Fig. 13.1
Genetic engineering in phytoremediation
Metal-hyperaccumulating plants and microbes with unique abilities to tolerate, accumulate, and detoxify metals and metalloids represent an important reservoir of unique genes. These genes could be transferred to fast-growing plant species to improve phytoremediation (Fulekar et al. 2009). It has been established that the adaptive metal tolerance is governed by a small number of major genes and some minor modifier genes. Probably, it is this adaptive metal tolerance that gears a plant species for hyperaccumulation.
Use of tissue culture techniques to select genes having enhanced bio-degradative properties (for organics) or the enhanced ability to assimilate metals helps to select plants with the desired characters. Molecular techniques such as the analysis of molecular variance of the random amplified polymorphic DNA (RAPD) markers are useful to investigate the genetic diversity and heavy metal tolerance in plant populations, providing the opportunity to investigate the first steps in the differentiation of plant populations under severe selection pressure and to select plants for phytoremediation.
The genetic and biochemical basis is becoming an interesting target for genetic engineering. A fundamental understanding of both uptake and translocation processes in normal plants and metal hyperaccumulators, regulatory control of these activities, and the use of tissue specific promoters offers the ability to develop effective and economic phytoremediation plants for soil metals. Examples include genes controlling the synthesis of peptides that sequester metals, like phytochelatins, genes encoding transport proteins, or genes encoding enzymes that change the oxidation state of heavy metals. The genes involved in the metabolism of chemical compounds can be isolated from various organisms, including bacteria, fungi, plants, and animals, and these genes are then introduced into candidate plants. The desired characters for phytoremediation can be improved by identifying candidate protein, metal chelators, and transporter genes for transfer and/or overexpression of a particular gene.
13.2.1 Plant Metal Transporters
One of the promising approaches to enhance the ability of metal ions to enter plant cells is the identification of the metal transporter proteins and introducing genes encoding transporter molecules. These are generally proteins found in the cell membrane, that either have an affinity for metal ions, or that create favorable energetic conditions to allow metals to enter the cell. Till date, several plant metal transporters have been reported and more remain to be recognized. Some of the transporters identified so far include the Arabidopsis IRT1 gene that encodes a protein that regulates the uptake of iron and other metals (Eide et al. 1996) and the MRP1 gene encoding, an Mg-ATPase transporter, also from Arabidopsis (Lu et al. 1997).
Further, success in this approach is achieved by identifying proteins such as ZNT1, ZIP1-4, IRT1, COPT1, LCT1, and tVramp-1/3/4 on the plasma membrane-cytosol interface; ZAT, ABC type, HMT1, AtMRP, CAX2 seen in vacuoles; and RAN1 in Golgi bodies. Manipulations of these transporters to achieve the removal of metal ions from the cell hold great potential (Tong et al. 2004). The natural resistance-associated macrophage proteins (Nramp) family of transporters has been recently characterized from rice and Arabidopsis.
13.2.2 Phytochelatins for Metal Sequestering
The principal classes of metal chelators include phytochelatins, metallothioneins, organic acids, and amino acids. Phytochelatins (PCs) are small metal-binding peptides found in plants. Iso-PCs, a series of PC-like homologous chelating peptides, are reported with varying terminal amino acids and have a C-terminal modified residue other than glycine. The PC and iso-PC molecules form complexes with heavy metals like Cd. In addition to PC-Cd complex other PC-metal complexes include Ag, Cu, and As (Shah and Nongkynrih 2007).
In vitro experiments have shown that a series of metal-sensitive plant enzymes can tolerate a 10- to 1,000-fold concentration of Cd in the form of a PC complex than as free radical ion. PC reactivate metal poisoned plant enzymes such as nitrate reductase up to 1,000-fold better than chelators such as glutathione (GSH) or citrate, signifying the extraordinary sequestering potential of these peptides.
13.2.3 Metallothioneins
Metallothioneins (MTs) are metal-binding proteins that confer heavy metal tolerance and accumulation (Hamer 1986; Malin and Bülow 2001). These are low molecular mass cysteine rich proteins that were originally isolated as Cu, Cd, and Zn binding proteins in mammals. MTs are able to bind a variety of metals by the formation of mercaptide bonds between the numerous cysteine (Cys) residues present in the proteins and the metal, and it is the arrangement of these residues that in part determines the metal-binding properties of the MT proteins. Researchers successfully reported more than 50 MTs in different plants categorized into four types, types 1–4. These are based on the Cys arrangement. Although MTs are expressed throughout the plant, some have been found to be expressed in a tissue-specific manner. Transgenic plants expressing MTs have been created, and although these plants exhibited enhanced tolerance to high metal concentrations, the uptake of metals was not enhanced. To enhance higher plant metal sequestration, the yeast MT CUP1 was introduced into tobacco plants, and the cup 1 gene expression and Cu and Cd phytoextraction were determined. Overexpression of Cu inducible MT cup 1 also enhanced Cu tolerance in plants. In plants, a wide range of MT genes from various sources have been overexpressed including those from human, mouse, Chinese hamster, and yeast.
The vacuole is generally considered to be the main storage site for metals in yeast and plant cells and there is evidence that phytochelatin-metal complexes are pumped into the vacuole. The best characterized of the known vacuolar transporters and channels involved in metal tolerance are YCF1 from yeast, Saccharomyces cerevisiae. YCF1 is an MgATP-energized glutathione S-conjugate transporter responsible for vacuolar sequestration of compounds after their S-conjugation with glutathione. Overexpression of the YCF1 gene in Arbidobsis thaliana and the YCF1 proteins were found to be associated with the tonoplast and the plasma membrane. The vacuoles of the YCF1-transgenic plants exhibited a 4-fold higher rate of glutathione-Cd uptake than those of wild-type plants, indicating that expression of YCF1 strongly increases Cd transport activity. The transgenic plants showed improved resistance to both Cd and Pb and elevated metal content, characteristics desirable for phytoremediation.
13.2.4 Genes to Change the Oxidation State of Heavy Metals
Genes may be introduced that code for enzymes to change the oxidation state of heavy metals like Hg and selenium (Se). For instance, introduction of the bacterial merA gene encoding mercuric oxide reductase (Rugh et al. 1996), or that which converts metals into less toxic forms, such as enzymes that can methylate Se into dimethylselenate (Hansen et al. 1998). In both these cases, the resulting form of the metal is volatile, so that one can create a plant capable of metal remediation by phytovolatilization, another type of phytoremediation.
13.3 Genetically Modified Plants for Metal Uptake, Tolerance, and Detoxification
Several researchers have already reported encouraging results using plants bioengineered with increased heavy metal tolerance and uptake of heavy metals for the purpose of phytoremediation. The majority of these novel plants have only been tested under limited laboratory conditions and very few have been grown in the field (Table 13.1).
Table 13.1
Genetically modified organisms used for enhanced phytoremediation of organic contaminants
Transgenic species | Gene source | Gene(s) | Enzyme(s) | Transgene effects | References |
---|---|---|---|---|---|
Arabidopsis thaliana | Cotton | LACI | Root-specific laccase | Cotton secretes laccase to the rhizosphere and has shown enhanced resistance to phenolic alleleochemicals and enhanced tolerance to 2, 4, 6,-trichlorophenol | Wang et al. (2004) |
A. thaliana | A. thaliana | 743B4, 73C1 | Glycosyltransferases (UGTs) | Overexpression of UGTs genes resulted in the enhanced detoxification of TNT and enhanced root growth | Gandia-Herrero et al. (2008) |
Potato (Solanum tuberosum) | Rat | CYP1A1 | Cytochrome P450 monooxygenase | Increased tolerance to atrazine and chlortoluron, assumed to be via metabolism to less-toxic derivatives | Yamada et al. (2002) |
Tobacco (Nicotiana tabacum) | Human | CYP2E1 | Cytochrome P450 monooxygenase | Dramatically enhanced metabolism of trichloroethylene; increased uptake and debromination of ethylene dibromide | Doty et al. (2000) |
Tobacco (N. tabacum) | Enterobacter cloacae | onr | Pentaerythritol tetranitrate | Enhanced denitration of glycerol trinitrate | French et al. (1999) |
Tomato (Lycopersicon esculentum) | Roots of L. esculentum | tpxI | Peroxidases | The overexpression of tpxI gene in transgenic tomato hairy roots resulted in the enhanced removal of phenol | Oller et al. (2005) |
Hybrid poplar (Populus tremula × Populus alba) | Rabbit | CYP2E1 | Cytochrome P450 monooxygenase | Enhanced removal and degradation of trichloroethylene, vinyl chloride, carbon tetrachloride, benzene, and chloroform; enhanced removal of gaseous trichloroethylene, chloroform, and benzene | Doty et al. (2007) |
Brassica juncea | Brassica juncea | γ–ECS, GS | γ-Glutamycysteine synthetase; Glutathione synthetase | Overexpression of ECS and GS resulted in enhanced tolerance to atrazine, 1-chloro-2, 4-dinitrobenzene, phenanthrene, metolachlor | Flocco et al. (2004) |
Rice (Oryza sativa) | Human | CYP1A1 | Cytochrome P450 monooxygenase | Enhanced metabolism of atrazine, norflurazon, and chlortoluron (should also metabolize PAHs) | Kawahigashi et al. (2003) |
Rice (O. sativa) | Human | CYP1A1, CYP2B6, CYP2C19 | Cytochrome P450 monooxygenases | Enhanced metabolism of atrazine, norflurazon, and metolachlor from soil (should also metabolize PAHs) | Kawahigashi et al. (2006) |
Rice (O. sativa) | Human | CYP2C9 | Cytochrome P450 monooxygenases | Tolerance to sulfonylurea | Hirose et al. (2005) |
Pseudomonas fluorescens | Burkholderia sp. | bph operon | Suite of enzymes for the complete PCB degradation pathway | Enhanced rate of degradation of numerous PCBs (resting cell assays) | Villacieros et al. (2005) |
P. fluorescens (psychrotolerant strain) | Burkholderia sp. | dnt genes | Suite of enzymes for degradation of 2, 4-dinitrotoluene to pyruvate and propionyl-CoA | Complete degradation of 2, 4-dinitrotoluene as a co-substrate at temperatures as low as 10 °C | Monti et al. (2005) |
P. fluorescens | Burkholderia cepacia | tomA | Toluene-o-mono-oxygenase | Sixty-three percent degradation of trichloroethylene after 4 days in wheat rhizosphere | Yee et al. (1998) |
13.3.1 Arabidopsis
Arsenic is an extremely toxic metalloid pollutant and the decontamination of polluted sites can be environmentally destructive. It is a lethal poison that is released into the environment from natural processes and via arsenic-based chemicals. Genetically engineered Arabidopsis plants can sequester As from the soil. These plants can transport As aboveground, reduce it to arsenite, and sequester it in thiol-peptide complexes (Dhankher et al. 2002). By coexpressing two bacterial genes, arsenate reductase (ArsC) and γ-glutamylcysteine synthetase (γ-ECS) in Arabidopsis plants, it was observed that plants expressing SRS1p/ArsC and ACT2p/γ-ECS together showed substantially greater As tolerance than wild-type plants or plants expressing γ-ECS alone. In addition, when grown on As, these plants accumulated 4- to 17-fold greater fresh shoot weight and accumulated 2- to 3-fold more As per gram of tissue than wild-type plants or plants expressing γ-ECS or ArsC alone.
Extensive progress has also been achieved in identifying genes and proteins involved in uptake of iron (Fe) by yeast and plants. As described earlier, the utility of the yeast protein YCF1, a protein which detoxifies Cd by transporting it into vacuoles, has been implemented for the remediation of Cd and Pb. Transgenic A. thaliana plants overexpressing YCF1 showed an enhanced tolerance and accumulated greater amounts of Cd and Pb.
The close relationship between Arabidopsis halleri, a metal tolerant and hyperaccumulating relative of the biological model species A. thaliana, has recently allowed the use of A. thaliana GeneChips to compare gene expression levels between A. halleri and the nontolerant A. thaliana and, consequently, permitted the identification of genes potentially involved in metal tolerance and/or hyperaccumulation. The complete annotation of the A. thaliana genome sequence provides a solid foundation for comparative mapping studies within the Brassicaceae family. The genome organization of A. thaliana has already been compared with those of several species like Arabidopsis lyrata, Arabidopsis petraea and Capsella rubella. Moreover, A. halleri is a species that has undergone natural selection for Zn tolerance. Isolation of the quantitative trait loci (QTL) associated with this trait holds great promise for the identification of the main genes responsible for this adaptation.
13.3.2 Brassica
Brassica juncea was genetically engineered to investigate rate-limiting factors for glutathione and phytochelatin production. To achieve this Escherichia coli gshII gene was introduced. Cd-treated GS plants had higher concentrations of glutathione, phytochelatin, thiol, sulphur, and calcium than wild-type plants (Zhu et al. 1999). A study showed that γ-glutamylcysteine synthetase inhibitor, L-buthionine-[S,R]-sulphoximine (BSO), dramatically increased As sensitivity both in nonadapted and As-hypertolerant plants, showing that phytochelatin-based sequestration is essential for both normal constitutive tolerance and adaptative hypertolerance to this metalloid (Schat et al. 2002).
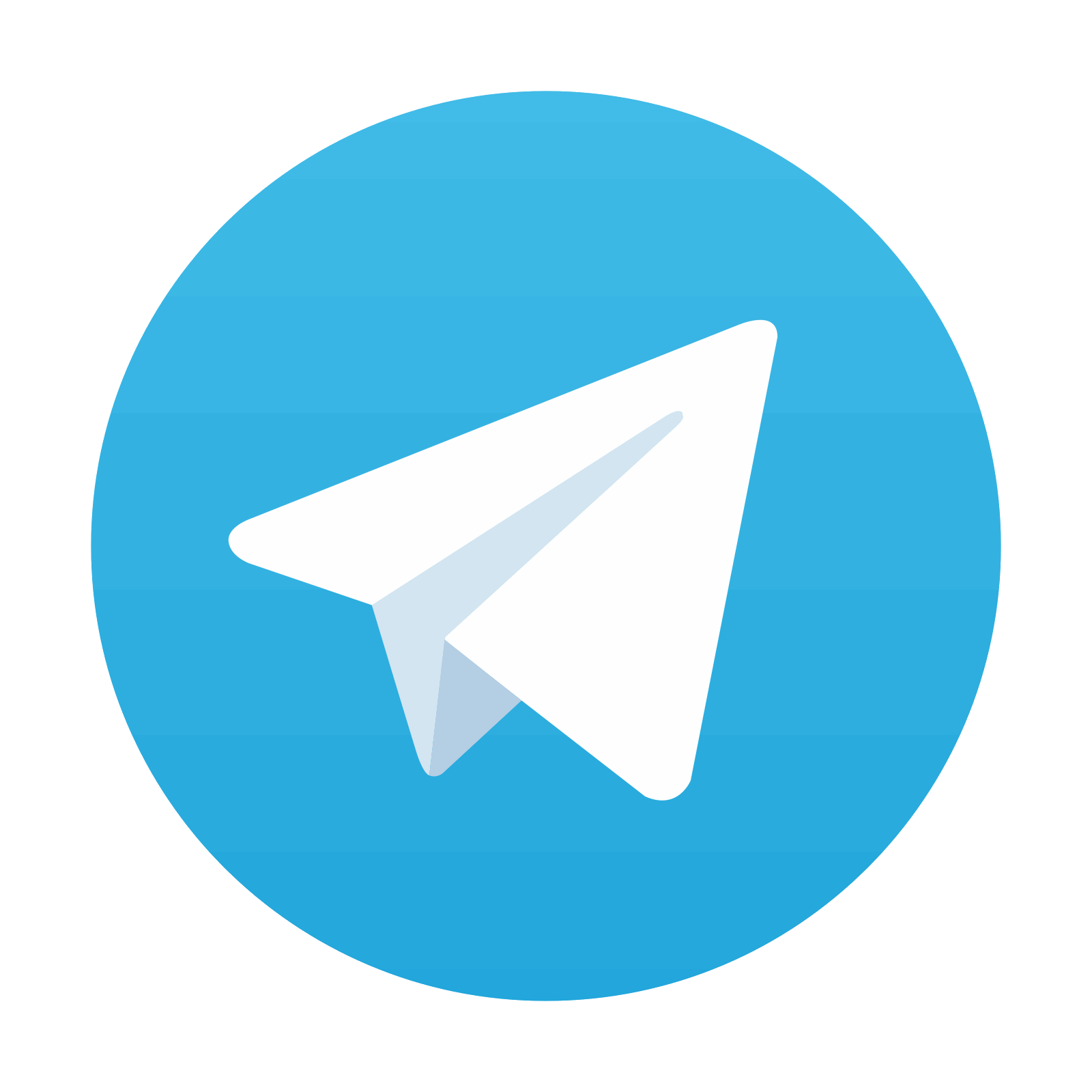
Stay updated, free articles. Join our Telegram channel

Full access? Get Clinical Tree
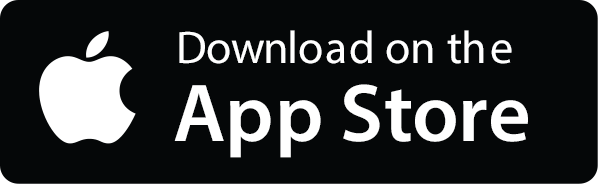
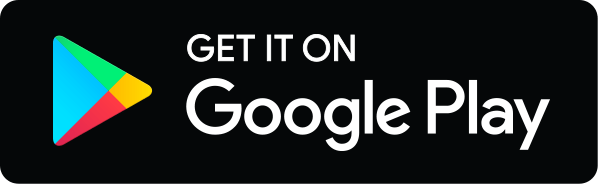