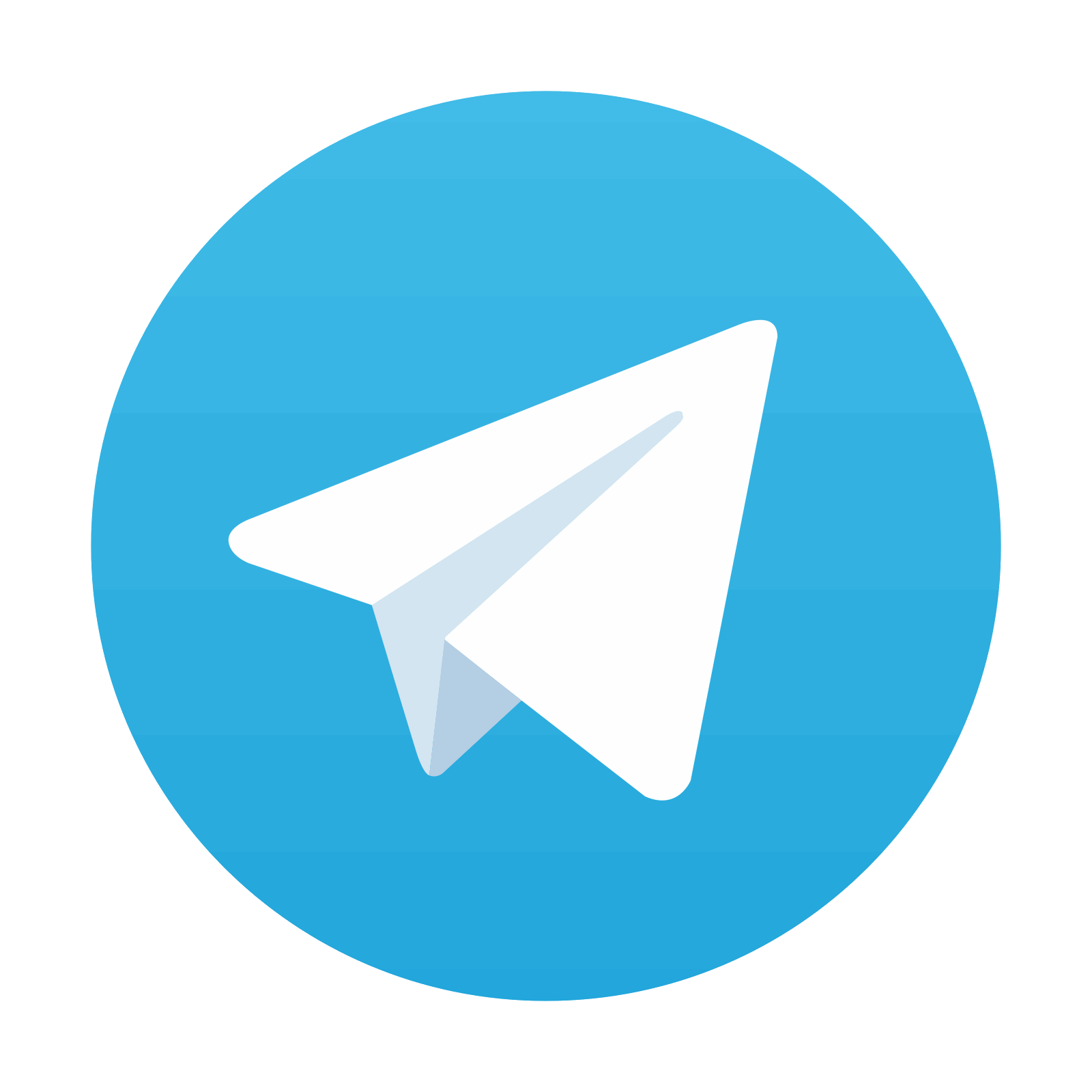
Stay updated, free articles. Join our Telegram channel

Full access? Get Clinical Tree
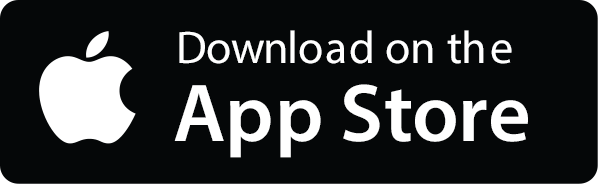
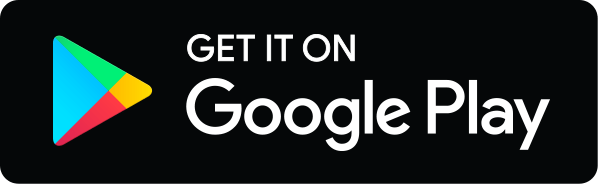