3.1 Introduction
Chapter 2 highlighted the different approaches and commercial devices used to deliver medicines to the respiratory tract. For the routine treatment of nonsevere asthma, the two most commonly used devices are pressurized metered-dose inhalers (pMDIs) and dry powder inhalers (DPIs). As discussed in Chapter 2, a wide range of devices are available on the market and research within this area spans over 50 years. The technology underpinning the formulation of these systems is thus extensive. This chapter gives an insight into the principles and research governing the formulation of pMDI and DPI systems.
3.2 Pressurized Metered-Dose Inhaler (pMDI) Formulation
The formulation of active pharmaceutical ingredients in pressurized liquids is relatively complex, since the propellants used have low dielectric constants and are relatively inert. Historically, chlorofluorocarbons (CFCs) were used, but due to their ozone-depleting effect [1] they were phased out following the 1989 Montreal Protocol, and a replacement was required.
Hydrofluoroalkanes (HFAs) were identified as a potential alternative, since they were liquid propellants and were considered inert with respect to the environment [2]. Furthermore, HFAs had no potential toxicological issues [3].
Two HFAs are utilized in the formulation of pMDIs: namely, HFA 227 and HFA 134a. The nomenclature for their numbering (XYZ) is as follows: X = carbon atom number − 1; Y = hydrogen atom number + 1; Z = number of fluoride atoms. A letter following this sequence is sometimes used to indicate isomerism (as in HFA 134a), with increasing suffix value indicating decreased symmetry [4]. The structures of HFA 227 and HFA 134a are shown in Figure 3.1, and some of their basic physical properties are given in Table 3.1.
Table 3.1 Some physical properties of HFA 227 and HFA 134a [4]
HFA 227 | HFA 134a | |
Chemical name | 1,1,1,2,3,3,3-heptafluoropropane | 1,1,1,2-tetrafluoroethane |
Chemical formula | CF3-CFH-CF3 | CF3CH2F |
MW | 170.03 | 102.03 |
Boiling point (°C) | −16.5 | −26.3 |
Vapor pressure (bar) | 3.90 | 5.72 |
Dielectric constant | 4.1 | 9.8 |
Liquid density (kg/m3) | 1408 | 1226 |
Solubility of water (g/kg 25 °C) | 0.61 | 2.20 |
Solubility of ethanol (25 °C) | Miscible | Miscible |
HFAs have low boiling points, high vapor pressures, and low dielectric constants. Although many of the physical properties of HFAs are similar to those of CFCs, direct translation of CFC formulations to HFA formulations was not possible. Historically, CFC formulations contained drug suspended in CFCs that were stabilized using surfactants. With the translation to HFA-based systems, it quickly became evident that the capacity for HFAs to solubilize these surfactants was not sufficient [5], and thus a stable flocculated system could not be formed. HFA pMDI systems thus had to be reformulated from scratch.
Formulations of HFA-based pMDI systems are generally categorized as either suspension or solution technologies. As the names suggest, suspension formulations contain a suspended drug formulation with or without a stabilizing agent, while solution-based formulations contain the drug solubilized in the HFA, with or without cosolvents, additives, and stabilizers. These approaches are discussed in more detail below.
3.2.1 Suspension Technology
To highlight the importance of suspension stability in pMDI systems, one must consider the metering system. Unlike conventional pharmaceutical suspensions, pMDI systems have to be metered in such a way as to maintain the integrity of the bulk formulation while exposing a small amount of pressurized liquid to standard pressure. Upon exposure, this small volume rapidly expands and an aerosol is generated (Figure 3.2).
The important thing to consider here is the metering chamber. During storage, and prior to actuation, the bulk formulation has continual access to the metering chamber. Furthermore, the chamber volume is small; typically around 50 μL. Thus, in order to ensure a consistent dose, the formulation needs to either (1) remain as a homogeneous suspension or (2) be readily resuspended, such that a representative sample of the bulk can be introduced into the metering chamber.
Another important issue is the materials used. Components—including spring, metering chamber, canister wall, and gaskets—need to have a relatively low adhesion/absorption potential with respect to the drug. Furthermore, pressure-sealing gaskets need to have low water permeability and minimal swelling with respect to the liquids used in the formulation [6, 7].
A review of any pharmaceutical textbook will provide a series of strategies for making a conventional suspension: utilizing the combination of Stokes’ law and electro-double-layer forces to create a stable, flocculated system (i.e. utilizing the Derjaguin and Landau, Verwey and Overbeek (DLVO) theory). Unfortunately, HFAs have a low dielectric constant (Table 3.1) and thus will exert only a weak double-layer force on suspended particles.
To overcome such issues, formulations must either alter the fundamental properties of the drug and media (for example, by altering propellant/drug density or by altering the particle size, to reduce sedimentation velocity) or modify the contact dynamics between the particulate systems. While the former approach is enticing, changing the particle size or density is fraught with difficulties, since there is potential for Oswald ripening (i.e. crystal growth within the formulation) when the size of particles is reduced or cosolvents are added to a formulation.
Changing the contact dynamics between particles appears more straightforward, as surfactants are routinely used in pharmaceutical formulations to alter the interfacial tension, form colloid bridges, or act as a steric shell. While surfactants such as oleic acid were historically used in CFC formulations, they are not soluble in HFAs and require cosolvents, which may influence the stability and solubility of the suspended drug [5]. Luckily, potential replacements exist that are soluble in HFAs; these include long-chain polymers such as polyethyleneglycol (PEG) or polyvinylpirrolidone (PVP), fluorinated carboxylic acids/ester surfactants, and hydrophilic surfactants.
These HFA-soluble surfactants have the ability to generate stable suspensions via reduced particle–particle and particle–pMDI component interactions. Figure 3.3a shows the influence of PEG concentration and molecular weight on the adhesion between drug particles in a model propellant, for example [8].
Figure 3.3 (a) Influence of polymer concentration and chain length on the adhesion of drug particles in an HFA-based system. Reproduced with permission from Traini D, Young PM, Rogueda P, Price R. Investigation into the influence of polymeric stabilizing excipients on inter-particulate forces in pressurised metered dose inhalers. Int J Pharm. 2006. (b) Adhesion of drug to different canister materials. Based on data from Traini D, Young PM, Rogueda P, Price R. Investigation into the influence of polymeric stabilizing excipients on inter-particulate forces in pressurised metered dose inhalers. Int J Pharm. 2006.
Image not available in this digital edition.
In addition to altering the “surface chemistry” of the drug particles via addition of surfactants, the surface energy of the pMDI canister components may be changed by material selection or coating technologies. This can be seen in Figure 3.3b when comparing the adhesion of drug to glass, aluminum, or polytetrafluoroethylene (PTFE). The careful selection of canister materials has already found use in commercial pMDI products and can most notably be observed in the Ventolin HFA product, which uses a fluorinated canister-coated pMDI with no additional surfactants [9].
3.2.2 Solution Technology
Solution-based pMDIs do not encounter the same issues associated with suspensions (i.e. sedimentation, caking, particle adhesion, Oswald ripening, etc.); however, they have their own unique issues, which need to be considered. Solution pMDIs are effectively dispersions in which individual drug molecules interact with the HFA/formulation at the molecular level. Such systems will thus have a higher potential for chemical degradation when compared to their suspension counterparts. In addition, drug molecules conventionally used in pMDIs are not readily soluble in HFAs and thus require a cosolvent. Finally, when solubilized, these systems require good physical stability and must not precipitate when exposed to variations in temperature.
As outlined in Table 3.1, ethanol is miscible in HFAs and is also a good solvent for many hydrophobic pharmaceutical drugs. Thus ethanol may be used as a cosolvent.
An example of an ethanol cosolvent/HFA formulation is 3Ms beclomethosone dipropionate formulation: Qvar. Interestingly, Qvar was reported as having improved respiratory deposition (50–60% in human studies) when compared to previous suspension pMDIs [10]. This is most likely due to the nature of solution formulations compared with suspensions. In suspensions, the particle size of the inhaled drug will be dependent on the size of the micronized material. However, in solution-based pMDIs, the inhaled particle size is dependent on the concentration of drug in the propellant and the nature of the droplet formulation during aerosolization (i.e. small droplets result in smaller particles). By controlling parameters such as orifice diameter and vapor pressure, the final particle size distribution may thus be controlled, along with the respiratory deposition profile (Figure 3.4). For example, by reducing the orifice size (the hole through which the aerosol droplets form when they exit the pMDI), a reduction in the droplet diameter will be observed. This results in a decrease in the final particle size and an increase in respirator deposition.
Figure 3.4 Influence of orifice diameter on the fine particle dose of beclomethasone diproprionate (BDP) from a solution pMDI. Reproduced with permission using data from Ganderton D, Lewis D, Davies R, Meakin B, Brambilla G, Church T. Modulite (R): a means of designing the aerosols generated by pressurized metered dose inhalers. Respiratory Medicine 2002;96:S3–S8
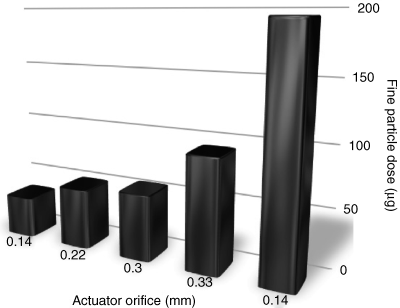
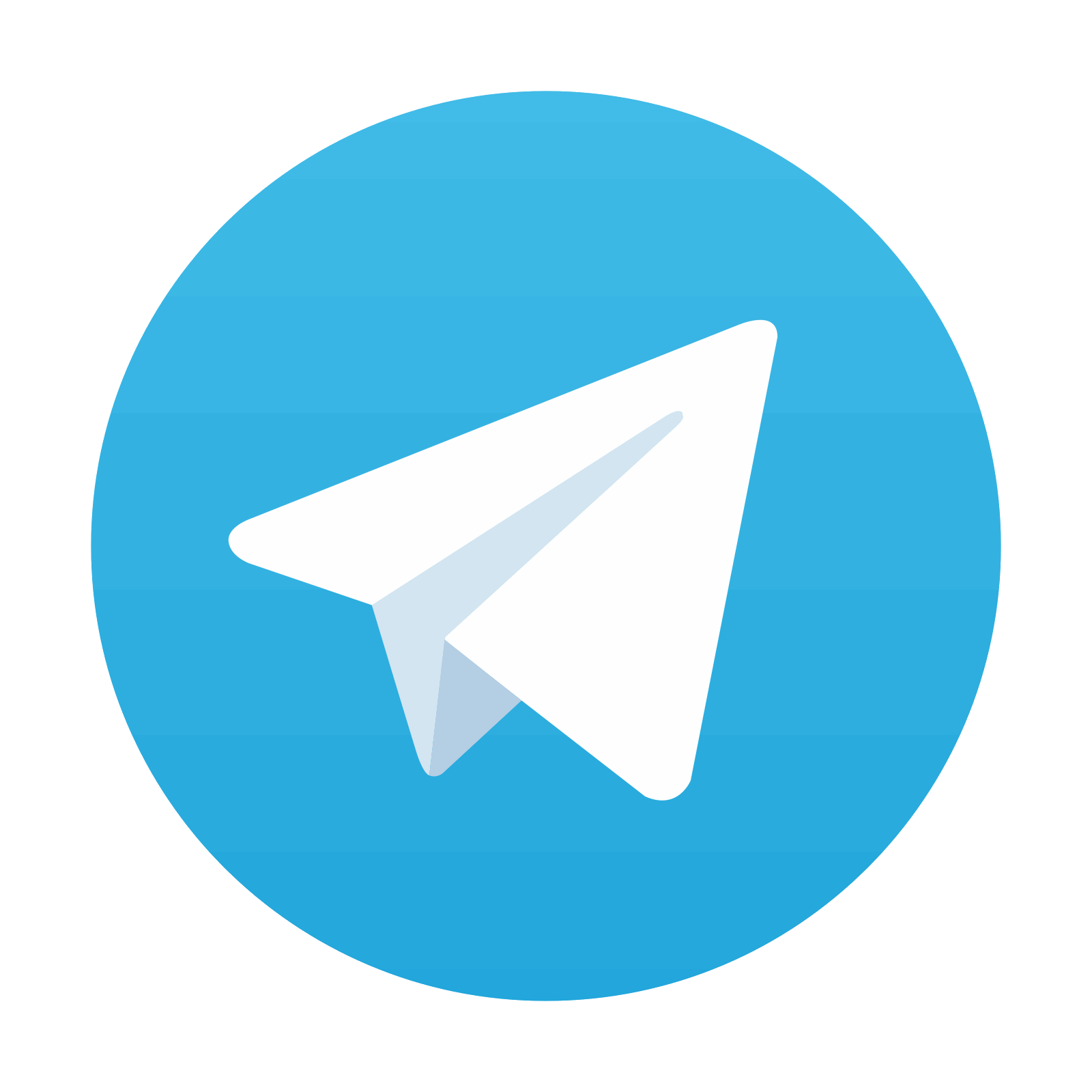
Stay updated, free articles. Join our Telegram channel

Full access? Get Clinical Tree
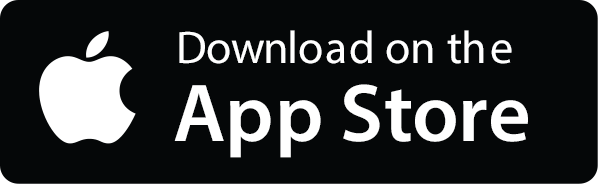
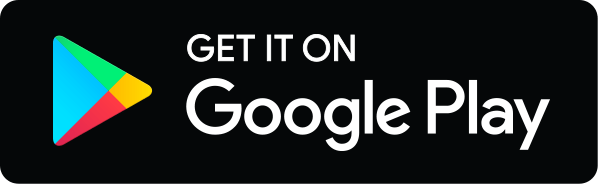