7Enzymes: Mechanism of Action
Peter J. Kennelly, PhD & Victor W. Rodwell, PhD
OBJECTIVES
After studying this chapter, you should be able to:
Illustrate the structural relationships between B vitamins and coenzymes.
Outline the four principal mechanisms by which enzymes achieve catalysis.
Describe how an “induced fit” facilitates substrate recognition and catalysis.
Outline the underlying principles of enzyme-linked immunoassays. Explain how coupling an enzyme to an NAD(P)+-dependent dehydrogenase can simplify assay of its activity.
Identify enzymes and proteins whose plasma levels are used for the diagnosis and prognosis of a myocardial infarction.
Describe the application of restriction endonucleases and of restriction fragment length polymorphisms in the detection of genetic diseases.
Explain the utility of site-directed mutagenesis for the identification of residues involved in catalysis, in the recognition of substrates or allosteric effectors, or in the mechanism of enzyme action.
Describe how the addition of fused affinity “tags” via recombinant DNA technology can facilitate purification of a protein expressed from its cloned gene.
Indicate the function of specific proteases in the purification of affinity-tagged enzymes.
Discuss the events that led to the discovery that RNAs can act as enzymes.
BIOMEDICAL IMPORTANCE
Enzymes are biologic polymers that catalyze the chemical reactions that make life, as we know it, possible. The presence and maintenance of a complete and balanced set of enzymes is essential for the breakdown of nutrients to supply energy and chemical building blocks; the assembly of those building blocks into proteins, DNA, membranes, cells, and tissues; and the harnessing of energy to power cell motility, neural function, and muscle contraction. The vast majority of enzymes are proteins. Notable exceptions include ribosomal RNAs and a handful of self-cleaving or self-splicing RNA molecules known collectively as ribozymes. The ability to assay the activity of specific enzymes in blood, other tissue fluids, or cell extracts aids in the diagnosis and prognosis of disease. Deficiencies in the quantity or catalytic activity of key enzymes can result from genetic defects, nutritional deficits, or toxins.
Defective enzymes can result from genetic mutations or infection by viral or bacterial pathogens (eg, Vibrio cholerae). Medical scientists address imbalances in enzyme activity by using pharmacologic agents to inhibit specific enzymes and are investigating gene therapy as a means to remedy deficits in enzyme level or function.
In addition to serving as the catalysts for all metabolic processes, their impressive catalytic activity, substrate specificity, and stereospecificity enable enzymes to fulfill key roles in other processes related to human health and well-being. The absolute stereospecificity of enzymes is of a particular value for use as soluble or immobilized catalysts for specific reactions in the synthesis of a drug or antibiotic. Proteases and amylases augment the capacity of detergents to remove dirt and stains. Enzymes play an important role in producing or enhancing the nutrient value of food products for both humans and animals. The protease rennin, for example, is utilized in the production of cheeses while lactase is employed to remove lactose from milk for the benefit of lactose-intolerant persons deficient in this hydrolytic enzyme (Chapter 43).
ENZYMES ARE EFFECTIVE & HIGHLY SPECIFIC CATALYSTS
The enzymes that catalyze the conversion of one or more compounds (substrates) into one or more different compounds (products) enhance the rates of the corresponding non-catalyzed reaction by factors of at least 106. Like all catalysts, enzymes are neither consumed nor permanently altered as a consequence of their participation in a reaction.
In addition to being highly efficient, enzymes are also extremely selective catalysts. Unlike most catalysts used in synthetic chemistry, enzymes are specific both for the type of reaction catalyzed and for a single substrate or a small set of closely related substrates. Enzymes are also stereospecific catalysts and typically catalyze reactions of only one stereoisomer of a given compound—for example, D- but not L-sugars, L- but not D-amino acids. Since they bind substrates through at least “three points of attachment,” enzymes can even convert non-chiral substrates to chiral products. Figure 7–1 illustrates why the enzyme-catalyzed reduction of the nonchiral substrate pyruvate produces only L-lactate, not a racemic mixture of D- and L-lactate. The exquisite specificity of enzyme catalysts imbues living cells with the ability to simultaneously conduct and independently control a broad spectrum of chemical processes.
FIGURE 7–1 Planar representation of the “three-point attachment” of a substrate to the active site of an enzyme. Although atoms 1 and 4 are identical, once atoms 2 and 3 are bound to their complementary sites on the enzyme, only atom 1 can bind. Once bound to an enzyme, apparently identical atoms thus may be distinguishable, permitting a stereospecific chemical change.
ENZYMES ARE CLASSIFIED BY REACTION TYPE
The commonly used names for most enzymes describe the type of reaction catalyzed, followed by the suffix -ase. For example, dehydrogenases remove hydrogen atoms, proteases hydrolyze proteins, and isomerases catalyze rearrangements in configuration. Modifiers may precede the name to indicate the substrate (xanthine oxidase), the source of the enzyme (pancreatic ribonuclease), its regulation (hormone-sensitive lipase), or a feature of its mechanism of action (cysteine protease). Where needed, alphanumeric designators are added to identify multiple forms of an enzyme (eg, RNA polymerase III; protein kinase Cβ).
To address ambiguities, the International Union of Biochemists (IUB) developed an unambiguous system of enzyme nomenclature in which each enzyme has a unique name and code number that identify the type of reaction catalyzed and the substrates involved. Enzymes are grouped into the following six classes.
1. Oxidoreductases—enzymes that catalyze oxidations and reductions.
2. Transferases— enzymes that catalyze transfer of moieties such as glycosyl, methyl, or phosphoryl groups.
3. Hydrolases— enzymes that catalyze hydrolytic cleavage of C—C, C—O, C—N and other covalent bonds.
4. Lyases— enzymes that catalyze cleavage of C—C, C—O, C—N and other covalent bonds by atom elimination, generating double bonds.
5. Isomerases—enzymes that catalyze geometric or structural changes within a molecule.
6. Ligases—enzymes that catalyze the joining together (ligation) of two molecules in reactions coupled to the hydrolysis of ATP.
Despite the clarity of the IUB system, the names are lengthy and relatively cumbersome, so we generally continue to refer to enzymes by their traditional, albeit sometimes ambiguous names. The IUB name for hexokinase illustrates both the clarity of the IUB system and its complexities. The IUB name of hexokinase is ATP:D-hexose 6-phosphotransferase E.C. 2.7.1.1. This name identifies hexokinase as a member of class 2 (transferases), subclass 7 (transfer of a phosphoryl group), subsubclass 1 (alcohol is the phosphoryl acceptor), and “hexose-6” indicates that the alcohol phosphorylated is on carbon six of a hexose. However, we continue to call it hexokinase.
PROSTHETIC GROUPS, COFACTORS, & COENZYMES PLAY IMPORTANT ROLES IN CATALYSIS
Many enzymes contain small nonprotein molecules and metal ions that participate directly in substrate binding or in catalysis. Termed prosthetic groups, cofactors, and coenzymes, these extend the repertoire of catalytic capabilities beyond those afforded by the limited number of functional groups present on the aminoacyl side chains of peptides.
Prosthetic Groups Are Tightly Integrated Into an Enzyme’s Structure
Prosthetic groups are tightly and stably incorporated into a protein’s structure by covalent or noncovalent forces. Examples include pyridoxal phosphate, flavin mononucleotide (FMN), flavin adenine dinucleotide (FAD), thiamin pyrophosphate, biotin, and the metal ions of Co, Cu, Mg, Mn, and Zn. Metals are the most common prosthetic groups. The roughly one-third of all enzymes that contain tightly bound metal ions are termed metalloenzymes. Metal ions that participate in redox reactions generally are complexed to prosthetic groups such as heme (Chapter 6) or iron–sulfur clusters (Chapter 12). Metals also may facilitate the binding and orientation of substrates, the formation of covalent bonds with reaction intermediates (Co2+ in coenzyme B12), or by acting as Lewis acids or bases to render substrates more electrophilic (electron-poor) or nucleophilic (electron-rich), and hence more reactive.
Cofactors Associate Reversibly With Enzymes or Substrates
Cofactors serve functions similar to those of prosthetic groups, but bind in a transient, dissociable manner either to the enzyme or to a substrate such as ATP. Unlike the stably associated prosthetic groups, cofactors must be present in the medium surrounding the enzyme for catalysis to occur. The most common cofactors also are metal ions. Enzymes that require a metal ion cofactor are termed metal-activated enzymes to distinguish them from the metalloenzymes for which metal ions serve as prosthetic groups.
Coenzymes Serve as Substrate Shuttles
Coenzymes serve as recyclable shuttles—or group transfer agents—that transport many substrates from one point within the cell to another. The function of these shuttles is twofold. First, they stabilize species such as hydrogen atoms (FADH) or hydride ions (NADH) that are too reactive to persist for any significant time period in the presence of the water or organic molecules that permeate the cell interior. They also serve as an adaptor or handle that facilitates the recognition and binding of small chemical groups, such as acetate (coenzyme A), by their target enzymes. Other chemical moieties transported by coenzymes include methyl groups (folates) and oligosaccharides (dolichol).
Many Coenzymes, Cofactors & Prosthetic Groups Are Derivatives of B Vitamins
The water-soluble B vitamins supply important components of numerous coenzymes. Several coenzymes contain, in addition, the adenine, ribose, and phosphoryl moieties of AMP or ADP (Figure 7–2). Nicotinamide is a component of the redox coenzymes NAD and NADP, whereas riboflavin is a component of the redox coenzymes FMN and FAD. Pantothenic acid is a component of the acyl group carrier coenzyme A. As its pyrophosphate, thiamin participates in decarboxylation of α-keto acids, and the folic acid and cobamide coenzymes function in one-carbon metabolism.
FIGURE 7–2 Structure of NAD+ and NADP+. For NAD+, . For NADP+,
CATALYSIS OCCURS AT THE ACTIVE SITE
An important early 20th-century insight into enzymic catalysis sprang from the observation that the presence of substrates renders enzymes more resistant to the denaturing effects of elevated temperatures. This observation led Emil Fischer to propose that enzymes and their substrates interact to form an enzyme–substrate (ES) complex whose thermal stability was greater than that of the enzyme itself. This insight profoundly shaped our understanding of both the chemical nature and kinetic behavior (Chapter 8) of enzymic catalysis.
Fischer reasoned that the exquisitely high specificity with which enzymes discriminate their substrates when forming an ES complex was analogous to the manner in which a mechanical lock distinguishes the proper key. In most enzymes, the “lock” is formed by a cleft or pocket on the protein’s surface that forms part of a region called the active site (Figures 5–6 and 5–8). As implied by the adjective “active,” the active site is much more than simply a recognition site for binding substrates. Within the active site, substrates are brought into close proximity to one another in optimal alignment with the cofactors, prosthetic groups, and amino acid side chains responsible for catalyzing their chemical transformation into products (Figure 7–3). Catalysis is further enhanced by the capacity of the active site to shield substrates from water and generate an environment whose polarity, hydrophobicity, acidity, or alkalinity can differ markedly from that of the surrounding cytoplasm.
FIGURE 7–3 Two-dimensional representation of a dipeptide substrate, glycyl-tyrosine, bound within the active site of carboxypeptidase A.
ENZYMES EMPLOY MULTIPLE MECHANISMS TO FACILITATE CATALYSIS
Enzymes use various combinations of four general mechanisms to achieve dramatic catalytic enhancement of the rates of chemical reactions.
Catalysis by Proximity
For molecules to react, they must come within bond-forming distance of one another. The higher their concentration, the more frequently they will encounter one another, and the greater will be the rate of their reaction. When an enzyme binds substrate molecules at its active site, it creates a region of high local substrate concentration. This environment also orients the substrate molecules spatially in a position ideal for them to interact, resulting in rate enhancements of at least a thousandfold.
Acid–Base Catalysis
The ionizable functional groups of aminoacyl side chains and (where present) of prosthetic groups contribute to catalysis by acting as acids or bases. Acid-base catalysis can be either specific or general. By “specific” we mean only protons (H3O+) or OH– ions. In specific acid catalysis or specific base catalysis, the rate of reaction is sensitive to changes in the concentration of protons of but independent of the concentrations of other acids (proton donors) or bases (proton acceptors) present in the solution or at the active site. Reactions whose rates are responsive to all the acids or bases present are said to be subject to general acid catalysis or general base catalysis.
Catalysis by Strain
Enzymes that catalyze lytic reactions that involve breaking a covalent bond typically bind their substrates in a conformation that is somewhat unfavorable for the bond that will undergo cleavage. This conformation mimics that of the transition state intermediate, a transient species that represents the transition state, or half-way point, in the transformation of substrates to products. The resulting strain stretches or distorts the targeted bond, weakening it and making it more vulnerable to cleavage. Nobel Laureate Linus Pauling was the first to suggest a role for transition state stabilization as a general mechanism by which enzymes accelerate the rates of chemical reactions. Knowledge of the transition state of an enzyme-catalyzed reaction is frequently exploited by chemists to design and create more effective enzyme inhibitors, called transition state analogs, as potential pharmacophores.
Covalent Catalysis
The process of covalent catalysis involves the formation of a covalent bond between the enzyme and one or more substrates. The modified enzyme then becomes a reactant. Covalent catalysis introduces a new reaction pathway whose activation energy is lower—and the reaction therefore is faster—than the reaction pathway in homogeneous solution. Chemical modification of the enzyme is, however, transient. Completion of the reaction returns the enzyme to its original unmodified state. Its role thus remains catalytic. Covalent catalysis is particularly common among enzymes that catalyze group transfer reactions. Residues on the enzyme that participate in covalent catalysis generally are cysteine or serine and occasionally histidine. Covalent catalysis often follows a “ping-pong” mechanism—one in which the first substrate is bound and its product released prior to the binding of the second substrate (Figure 7–4).
FIGURE 7–4 “Ping-pong” mechanism for transamination. E—CHO and E—CH2NH2 represent the enzyme-pyridoxal phosphate and enzyme-pyridoxamine complexes, respectively. (Ala, alanine; Glu, glutamate; KG, α-ketoglutarate; Pyr, pyruvate.)
SUBSTRATES INDUCE CONFORMATIONAL CHANGES IN ENZYMES
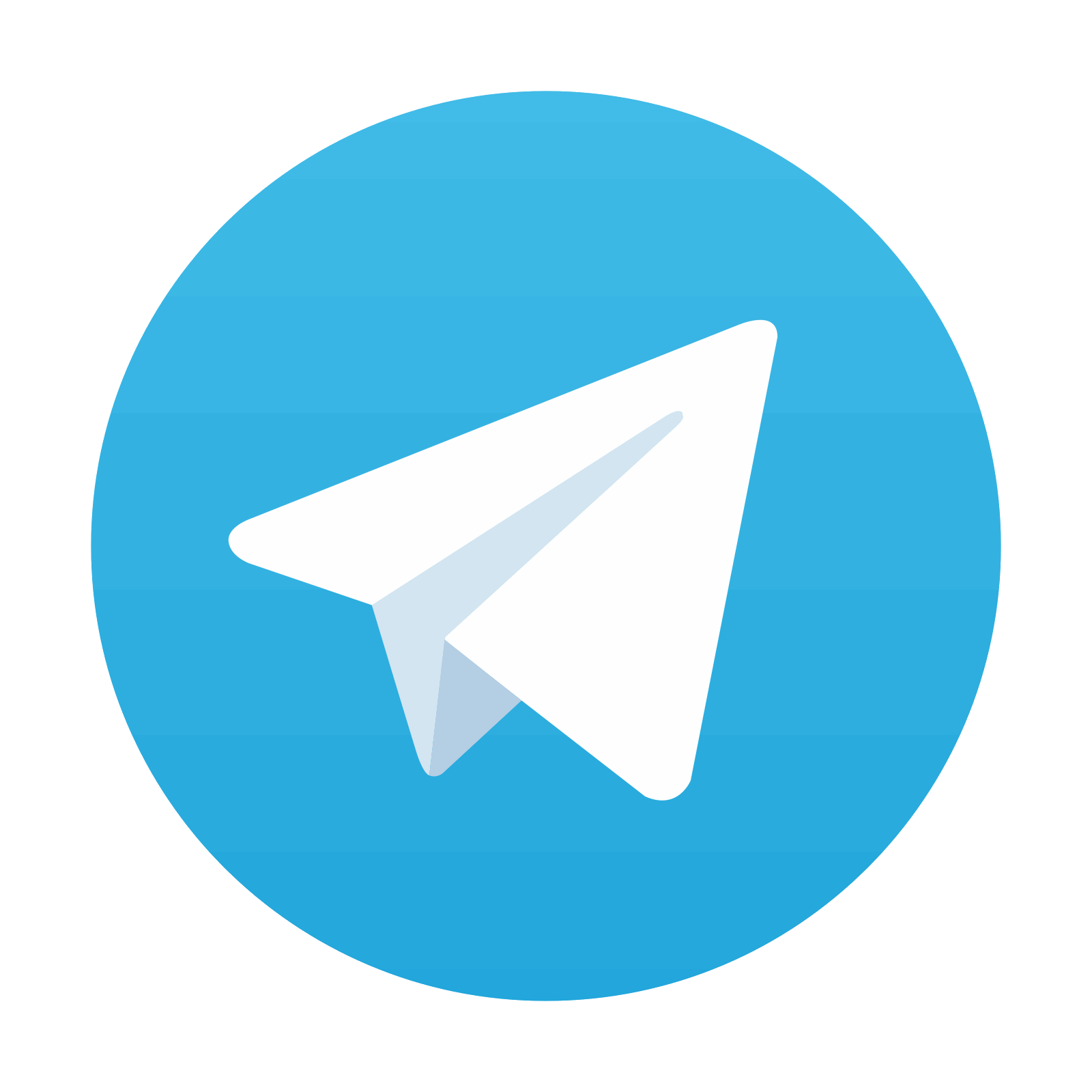
Stay updated, free articles. Join our Telegram channel

Full access? Get Clinical Tree
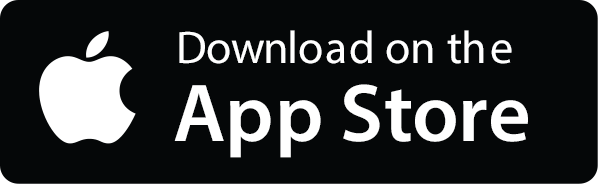
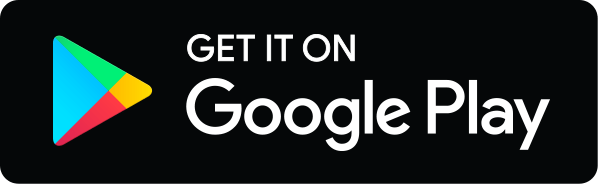