Fig. 3.1
Selection of an anatomically well-defined defect for study of induced skin regeneration. Top left: Incision through the entire dermis. Top right: The epidermis has been removed but the dermis remains intact. Bottom left: Partial-thickness skin excision. Bottom right: Full-thickness skin excision. Of the four configurations only the last is an appropriate defect
An example of a wound model of peripheral nerve regeneration that deviates from the requirements set above is the transection of only a fraction of the cross-section of a peripheral nerve (hemisection). This wound features the presence of residual nerve trunk at the site of partial transection (Fig. 3.2), while the remainder of the cross-section may be occupied by, for example, an experimental biomaterial. Such an experimental configuration appears unsuited for an accurate assay of the quality of whole nerve regeneration by electrophysiological methods, normally conducted by passing an electrical signal along the length of the nerve. Since the signal is expected to pass through both types of tissues, (residual and experimentally generated), the interpretation, either of electrophysiological or of histological data, becomes difficult, requiring use of specific labels or use of mutant animals. These considerations do not rule out use of the hemisection model for studies of nerve regeneration; however, they indicate the additional degree of experimental difficulty that is introduced by this choice of injury. In contrast, the completely transected nerve conducts no electrophysiological signal unless a suitable regenerate is present and can also be analyzed histologically with great confidence by labeling the transection site with each stump using, for example, suture marks or other convenient location marker.

Fig. 3.2
Selection of an anatomically well-defined defect for study of induced peripheral nerve regeneration. Top: Hemisection. Middle: Complete transection. Bottom: Stumps have been inserted into tube (tubulation). The transected nerve is an anatomically well-defined defect. A tube serves as a device for containment of exudate but frequently contributes strongly to regeneration across the gap
It has been pointed out (Swamydas et al. 2004) that partial peripheral nerve injuries, in contrast to complete nerve transection, occur more commonly in clinical practice and frequently result in the development of chronic neuropathic pain. Accordingly, these authors studied a peripheral nerve hemisection model in order to detect changes in concentration levels of pain-related peptides (Swamydas et al. 2004). In another example, important information about axonal regeneration after trauma in the central nervous system has been obtained by use of animal models that differ from the description of an anatomically well-defined defect presented here. In one of these studies of the central nervous system, information on axonal growth has been obtained using an animal model injured by midthoracic dorsal spinal cord hemisection (Duffy et al. 2009; Deng et al. 2013). Hemisection models of nerve injury appear to provide an experimental volume that allows studies of wound healing or of axonal regeneration rather than studies of whole organ regeneration.
Another major requirement for a standardized experimental defect is inclusion of the tissue exudate that forms spontaneously inside the wound. The initial events in the inflammatory response following injury are flow of blood and extravascular tissue fluid inside the wound, together with migration of cells from adjoining tissues. This fluid is often collectively referred to as “wound fluid”; it will be referred to here as “exudate”. The exudate that fills deep wounds, both in skin and nerve, contains a large number of soluble regulators of cell function that orchestrate the inflammatory response. Substantial information is known about the composition of exudate in skin wounds, showing that this fluid is endowed richly with soluble regulators (Regan and Barbul 1991; Breuing et al. 1992; Kroeze et al. 2012). An analysis of required reactants for inducing regeneration in skin and peripheral nerves, described in detail in Chap. 7, suggests strongly that the exudate provides indispensable endogenous reactants that contribute to induction of regeneration.
Uncontrolled escape of exudate from a defect, or even a significant alteration in concentration of its soluble regulators, has significantly modified the outcome both of a spontaneous and an induced healing process. For example, the rate of epithelialization in dehydrated skin wounds, in which the soluble regulators were presumably unable to exist in a sufficiently diffusible state due to lack of aqueous solvent, was significantly inhibited and the time for wound closure was increased compared with wounds that were maintained simply moist by use of an occlusive dressing (Winter 1972). The importance of a moderate amount of moisture in skin wound healing has been confirmed in later studies while dehydrated wounds or wounds with excessive fluid have been considered much less favorable for optimal healing (Bishop et al. 2003; Lachenbruch and VanGilder 2012).
A little more is known about the role of wound exudate in peripheral nerve regeneration . Following transection of the sciatic nerve in the rat, a cylindrical tissue about 1 mm in diameter, fluid exudate leaves the stumps at a rate estimated at about 1 ml/h (Longo et al. 1983a, b; Williams and Varon 1985). The exudate comprises primarily of plasma that has leaked out of blood vessels, as a result of the increase in vascular permeability associated with trauma, as well as resulting from components synthesized by the injured neuron (Fu and Gordon 1997). Axotomized neurons synthesize cytokines, including platelet-derived growth factor (PDGF) and acidic fibroblast growth factor (FGF1), which contribute to the inflammatory response of the transected nerve. These cytokines synthesize neurotrophic factors, including nerve growth factor (NGF; Lundborg et al. 1982b; Longo et al. 1983a, b; Fu and Gordon 1997). These cytokines also upregulate migration of nonneuronal cells and enhance angiogenesis (Fu and Gordon 1997). In an example from peripheral nerve wound healing, a transected nerve that was allowed to lose a significant mass of exudate before the two stumps were sutured directly together had a markedly diminished expectation for regeneration (de Medinacelli et al. 1983; Terzis 1987). In another study, the activity of exudate was shown by injection of exudate from the transected sciatic nerve into the vitreous of the eye, a process that induced significantly enhanced formation of axon-like processes (Cho and Chung 1996).
In studies of spontaneous healing in the adult, that is, in the absence of exogenous reactants, the contents of the wound are simply allowed to contract the wound and synthesize scar. When the object of the study is to attempt modification of the healing process, various experimental exogenous reactants (referred to simply as reactants below) are supplied to the defect. One or more of these reactants, together with the exudate (endogenous reactant), may be regeneratively active and may suffice to modify the healing process by inducing synthesis of scarless tissues.
Clearly, much more information is needed before the detailed role of exudate in an experimental wound is fully understood. We will regard the wound exudate as one of the critical reactants in a study of induced regeneration and will advocate its required inclusion in the experimental volume.
3.4 Generation of the Experimental Volume in an Animal Model
Using an analogy with synthetic chemistry, we will refer to the experimental volume as a laboratory reactor, maintained under carefully controlled conditions. The reactor must have sufficient volume to accommodate occasionally bulky reactants as well as a substantial mass of newly synthesized product. The reactants may include any combination of cell cultures, cytokine solutions, matrix components, or a scaffold. The product will eventually be separated from residual reactants as well as from the tissues that comprise the boundaries of the reactor itself and will be identified by suitable analytical techniques described in the next chapter.
Energy Source Used to Generate Injury
The most direct surgical procedure that can be used to generate experimental space by inflicting an injury is the controlled deletion of tissues by a surgical instrument, such as a scalpel. Injuries such as those inflicted by freezing tissue, by abrading skin superficially, and even by simply incising skin, or by crushing nerve, do not delete tissue mass and cannot generate the desired volume. Excision of skin is typically carried out by mechanical instruments, such as a scalpel, fine scissors, or a dermatome. There is also a need to consider methods of tissue deletion using other forms of energy, such as a laser.
A direct comparison was made between three methods for producing a skin defect to a depth of equal thickness for each treatment, namely, excision with a dermatome, use of a laser to inflict burn injuries, and use of a heated brass template to burn swine skin by direct contact. The results showed unambiguously that generation of partial-thickness skin wounds by use of controlled burns significantly delayed the reepithelialization process compared to wounds produced by surgical excision (Schaffer et al. 1997). The delay in healing wounds of equal depth produced by burns was interpreted as a direct result of thermal tissue destruction extending considerably beyond the boundaries of the nominal defects (Schaffer et al. 1997). In another study, a delay in reepithelialization was observed in wounds produced by laser ablation relative to wounds produced by dermatome (Green et al. 1992). Further differences between laser resurfacing and ablative methods in extent of wound contraction were demonstrated in wounds of the same total depth of injury (Ross et al. 2000). In another study the extent of metal metalloproteinase expression was higher on days 1–5 post injury in full thickness laser-created wounds, indicating differences in the level of the inflammatory response, than in comparable same day excisional wounds (Draper et al. 2002). Each of these studies showed that the healing response in skin wounds depended to a significant extent on the method of generating the injury.
Examples of different outcomes resulting from various peripheral nerve surgery protocols also emphasize the importance of the energy source for generating the wound. In a comparative study of neuroma formation in the rat sciatic nerve after neurectomy by laser and by scalpel it was observed that neuromas were formed in both types of injuries; however, neuromas produced by laser showed a foreign body reaction with multinucleated giant cells surrounding carbonaceous debris that was not present in scalpel neuromas (Fischer et al. 1983). Another study (Shapiro et al. 1989) focused on differences between scalpel transections and laser transections in outcomes of typical neuromas following transection of the rat sciatic nerve. Although there was no difference in the size of neuromas produced by either method of transection, laser transections produced neuromas characterized by multinucleated giant cells and carbonaceous debris in apparent confirmation of the differences observed earlier (Fischer et al. 1983).
The available evidence from comparative studies suggests strongly that generation of a defect either in skin or in peripheral nerves by laser treatment, at least as currently practiced, introduces important differences in injury response compared to changes produced following excision by scalpel. Since, the overwhelming method for injury generation described in the literature is by excision, it would make sense to regard use of a laser as an interesting experimental variant and to consider excision as the standard method for defect generation. For this reason, the discussion below is limited to defects in skin and peripheral nerves produced by surgical excision.
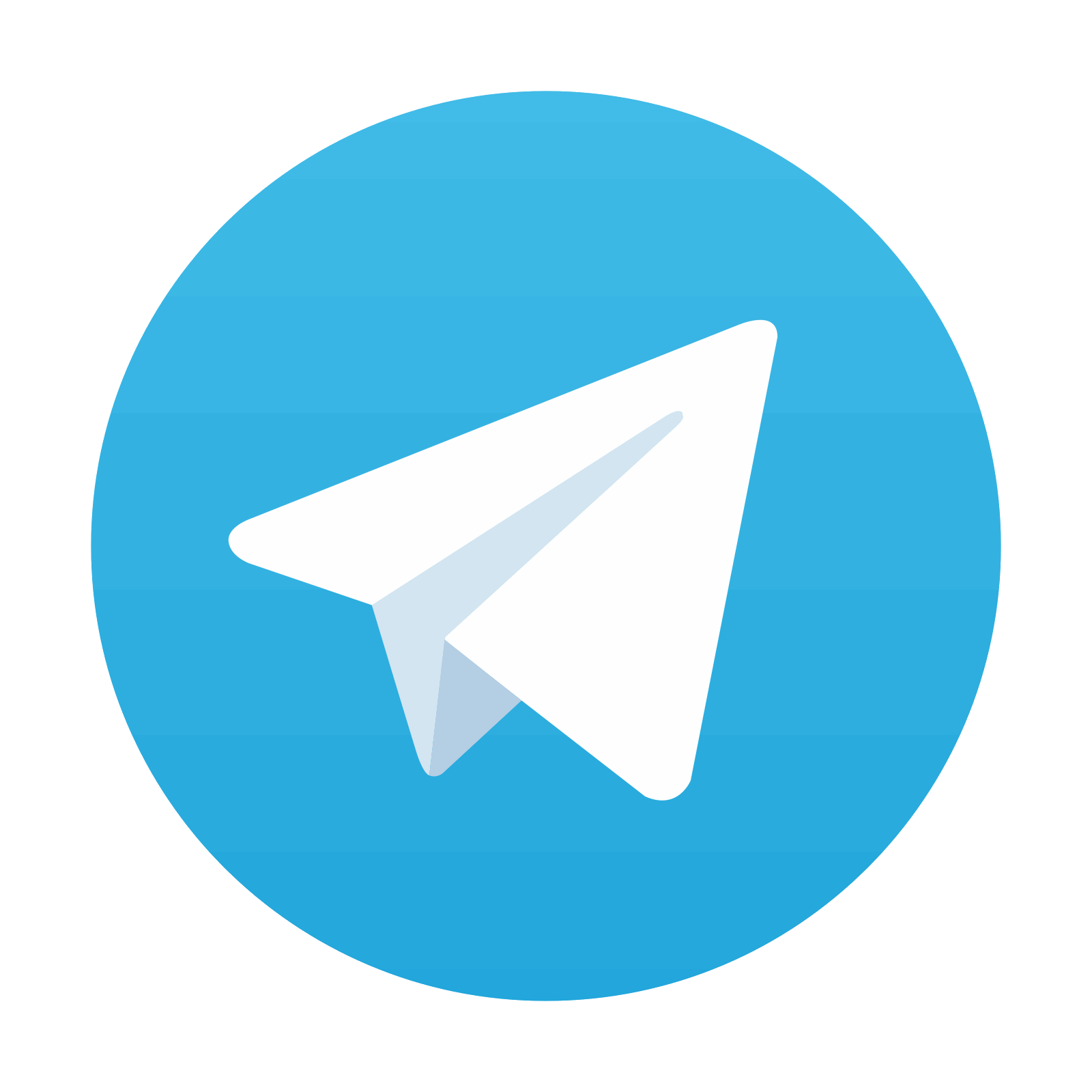
Stay updated, free articles. Join our Telegram channel

Full access? Get Clinical Tree
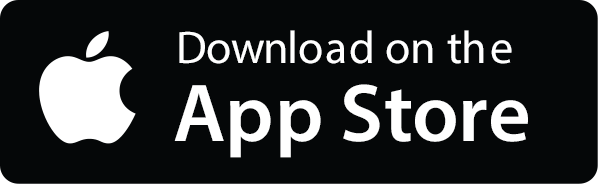
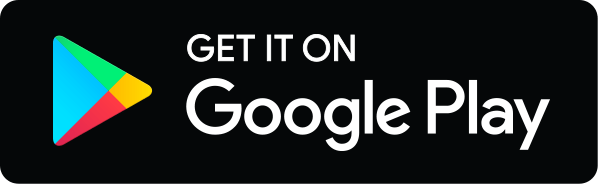