Fig. 31.1
State-of-the-art medical linear accelerator (a) and a patient dose distribution generated by a treatment technique called RapidArc (b). Small animal conformal radiotherapy system at Stanford University based on a modified microCT scanner (c) and a mouse dose distribution delivered by the system (d)
31.2 Components of a Small Animal Conformal Radiotherapy System
A typical small animal conformal radiotherapy system consists of a source of radiation, a collimation system mounted on a gantry, a movable specimen table, and an imaging system. Prior to treatment, the subject is typically imaged with X-ray cone-beam computed tomography (CBCT), reconstructed 3D CBCT volumes are used for specimen positioning and treatment planning. Animals are irradiated with a number of beams calculated by a treatment-planning program, an integral part of every system. Treatment beams have to be collimated to a large degree, so that the beam penumbra (unsharpness) does not degrade the quality of dose distributions and small target volumes can be irradiated while surrounding healthy tissue is spared. The individual components of a small animal conformal radiotherapy system depicted in Fig. 31.2 are described in detail in the following sections.
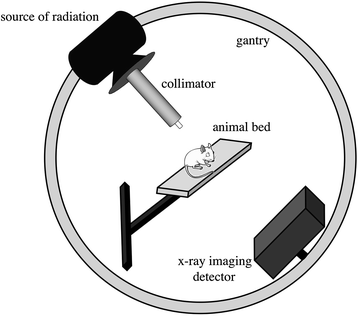
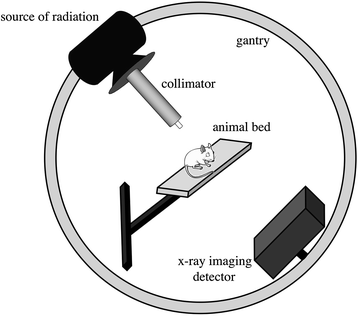
Fig. 31.2
The main components of a small animal conformal radiotherapy system: source of radiation, collimator, X-ray detector, animal bed, and a gantry
31.2.1 Sources of Radiation
Due to the typical size of small animals, clinically used high-energy MV photon beams with maximum dose deposition at depths of 1.5–3.5 cm are not suitable for preclinical irradiations. Kilovoltage (kV) photon beams are preferred for small animal radiotherapy systems for three primary reasons:
1.
More suitable depth–dose deposition for irradiation of small animals compared to MV beams.
2.
Less collimation material needed to generate conformal beams.
3.
Lower radiation-shielding requirements compared to clinical radiotherapy shielded by thick concrete walls.
4.
Generated by more cost-effective and compact X-ray tubes compared to clinical linear accelerators.
As mentioned above, radiotherapy in most current small animal conformal systems is delivered by kV X-ray beams generated with X-ray tubes, but radionuclide sources have also been considered. Dose deposition of a clinical 15-MV linac photon beam, X-ray tube generated 70, 120, and 225 kV beams, and an 192Ir radionuclide source are plotted in Fig. 31.3. Small animals with sizes from 1 to 5 cm can be conveniently treated with kV photon beams and an 192Ir source.
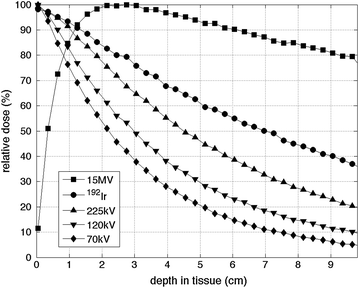
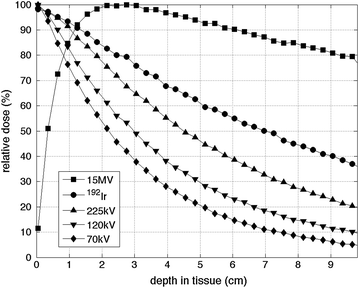
Fig. 31.3
Dose deposition by a 1 × 1 cm beam as a function of depth for a 15 MV, 70, 120, 225 kV X-ray beams and an 192Ir γ-ray source calculated by Monte Carlo simulation
31.2.1.1 X-Ray Tubes
X-ray tubes are used for radiotherapy of small animals because of their compactness and the dose deposition properties of kV X-ray beams. In an X-ray tube, electrons emitted from a cathode are accelerated over a high voltage (50–300 kV) toward an angled anode usually made of tungsten. Electrons interact with the anode material over an area defined by the electron beam size (the focal spot) and generate X-rays in the form of Bremsstrahlung and characteristic radiation. The tube voltage determines the energy and penetration power of the X-ray beam, the higher the energy the deeper the beam penetration.
The output of an X-ray tube (i.e., the dose rate) linearly scales with tube current and with square of tube voltage. For highest dose rate, the X-ray tube current and tube voltage are maximized and limited by the generator power. Small animal conformal radiotherapy is typically done with 120–225 kV and 10–50 mA.
X-ray beam attenuation (beam quality) can be modified by inserting filters in the path of the X-ray beam, generally directly in front of the X-ray tube window. While filtration increased the mean beam energy resulting in less steep attenuation, it decreases the dose rate. A compromise between beam attenuation and output must be found. Mean energies of X-ray beams used for small animal radiotherapy are between 80 and 150 keV corresponding to tube voltages of 120–225 keV.
For larger subjects irradiated with large X-ray beams, the X-ray beam heel effect caused by the angled anode must be accounted for, because it results in uneven beam intensity across the direction of the anode angle. For example, for a 12° anode and a 120 kV beam, the difference between the central axis dose and dose 2 cm off axis at 1 cm depth in tissue and 30 cm source-to-skin distance is 10 %. To accurately account for the heel effect during irradiation, Monte Carlo (MC) dose calculations are performed (Sect. 31.3). The focal spot size is one of the factors determining the geometric beam penumbra (Fig. 31.4a, c). The larger the focal spot size, the larger the beam penumbra. With a larger beam penumbra, it becomes more difficult to irradiate the tumor in a conformal fashion. Typical X-ray tubes offer focal spot sizes with diameters from 0.3 to 5 mm. While use a smaller focal spot is preferred for penumbra reasons, small focal spots exacerbate tube heating, and ultimately limit output.
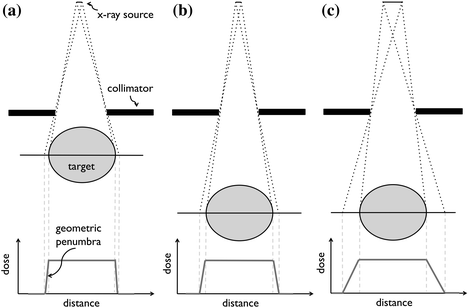
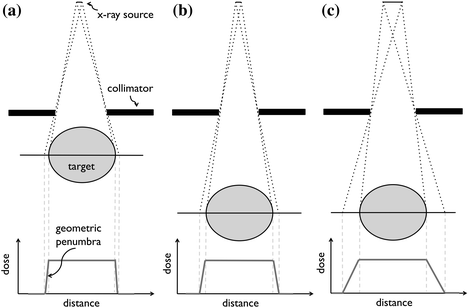
Fig. 31.4
Demonstration of small (a), medium (b), and large (c) geometric beam penumbra (unsharpness) due to treatment source size and collimator-to-target distance. In (a), a small X-ray source and short collimator-to-target distance results in small geometric penumbra. The collimator-to-target distance is enlarged in (b), generating a larger penumbra. In (c), enlarging the X-ray source further increases the beam penumbra. The ideal geometry consists of a small radiation source and a short collimator-to-target geometry
31.2.1.2 Radioisotopes
As an alternative to X-ray tube sources, radioisotopes can be used as treatment sources in small animal conformal radiotherapy systems. The source should have γ-ray energy between 40 and 500 keV for reasonable dose deposition without significant shielding requirements. Moreover, the activity of the source must be high to ensure a high-dose rate, and the half-life of the radionuclide should be reasonable long so that it does not need to be replaced frequently. Moreover, the size of the source affects the geometric beam penumbra (Fig. 31.4), so source sizes in the order of a few millimeters are desirable.
Similar to clinical 60Co source with 1.25 MeV mean beam energy, an 192Ir source with 380 keV γ-ray mean energy can be used for small animal radiotherapy. The deposition of a 192Ir source with a half-life of 74 days is shown in Fig. 31.3. The dose rates of a 192Ir high-dose-rate brachytherapy source are typically on the order of 12 Gy/min at 1 cm distance. Unlike electrically controlled X-ray tubes, radioisotopes cannot be switched off and special care must be taken concerning shielding and personnel exposure.
31.2.2 Collimation Systems
Delivery of conformal radiotherapy is dependent upon shaping the treatment beam to the shape of the target (the tumor). This is clinically achieved by blocking the radiation beam using thick collimators made of high-density materials (typically tungsten). Clinical collimators are designed with various degrees of complexity. Even though small animal radiotherapy beams are of lower energies than clinical beams and therefore require smaller collimator thicknesses, these beams must be smaller, sharper and delivered with a higher spatial accuracy. This presents a challenge in designing collimators for small animal radiotherapy.
Collimator material and thickness are chosen to attenuate the X-ray beam in a small thickness of material. As such, high-density materials are preferred; however, the clinically used costly tungsten is typically replaced by lead or brass. Collimators must attenuate the X-ray beam to 0.1 % of the initial X-ray fluence. The thicknesses of lead and brass can be calculated using the linear attenuation coefficients obtained from the XCOM database [1] based on Beer’s law:
where I and I 0 are the intensity of the attenuated and initial X-ray beam, respectively, μ is the linear attenuation coefficient of the collimator material and d is collimator thickness. Table 31.1 summarizes collimator thicknesses required to attenuate initial X-ray beam with a number of mean energies to 0.1 % of the original X-ray fluence. Lead attenuates X-rays more efficiently and is preferred over brass.

Table 31.1
Linear attenuation coefficient μ and collimator thickness d required to attenuate initial X-ray beam to 0.1 % of its original fluence as a function of beam energy and collimator material
Mean energy (keV) | Lead | Brassa | ||
---|---|---|---|---|
μ (cm−1) | d (mm) | μ (cm−1) | d (cm) | |
80 | 25.8 | 2.7 | 6.7 | 10.3 |
120 | 37.4 | 1.8 | 2.8 | 24.7 |
150 | 21.5 | 3.2 | 1.9 | 35.8 |
In order to generate a sharp beam with a small geometric penumbra, collimators should be placed as close to the target as possible (Fig. 31.4a, b). The distance from the collimator to the isocenter of the machine (the radiation target) is limited by the size of the irradiated animal. Typical distances between the source and the target are 5–15 cm.
Beam-targeting accuracy is driven by the positioning accuracy of the collimators. Careful beam alignment is performed by irradiating a film from a number of beam angles and observing the intersection of the beams.
The four main collimator designs considered for conformal small animal radiotherapy are discussed in the following sections.
31.2.2.1 Removable Collimators
A simple way of collimating the beam is to attach a static removable collimator to the X-ray tube (Fig. 31.5a). Removable collimators typically employ circular openings to create circular fields, or rectangular openings to create rectangular fields. Removable collimators offer the advantage of being in general closer to the target and thus suffer less from geometric penumbra. However, such collimators have to be manually mounted before each beam if the beam size varies for a multibeam treatment. A state-of-the art modulated arc therapy is therefore not possible with removable collimators that have to be inserted manually. Moreover, removable collimators typically offer only a limited number of beam sizes and they are symmetric.
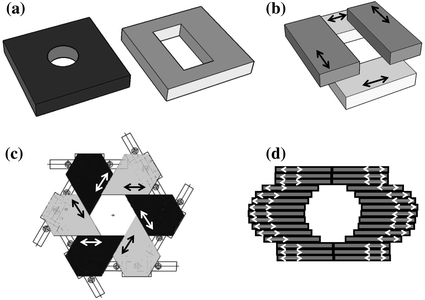
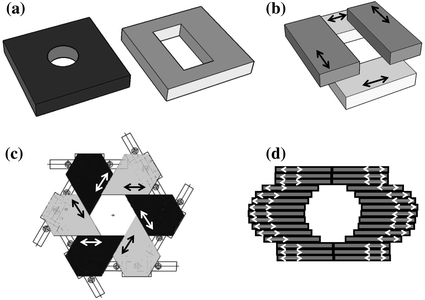
Fig. 31.5
Collimation systems for small animal radiotherapy: removable collimators with circular and rectangular openings (a), movable collimator jaws (b), an iris collimator with six collimator blocks forming a hexagonal opening (c), and a multileaf collimator (d). Arrows indicate the direction of motion of movable parts
31.2.2.2 Movable Jaws
Movable jaws, also used in clinical radiotherapy machines, consist of two pairs of motorized jaws shaping the beam in two perpendicular directions (Fig. 31.5b). The geometric beam penumbra is larger in the direction of the jaw closer to the X-ray tube. Movable jaws can generate rectangular shapes of any sizes in any location. Moreover, X-ray beams shaped by movable jaws can be asymmetric. In principle, the jaws can move during irradiation, which can result in a sophisticated angled dose distribution resembling the clinical enhanced wedge.
31.2.2.3 Iris Collimators
An iris collimator (Fig. 31.5c) consists of a number (n) of motorized blocks organized in a circular geometry so that they form a symmetric polygon opening. The iris collimator has been recently been added to the CyberKnife, a 6 MV clinical linear accelerator mounted on a robotic arm [2]. The iris collimator in Fig. 31.5c generates hexagonal shapes that for small target can approximate circular beams. In order to create more circular-like beam, two n-sided iris collimators rotated by 180/n can be placed in two stages in a similar setup to the movable jaws described above. The size of an iris collimator can be adjusted to any desirable opening. The shape, however, is defined the number of collimator blocks (n) and cannot be modified, and moreover the aperture is always isocentric. This design has been implemented for small animal radiotherapy [3].
31.2.2.4 Multileaf Collimators
The treatment beam in state-of-the art clinical irradiators is shaped by the so-called multileaf collimator (MLC, Fig. 31.5d). A large number of 2.5–5 mm tungsten leaf-pairs (approximately 120) move independently forming the field in practically any beam shape. MLCs have not been yet implemented in small animal conformal radiotherapy, but the field is moving toward their application. Based on Table 31.1, it is most likely that the collimator leafs will be made of lead. Assuming a 1 mm resolution at the target, a small focal spot size, and location of the MLC halfway between the focal spot and target, the MLC leaves must be 0.5 mm wide. To cover a 3 cm field in the isocentric plane, 30 leaf-pairs are needed. Packing a large amount of electronics to operate each of the 60 leafs independently presents an engineering challenge.
31.2.3 Gantry and Animal Bed
Conformal radiotherapy also depends upon delivering treatment beams from multiple angles, which results in lowering the skin and normal tissue dose. For this purpose, the treatment X-ray source is mounted on a gantry enabling its rotation. The rotational gantry also supports beam collimation. Ideally, a full arc 360° rotation is available; however, attempts have been made to deliver small animal and clinical conformal radiotherapy over a smaller angle.
The rotational gantry must support the weight of the equipment (the X-ray tube or radioisotope source, and in some cases the imaging panel) while performing accurate rotation with high positioning accuracy and minimal sagging. Gantry rotation speed requirements are similar to clinical radiotherapy machines that can only rotate at 1 rpm according to IEC rotation limits. If the gantry also contains imaging equipment, a faster rotation of 6 rpm is desirable for faster imaging.
In order to irradiate a target anywhere in a small animal body, it is necessary to either allow off-isocenter beams or to place the target into the isocenter of the gantry, where all beams intersect. As most collimation systems do not allow the former at present, a motorized three-dimensional translation animal bed is therefore an important part of a small animal conformal system. Bed positioning accuracy must be within 0.2 mm in all directions. It should have a high reproducibility with travel ranges at least 10 cm to cover the entire small animal body. Lasers along two or three perpendicular directions can be used for a more accurate animal positioning.
Small animals are irradiated under anesthesia that is either delivered by intraperitoneal injection prior to the experiment, or via inhalation during the procedure. In the latter, more common case, a mixture of oxygen and isoflurane gas is supplied to the animal from outside of the shielded system by tubes. Moreover, due to possible long irradiation times, animals are kept on an electric heating pad to maintain body temperature. Tubing and cables are run through a special entry port in order to minimize radiation leakage from the irradiator.
31.2.4 Integrated Imaging
Imaging is a crucial component of conformal radiotherapy machines, as it is important to accurately locate and define the radiation target. Historically, X-ray computed tomography (CT) imaging has been used for localization of the target, as well as for dose calculations. Similar to clinical radiotherapy, CT images must also be acquired for accurate calculation of radiation dose to the small animal. More recently, molecular bioluminescence imaging (BLI) has been considered for the use in targeting small animal conformal radiotherapy. Both of these techniques are discussed in the following sections.
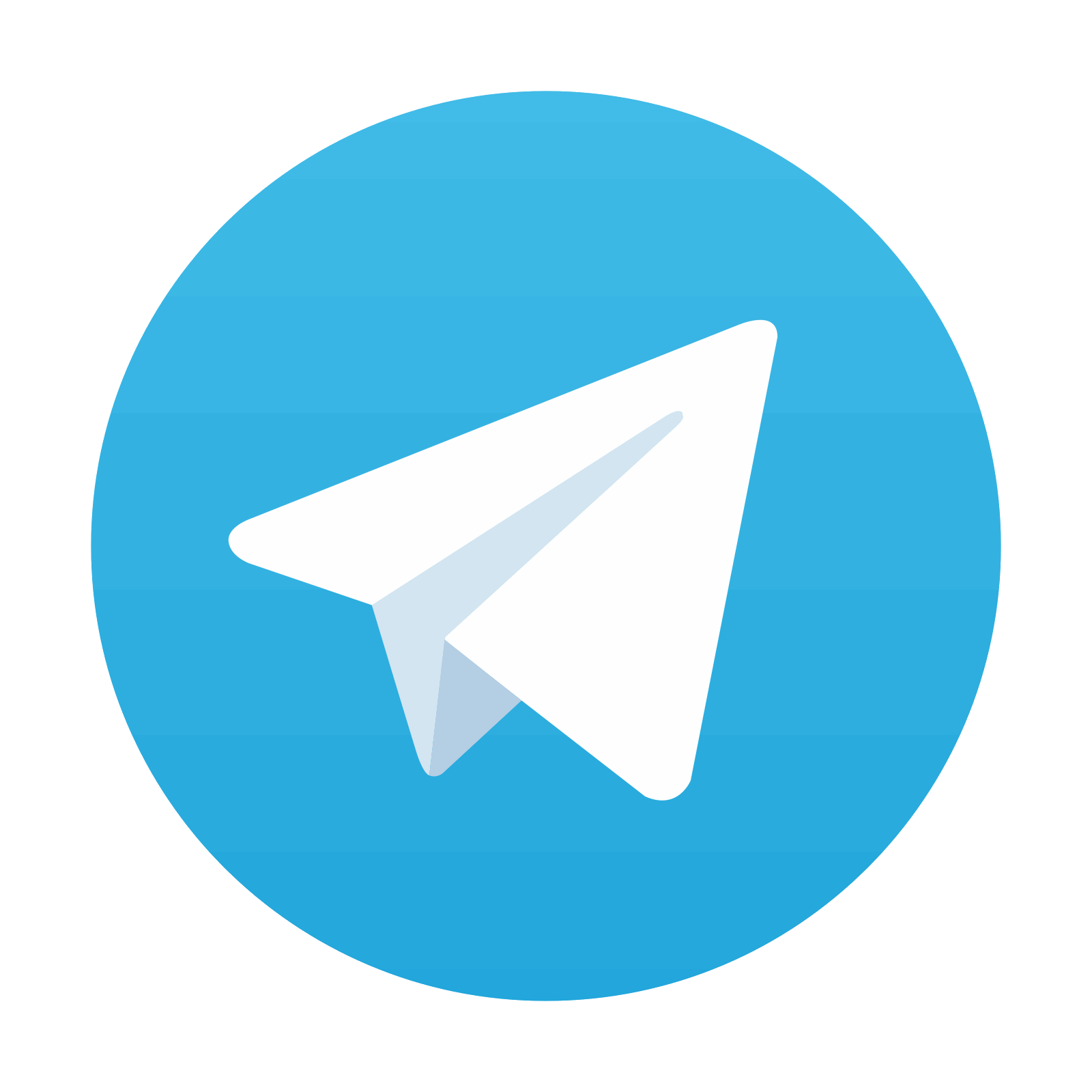
Stay updated, free articles. Join our Telegram channel

Full access? Get Clinical Tree
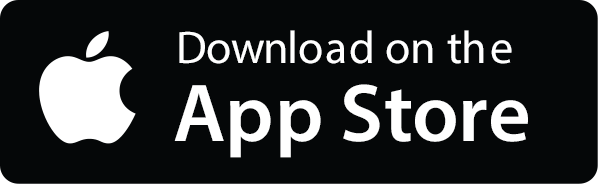
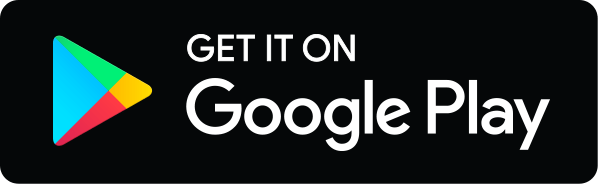