Enteroaggregative Escherichia coli
Nadia Boisen1, Karen A. Krogfelt2 and James P. Nataro1, 1University of Virginia School of Medicine, Charlottesville, VA, USA, 2Statens Serum Institut, Copenhagen, Denmark
Introduction
Diarrhea is a significant cause of morbidity and mortality worldwide, particularly in children under 5 years of age. In addition to almost one million directly attributable deaths per year (Black et al., 2010), morbidity associated with repeated episodes during childhood diarrhea can be lifelong (Petri et al., 2008). Repeated bouts of diarrhea during infancy result in malabsorption of nutrients leading to developmental disabilities, which include growth shortfalls and impaired cognition.
Collectively, the diarrheagenic Escherichia coli (DEC) represent the most common bacterial pathogen worldwide (Farthing, 2000; Wanke, 2001; Ina et al., 2003). Included among the DEC is enteroaggregative Escherichia coli (EAEC), a pathogen of emerging significance. However, identification and isolation of pathogenic strains remain elusive, given an as yet imperfect definition of this pathotype. Thus, the global burden of diarrheal diseases resulting from EAEC may be vastly underestimated (Bryce et al., 2005). This underestimation is also true of the sequelae of persistent diarrhea for which EAEC is reportedly a prevalent cause (Fang et al., 1995; Lima et al., 2000). Hence, detailed understanding of EAEC heterogeneity and pathogenic mechanisms are important.
Enteroaggregative Escherichia coli (EAEC) history
EAEC was first described by the senior author in 1983, while a student in the lab of Dr. James Kaper. During adhesion studies of E. coli to Hep-2 cells it was observed that three distinct adhesion patterns could be described as diffuse, localized and aggregative patterns. The role of these patterns in disease was yet to be elucidated. Thus, the first association of EAEC with diarrheal disease was published in 1987, as part of a prospective study of pediatric diarrhea in Chile (Nataro et al., 1987). Shortly thereafter, EAEC was associated with persistent diarrhea among children in three studies (Bhan et al., 1989a,b; Cravioto et al., 1991).
EAEC is a pathotype of DEC defined as E. coli that do not secrete the heat-stable (ST) or heat-labile (LT) toxins of enterotoxigenic Escherichia coli (ETEC), and which manifest a characteristic aggregative or ‘stacked brick’ pattern (AA) of adherence to HEp2-cells in culture (Figure 8.1).
Rapid developments in molecular microbiology lead to novel definitions for EAEC, yet the gold standard is the adhesion pattern on cell monolayers.
Typical EAEC applies to EAEC strains possessing the AggR regulon (described below). Typical EAEC strains have been linked to acute diarrhea (Sarantuya et al., 2004; Huang et al., 2006). However, atypical EAEC lack the AggR regulon and are not reliably associated with diarrhea (Nataro, 2005). Since the original description, EAEC has emerged as an important pathogen in several clinical scenarios, including travelers’ diarrhea (Adachi et al., 1999, 2001, 2002; Glandt et al., 1999; Tompkins et al., 1999), endemic pediatric diarrhea among children in developed countries (Tompkins et al., 1999), and developing countries (Okeke et al., 2000a), as well as persistent diarrhea amongst HIV-infected patients (Wanke et al., 1998a,b; Durrer et al., 2000; Mossoro et al., 2002; Gassama-Sow et al., 2004). A meta-analysis by Huang et al. (2006) showed that EAEC is a cause of acute diarrheal illness among different subpopulations in both developing and industrialized regions.
Epidemiology
EAEC epidemiology is poorly understood. There is no evidence for an animal reservoir of the bacterium (Huang et al., 2004a). Foodborne outbreaks have been described (Itoh et al., 1997). Risk factors for EAEC infection include travel to developing countries, ingestion of contaminated food and water, poor hygiene, host susceptibility, and possibly immunosuppression (Nataro et al., 1985; Okeke and Nataro, 2001; Huang and Dupont, 2004, 2006).
Definitive data demonstrating pathogenicity of individual EAEC strains is sparse. In volunteer studies, archetype strain 042 elicited clinical diarrhea in three of five subjects, and enteric symptoms in another (Nataro et al., 1995). In a prior study, strain JM221 elicited enteric symptoms in some patients. Interestingly, however, some other EAEC strains failed to cause diarrhea in volunteers. These results suggest heterogeneity of EAEC virulence, although the molecular basis of this variation remains elusive. Some studies suggest that children are more likely to be affected in the first month of life (Gonzalez et al., 1997; Gascon et al., 1998), while others have stressed that most cases arise in older children (Okeke et al., 2000a). These disparate epidemiologic observations could be reconciled by diverse virulence characteristics of the strains circulating at the respective sites, in combination with a variety of host immunity and host resistance.
Outbreaks
The first reported EAEC outbreak occurred in a Serbian nursery in 1995 (Cobeljic et al., 1996); of the 19 afflicted infants, three developed persistent diarrhea. There have been two EAEC outbreaks reported in Mexico City (Eslava et al., 1993). Itoh et al. (1997) described a massive outbreak of EAEC diarrhea among Japanese children, affecting nearly 2700 patients. Outbreaks have also been reported among adults in the UK (Smith et al., 1997).
Morabito et al. (1998) described an outbreak of hemolytic uremic syndrome (HUS) in France caused by a Shiga toxin-producing EAEC strain. Sporadic small outbreaks of hemolytic uremic syndrome (HUS) have been attributed to Stx-producing EAEC of the O111:H2, O111:H21, and O104:H4 serotypes over the last 15 years (Morabito et al., 1998; Frank et al., 2011; Scheutz et al., 2011); however, they were localized to small populations in France, Ireland, and the Republic of Georgia and were not disseminated throughout Europe.
From May through June 2011, Europe was struck by an outbreak of massive proportions, infecting 4137 individuals and resulting in 54 deaths (see Chapter 11). By epidemiological linking, contaminated sprouts were found to be the source of the outbreak, although not isolated from the source (Frank et al., 2011; Scheutz et al., 2011). There are troubling differences between the German outbreak and previous large outbreaks: (i) HUS represented 22% (845) of the ascertained cases, which is a much higher proportion than in other outbreaks (6–10%); (ii) it was predominately seen in healthy adults, which was highly uncommon with diarrhea-associated HUS, that occurs primarily in children; and (iii) the causative agent was a non-O157 Stx-producing EAEC strain of serotype O104:H4 (Frank et al., 2011).
Rasko et al. (2011) performed genetic characterization of the outbreak strain classifying it within the EAEC pathotype. EAEC of serotype O104:H4 strains are closely related and form a distinct clade among E. coli and EAEC strains. However, the genome of the German outbreak strain can be distinguished from those of other O104:H4 strains because it contains a prophage encoding Shiga toxin 2 and a distinct set of additional virulence (such as AAF/I, Pic, SepA, and SigA) and antibiotic-resistance genes. Stepwise horizontal genetic exchange events allowed for the emergence of the highly virulent Shiga-toxin-producing EAEC strain (Rasko et al., 2011). Comparing genomes of related strains isolated during the outbreak suggested that environmental selection enhanced the magnitude of the outbreak (Grad et al., 2012).
Endemic diarrhea in developing countries
EAEC is best known for its role in persistent diarrhea (>14 days) in infants and children in developing countries. Studies in Mongolia (Sarantuya et al., 2004), India (Dutta et al., 1999), Brazil (Piva et al., 2003; Zamboni et al., 2004), Nigeria (Okeke et al., 2000a,b), Israel (Shazberg et al., 2003), Venezuela (Gonzalez et al., 1997), Congo (Jalaluddin et al., 1998) and many other countries, have identified EAEC as a highly prevalent (often the most prevalent) E. coli pathotype in infants. The role of EAEC as a cause of persistent diarrhea and malnutrition in Brazil has been demonstrated repeatedly (Lima and Guerrant, 1992; Fang et al., 1995; Huang et al., 2006). In one study, 68% of those with persistent diarrhea shed EAEC in their stools (Fang et al., 1995). In Guinea-Bissau the most common bacteria isolated from feces of children <2 years of age with diarrhea was EAEC (Valentier-Branth et al., 2003).
Endemic diarrhea in developed countries
A large prospective study in England (Tompkins et al., 1999), in which over 3600 cases of diarrhea and controls were studied, found that typical EAEC was the second most common bacterial cause of gastroenteritis, following Campylobacter. EAEC was significantly associated with diarrhea in both prospective cohorts and in patients presenting to physicians’ attention. Typical EAEC were found as major cause of bacterial diarrhea among infants in Cincinnati, OH, USA (Coohen et al., 2005). A large prospective study of diarrhea was conducted across all ages in Baltimore, MD, USA and New Haven, CT, USA and EAEC was significantly associated with diarrhea, being the most common bacterial cause of diarrhea at both sites (Nataro et al., 2006). This observation has recently been confirmed in a similar study performed in New Jersey (Cennimo et al., 2009). Moreover, a Scandinavian case-control study, found EAEC in significantly more diarrheal cases than controls (Bhatnagar et al., 1993). This is supported by studies from Germany (Huppertz et al., 1997) and Austria (Presterl et al., 1999), which emphasizes the role of EAEC in developed countries.
EAEC as a cause of diarrhea in AIDS patients
The role of EAEC as an important pathogen in AIDS patients continues to develop, and EAEC now ranks among the most important enteric pathogens in this sub-population (Wanke et al., 1998a,b). EAEC was reported as the predominant cause of diarrhea among HIV-infected patients in the Central African Republic (Germani et al., 1998). The importance of EAEC in persistent diarrhea among African AIDS patients was re-emphasized during a study in Senegal (Gassame-Sow et al., 2004). The finding that HIV replication is enhanced by inflammation and activation of NF-κB raises the concern that EAEC-induced inflammation may add to the rapid downward decline of millions of African AIDS patients (Klein et al., 2000).
EAEC in travelers
As outbreaks suggest, EAEC is capable of causing diarrhea in adults. In a recent review of all published studies of traveler’s diarrhea, EAEC was in aggregate second only to ETEC as the most common pathogen among patients with traveler’s diarrhea (Shah et al., 2009). EAEC was a major cause of diarrhea among Spanish travelers going to the developing world, with an incidence identical to that of ETEC (Gascon et al., 1998). Studies in Mexico have shown that in contrast with ETEC, in which travelers are most susceptible during the first weeks of exposure, travelers remain susceptible to EAEC infection throughout their stay (Adachi et al., 2002), likely reflecting the particular ability of EAEC to evade the immune system and cause persistent diarrhea (Okhuysen et al., 2010). Travelers in Mexico showed that the rate of EAEC colonization increased proportionally with the length of the stay (Adachi et al., 2002).
EAEC and malnutrition
Investigators working in Fortaleza, Brazil, have repeatedly implicated EAEC as the predominant agent of persistent diarrhea (Wanke et al., 1991; Fang et al., 1995), which is associated with growth retardation. Interestingly, in this study population even asymptomatic patients infected with EAEC exhibit growth retardation (Steiner et al., 1998) compared with uninfected controls. In longitudinal studies of an infant cohort in Guinea-Bissau, EAEC infection was highly prevalent and was accompanied by growth retardation, although a direct link could not be established (Valentiner-Branth et al., 2001). Given the high rate of asymptomatic excretion of EAEC in much of the developing world (Fang et al., 1995), understanding its potential role in malnutrition and growth retardation is a high priority.
Clinical manifestations of infection
The clinical manifestations of EAEC infection involve complex host–pathogen interaction. At play are the heterogeneity of EAEC strains, number of EAEC ingested, and specific host susceptibility (Huang et al., 2004b). Jiang et al. (2003) identified a specific host genetic determinant that influences the clinical manifestations of EAEC infection. These investigators found that a single nucleotide polymorphism in the promoter of the –251 site of IL-8 conferred an increased risk of developing EAEC diarrhea as well as presence of elevated fecal IL-8 (Jiang et al., 2003).
Data on the clinical presentation of EAEC infection are derived primarily from volunteer studies, studies on traveler’s diarrhea patients, and from outbreak investigations. The common clinical features of EAEC infection include watery diarrhea with or without passage of blood and mucus, abdominal pain, nausea, vomiting, borborygmi (abdominal gurgling), and fever (Nataro et al., 1995; Huppertz et al., 1997; Glandt et al., 1999; Bouckenooghe et al., 2000; Infante et al., 2004; Kahali et al., 2004; Regua-Mangia et al., 2004). As reviewed by Huang et al. (2006) the incubation period spans from 8 to 18 hours. Malnourished hosts, especially children living in developing countries, may be unable to repair mucosal damage and thus become prone to persistent (>14 days) diarrhea (Huang et al., 2006).
During the recent outbreak the disease manifestation was profound and severe since the EAEC strain acquired a Shiga-toxin-producing phage (Scheutz et al., 2011).
Microbial pathogenesis
EAEC as currently defined most likely encompasses both pathogenic and non-pathogenic E. coli strains (Kaper et al., 2004). This conundrum may be resolved upon further understanding of EAEC pathogenesis, which is becoming slowly elucidated. The EAEC genome is highly mosaic, with many putative virulence genes flanked by insertion sequences, and predictably, many E. coli strains harbor a few of the identified genes in various combinations (Boisen et al., 2012). Thus, a major obstacle in understanding EAEC is the inability to define a fundamental pathogenetic strategy. EAEC has unmistakably been associated with diarrhea in some individuals (e.g. in volunteers and outbreak patients), but subclinical colonization in endemic areas is common.
The essential differences between pathogenic and non-pathogenic strains are largely unknown, but pathogenesis studies suggest three general stages of infection: (i) adherence to the intestinal mucosa by virtue of aggregative adherence fimbriae (AAF) or other adherence factors (Tzipori et al., 1992; Hicks et al., 1996); (ii) stimulation of mucus production, forming a biofilm on the surface of the mucosa (Hicks et al., 1996); and (iii) toxicity to the mucosa, manifested by cytokine release, cell exfoliation, intestinal secretion, and induction of mucosal inflammation (Steiner et al., 1998, 2000; Bouckenooghe et al., 2000; Jiang et al., 2002; Harrington et al., 2005) (Figure 8.2).
FIGURE 8.2 The basic features of EAEC pathogenesis. Stages 1–3 highlighted in yellow illustrate the three main steps in EAEC pathogenesis. See text for discussion. Modified after Harrington et al. (2006).
Virulence determinants
Several putative virulence factors have been identified in EAEC (Table 8.1), including enterotoxins and cytotoxins, secreted proteins and many more. The virulence factors so far described are encoded either on the large virulence plasmid of EAEC called pAA, or on the chromosome. The clinical roles of these factors remain uncertain (Nataro, 2005).
TABLE 8.1
Putative EAEC virulence genes and factors
Factor | Description | Unique to EAEC |
Master regulator gene | ||
AggR | Master regulator of a package of EAEC plasmid virulence genes, including aggregative adherence factors, fimbriae AAF/I-AAF/IV, and a large cluster of genes inserted on a pathogenicity island at the PheU locus (Bernier et al., 2002) | Yes |
AggR regulated genes | ||
AatA | Encodes ABC protein responsible for transporting the dispersin protein out of the outer membrane of EAEC (Nishi et al., 2003) | ND |
Aap | Encodes a 10-kDa secreted protein named dispersin, and is responsible for ‘dispersing’ EAEC across the intestinal mucosa (Sheikh et al., 2002) | No |
AggA | Encodes AAF/I, mediates adherence to colonic mucosa and hemagglutination of erythrocytes (Nataro et al., 1992) | Yes |
AafA | Encodes AAF/II, mediates adherence to colonic mucosa and hemagglutination of erythrocytes (Czeczulin et al., 1997) | Yes |
Agg3A | Encodes AAF/III hemagglutination of erythrocytes (Bernier et al., 2002) | Yes |
Agg4A | Encodes AAF/IV, mediates adherence to colonic mucosa and hemagglutination of erythrocytes (Boisen et al., 2008) | Yes |
AaiC | AaiC, secreted protein. Encoded on the chromosome. Mode of action | ND |
ORF3/4a | Co-regulated two-gene cluster with homology to isoprenoid synthesis genes | ND |
ORF61a | Expressed contact hemolysis of erythrocytes | ND |
CapU | Hexosyltransferase homolog (Czeczulin et al., 1999) | ND |
Toxin | ||
Pet | A 108-kDa autotransporter protein that functions as a heat-labile enterotoxin and cytotoxin (Navarro-Garcia et al., 1998) | Yes |
SigA | IgA protease-like homolog (Rajakumar et al., 1997) | No |
Pic | Pic protein has mucinase activity and is capable of causing hemagglutination of erythrocytes (Henderson et al., 1999a) | No |
SepA | Shigella extracellular protein. May induce mucosal atrophy and tissue inflammation in S. flexneri (Benjelloun-Touimi et al., 1998) | No |
Sat | Secreted autotransporter toxin. Might induce cytoskeletal perturbation in intestinal epithelium accompanied by rearrangement of tight junction proteins (Guyer et al., 2000) | No |
EAST-1 | Encodes the enteroaggregative heat-stable toxin (EAST-1), which has physical and mechanistic similarities to E. coli STa enterotoxin (Savarino et al., 1993) | No |
Others | ||
EilA | Salmonella HilA homolog, activates the bacterial surface protein Air (Sheikh et al., 2006) | ND |
Air | Possible aggregation and adherence (Sheikh et al., 2006) | ND |
Pro. Fl. | Single protein (from strain 042) moiety that has a sequence similar to that of a flagelin from Shigella dysenteriae (Steiner et al., 2000) | No |
Irp2 | Encodes an iron-uptake system mediated by siderophore yersiniabactin that plays a role in iron transport and regulation (Schubert et al., 1998) | No |
Lectin | A complex carbohydrate that shows cross-reactivity to the bindings subunit of cholera toxin, and induces morphological changes in HEp-2 cells and fluid accumulation in the rabbit ileal loop (Basu et al., 2004) | No |
aUnpublished; ND, not determined ; Pro. Fl, proinflamatory flagelins.
The best-studied EAEC factor is AggR, putatively the master regulator of EAEC virulence. AggR is a member of the AraC/XylS family of transcriptional activators with its nearest neighbors being Rns of ETEC and RegA of Citrobacter rodentium, while ToxT of Vibrio cholerae is a distant relative.
Virulence factors regulated by AggR
AggR controls expression of adherence factors, a dispersin surface coat protein and a large cluster of genes encoded on the EAEC chromosome. Nataro (2005) have therefore suggested that a ‘package’ of plasmid-borne and chromosomal virulence factors for EAEC are required to execute pathogenesis, and that this set of factors is under the coordinate control of AggR. This hypothesis has not been rigorously tested.
The natural conditions that result in activation of AggR are unknown. However, activating conditions for the related RegA, Rns, and ToxT have been described: Rns (Grewal et al., 1997) and ToxT (Prouty et al., 2005) regulons are activated by bile, whereas sodium bicarbonate activates the RegA-dependent genes (Hart et al., 2008).
Once ingested the localization of EAEC in the gastrointestinal tract has not been well defined. Electron microscopy of infected small intestinal mucosa revealed bacteria in association with a thick mucus layer above an intact enterocyte brush border, which contained extruded cell fragments. In the colon EAEC induces cytotoxic effects (Hicks et al., 1996). All of these findings suggest that even though EAEC binds to many segments of the small intestinal tract, it is most pathogenic in the colonic epithelium (Huang et al., 2004a).
Aggregative adherence (AA) to the intestinal mucosa represents the first step in the pathogenesis of EAEC (Okeke et al., 2000a) (Figure 8.2). The EAEC-defining phenotype, AA, suggests that adhesins have an important role in pathogenesis, as they do for all enteric pathogens.
Aggregative adherence fimbriae (AAF) are the principal EAEC mucosal adhesins of which at least five variants are known (Nataro et al., 1992; Czeczulin et al., 1997; Bernier et al., 2002; Boisen et al., 2008). The AAFs are members of the chaperone-usher pili family (see Chapter 12) and display a high level of conservation of accessory genes, but with much greater divergence of the fimbrial (pilin) genes. Four structural subunits encoded by aggA (AAF/I), aafA (AAF/II) agg3A (AAF/III), and agg4A (AAF/IV) on the pAA plasmid have been described. AAF/I and AAF/IV confer hemagglutination to human erythrocytes and are responsible for the aggregative phenotype (Nataro et al., 1992; Boisen et al., 2008). Recently, a fifth AAF/V has been discovered (Protein ID number BAI44132.1) which shares ∼35% identities of the mature protein with Agg3A from prototype strain 55989 (Bernier et al., 2002). AAF/II is implicated in intestinal adherence (Czeczulin et al., 1997). AAF/III also functions as a cellular adhesin, and the agg3 biogenesis genes are closely related to those of the agg and aaf operons of AAF/I and AAF/II respectively (Bernier et al., 2002). The structural subunits of AAF/I and II are 25% identical and 47% similar. AAF/I is expressed by 31% of EAEC and AAF/II by 12% (Czeczulin et al., 1997, 1999; Elias et al., 1999).
The AAF family is related to the Dr-family of adhesins found in uropathogenic and diffuse adhering E. coli (DAEC) (see Chapters 9 and 11) (Savarino et al., 1994; Elias et al., 1999). The fimbriae themselves are composed of multimers of major and minor fimbrial subunits. The fimbriae of AAF/I, AAF/II, and AAF/III (AggA, AafA, and Agg3A, respectively) comprise a distinct phylogenetic cluster, as do the original Dr-family, comprising F1845 and the AFA adhesins (Boisen et al., 2008). Collectively, these four variants may account for AA in the large majority of EAEC strains (Boisen et al., 2008, 2009).
The genetic organization of AAF/I and AAF/IV comprises genes encoding a chaperone, usher, putative invasin, and major pilin protein in a single gene cluster (Savarino et al., 1994; Boisen et al., 2008).
The morphology of AAF/II from EAEC archetype strain 042 exhibits a semi-flexible bundle forming structure, which wraps around neighboring bacteria (Figure 8.3). In contrast to the bundle-forming morphology of AAF/I, II and AAF/IV, the AAF/III appear as peritrichous, long and flexible filaments. Transmission electron microscopy shows that fimbriae of AAF/II are thicker (5 nm diameter) compared to those of AAF/I, which are 2–3 nm in diameter (Czeczulin et al., 1997).
FIGURE 8.3 Scanning electron microscopy of EAEC wt strain 042. White arrow indicates AAF/II fimbriae. From Sheikh et al. (2002).
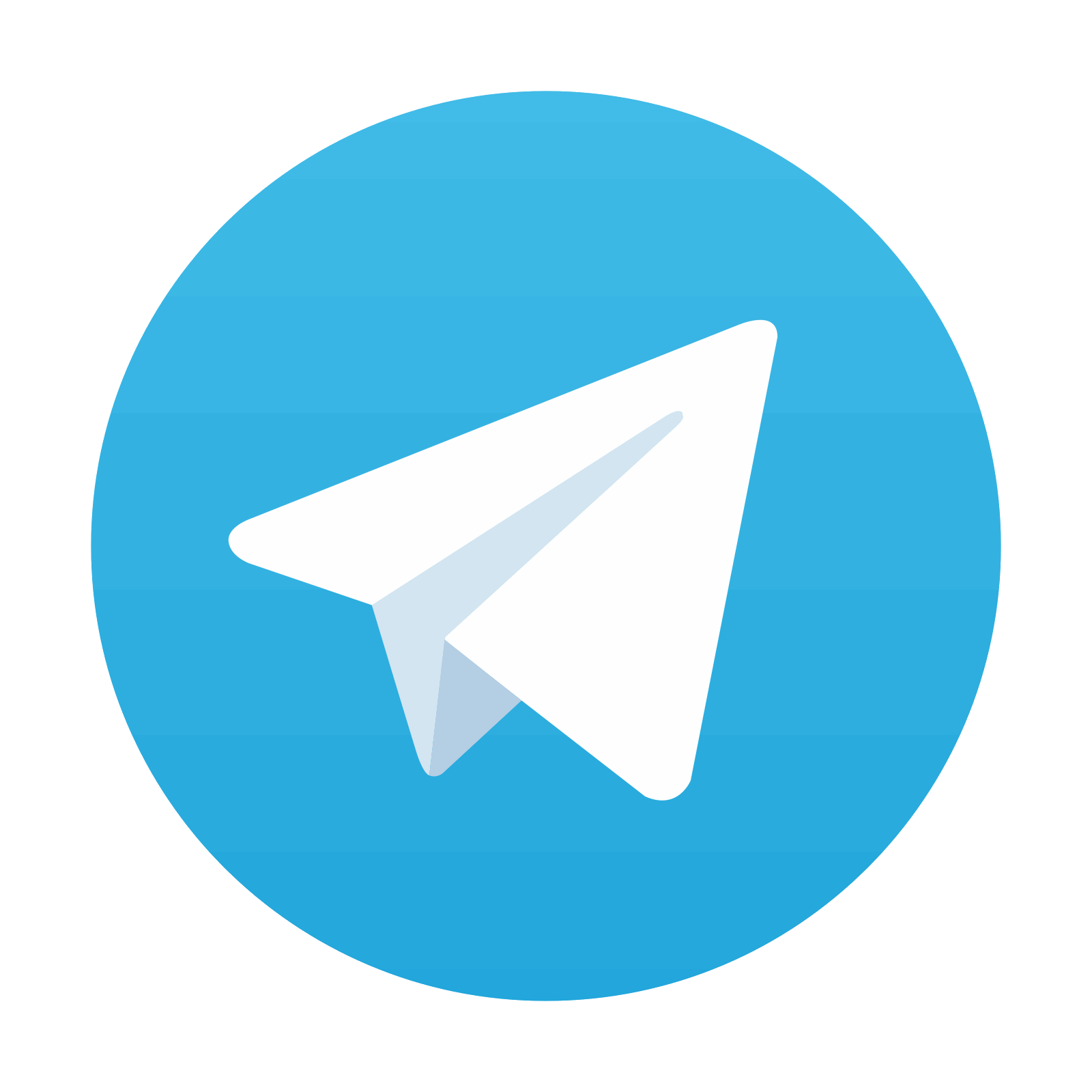
Stay updated, free articles. Join our Telegram channel

Full access? Get Clinical Tree
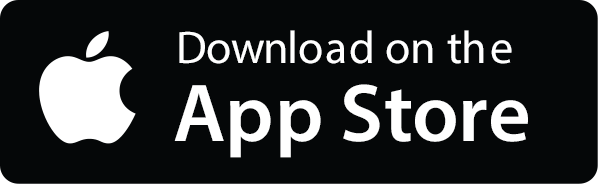
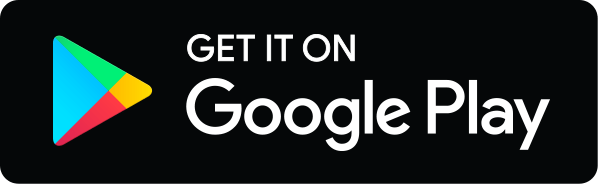