Fig. 12.1
Affibody-binding proteins: library and target binding. Illustration of the three-helix bundle affibody protein scaffold Z in band representation (green), with the 13 positions randomized during affibody protein library constructions highlighted (yellow) a. Side views b and top views c of the affibody protein scaffold showing the 13 surface-located positions in helices 1 and 2 employed for library constructions. d The structure of the complex between affibody proteins selected for binding to Taq DNA polymerase (ZTaq; white) and its anti-idiotypic affibody protein (anti-ZTaq; green with randomized positions in yellow). (Reproduced with permission from Ref. [10]. Copyright 2008 FEBS)
Affibody-binding proteins have no Ig origin and were functionally selected as a protein scaffold from libraries of a small and cysteine-free three-helix-bundle Z-domain [11]. Since Affibodies were first described, high-affinity Affibody proteins have been selected to bind assorted targets for various applications, such as bioseparation, functional inhibition, drug development, viral targeting, and in vivo targeted diagnostics and therapeutics [11]. With 13 amino acids as a binding sequence, Affibody proteins could potentially provide high-affinity binding proteins against different targets via protein engineering, and the small size of Affibody binders has been found to be advantageous for further modification, such as site-specific labeling and chelators’ conjugation.
12.2 Affibody Molecules Selection and Preparation
In recent years, numerous studies have examined the selection of Affibody proteins for molecular targeting. Currently, phage display technology has been introduced for all reported de novo selections of Affibody-binding proteins [12]. This technology was based on selection protocols and standard phage mid vectors. Other selection systems are also being investigated as alternative methods, including staphylococcal display, ribosomal display, microbead display, and b-lactamase-based protein fragment complementation [13]. The affinities obtained from Affibody proteins for different targets are similar to the affinities of antibody fragments or alternative scaffolds from similar native libraries. Different target structure characteristics and library complexity resulted in different dissociation constants (KD), ranging from micromolar to low picomolar. For example, the highest affinity for an Affibody target to the breast cancer marker ErbB2 was recently reported as only 22 pm [14]. The epidermal growth factor receptor (EGFR)-binding Affibody molecules with moderate affinity have also been isolated using phase-display selection technology. A study found that the molecules bind specifically to EGFR on EGFR-expressing cells in biosensors [15, 16]. Protein scaffolds can be prepared using either chemical or biological methods. For some small protein scaffolds, both methods can be used. After display selection, high-affinity scaffold molecules can be well established from the bulk of regular DNA templates. These DNA templates are produced using regular recombinant techniques. The high-affinity molecules exhibited high specificity and targeting capacity in protein engineering. For example, Affibodies can be prepared in E. coli, which makes the procedure easy and inexpensive compared with antibody production [17, 18]. In purification using a nickel column, a His-6 tag is typically added to the sequence of a scaffold protein when it is denatured [19]. This added His-6 can not only help purification but also achieve site-specific labeling with radionuclide 99mTc. More complicated post-transcription modifications can be either a disadvantage or advantage as is the case with glycosylation [19].
Small Affibody molecules can also be prepared directly by solid-phase peptide synthesis (SPPS), which is a desirable way to produce Affibody molecules with function groups for site-specifically labeling with a variety of reporters such as fluorophores, radionuclides, and nanoparticles [20, 21]. Synthesis of Affibodies using SPPS provides the freedom to label the protein with more than one reporter, and it facilitates the isolation of a homogeneous product when compared to recombinant production [21]. Furthermore, this approach could avoid the biological contaminants which largely exist in recombinant production. However, a limitation on this chemical approach is that the synthetic efficiency highly depends on the sequence and length of the Affibody proteins [22]. But the yield in organic synthesis of small proteins could be improved using a approach which involves the parallel synthesis of fragments and then assembling of them [23]. Importantly, it is feasible to perform chemical synthesis of the scaffold proteins without affecting the active amino acids or other functional group (Fig. 12.2) [20]. Manual modifications have been extensively applied to the synthetic Affibody proteins. For example, the resin-bound proteins have been manually modified by the introduction of thiol and biotin groups for surface immobilization (Fig. 12.2) [20].
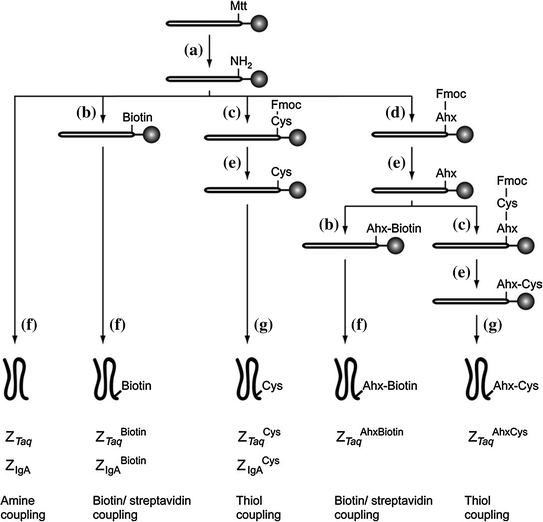
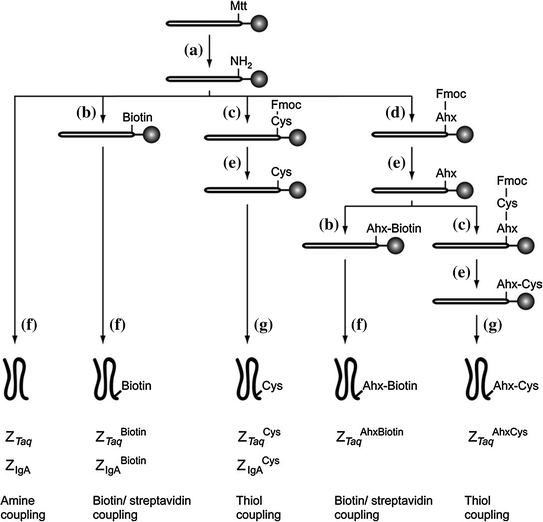
Fig. 12.2
Synthetic scheme for the preparation of five different Affibody variants: (a) TFA:TIS:DCM (1:5:94); (b) D-biotin, HBTU, HOBt, DIEA in NMP; (c) Fmoc-Cys(Trt)-OH, HBTU, HOBt, DIEA in NMP; (d) Fmoc-Ahx-OH, HBTU, HOBt, DIEA in NMP; (e) 20 % piperidine–NMP; (f) TFA:TIS:H2O (95:2.5:2.5); (g) TFA:TIS:EDT:H2O (94:1:2.5:2.5). (Reproduced with permission from Ref. [20]. Copyright 2005 Elsevier Inc.)
For the promotion the applications of Affibody, in addition to SPPS, combinatorial assembled Affibody molecules have been designed and prepared by native chemical ligation (NCL) [21, 22]. In Affibody molecules, the amino acids located in the N-terminal part of the protein (helices 1 and 2) determine the target-binding ability and specificity, whereas the C-terminal part (the third helix and its flanking regions) is preserved in all variants [24]. The distinct division of the protein sequence between variable and constant segment makes Affibody high tolerance toward scaffold modification. And then it makes a fragment condensation strategy very appealing. Thus, the concept of combinatorial assembling has been introduced for the synthesis of three different Affibody molecules. Specifically, the helice 3 of Affibody containing a cysteine residue located at the site of ligation is prepared and can be used for directed immobilization, and it has no unintentional interference to the function groups of the investigated proteins. Moreover, different C-terminal modifications to helice 3 are performed to improve yield in preparation of Affibody proteins [24]. Three different 2-helix Affibodies with specificity for IgG, insulin, or human epidermal growth factor receptor 2 (HER2) can then be coupled with helice 3 by NCL to produce the full Affibodies (Fig. 12.3) [23].
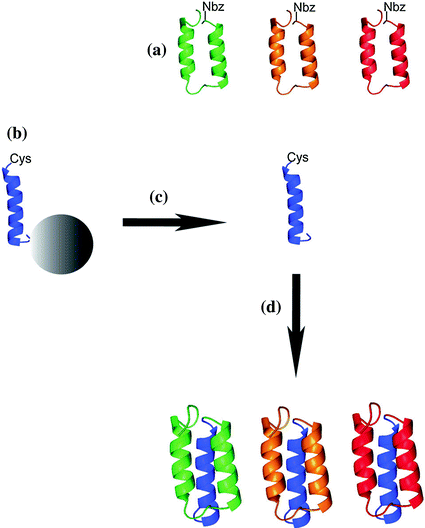
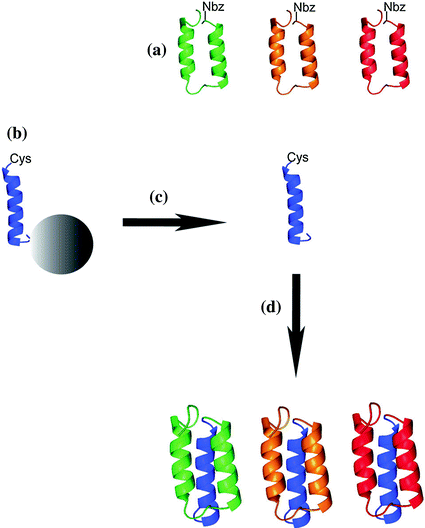
Fig. 12.3
Schematic overview of the combinatorial assembly approach by NCL for preparation of Affibody molecules (a): Selected Affibody molecules are determined by the N-terminal amino acid sequence that comprises helices 1 and 2. The C-terminal part contains helix 3 (b), and is constant in all Affibody molecules. Peptides are cleaved from the solid support (c), and NCL is used to yield the full-length Affibody molecules (d). (Reproduced with permission from Ref. [23]. Copyright 2012 Wiley–VCH Verlag GmbH& Co. KGaA, Weinheim)
12.3 Modification of Affibodies with Radionuclides
The most widely used molecular imaging agents in translational medicine are based on the radiolabeled molecules. It is very important to use appropriate labeling chemistry and find suitable radionuclides when developing protein based imaging probes or radiotherapeutics. Labeling chemistry, chosen for different radionuclides, is one of the most important factors in the successful engineering of new diagnostic or therapeutic proteins. It plays a substantial role in efficient radiolabeling and maintains the bioactivity of the molecules. For example, radionuclides can be used to label proteins and molecules directly by in situ oxidation [25–27]. This method is straightforward and typically results in efficient labeling. However, the function groups such as tyrosines may be critical for binding, and the directly labeling could results in low-binding affinity and targeting efficiency [26–28]. In some reports, anti-HER2 Affibody molecules labeled with radionuclides directly indeed show a loss of binding capacity in relation to the tyrosine modification in binding sites [25]. The inactivation of the HER2 Affibody molecule could be prevented using indirect labeling methods, such as site-specific modification with chelator or other moieties. Indirect labeling methods can consequently avoid the direct oxidization of protein and reduce the modification to the active amino groups of lysines and cisterns [25, 29]. Radionuclides selected for diagnosis and therapy highly depend on the properties of the targeting agent and its application. Physical half-life, the energy type of the emitted radiation, and the availability and cost of the nuclide must be considered. In addition, the requirements for matching the binder’s biological properties and targeting mechanisms with radionuclide’s physical properties should be considered. Different applications also require different radiation. For example, 18F, 11C, 13N, and 15O are the most common radionuclides for PET, whereas 99mTc and 111In are most often used for single-photon emission computed tomography (SPECT) [30, 31]. In these radionuclides, the physical half-lives of 11C, 13N, and 15O are too short to label peptides and proteins. Radionuclides with relatively longer half-lives, such as 68Ga and 89Zr, are used for PET imaging for targeted peptide or protein radiopharmaceuticals.
The cellular uptake and retention of radiolabeled molecules by cells and excretory organs are important to consider for the optimal design of an imaging probe. Different radionuclides (such as 125I and 111In) could impact targeting efficacy, imaging contrast, cellular protein degradation, and catabolism [30, 31]. For radio halogen-labeled biomolecules, after lysosomal degradation radionuclide catabolites are often quickly released from cells as uncharged lipophilic molecules. In contrast, radionuclides trapped in chelators are positively charged and typically hydrophilic and stay in cytoplasm for a long time. In addition, catabolism may occur in blood, excretory organs and even in tumor sites. As a result, the radio catabolites could re-enter the circulation and be redistributed [32]. This non-specific radioactivity would contribute to a high image background. Thus, the properties of radio catabolites should be considered when selecting a radionuclide for translational medicine engineering. Depending on their chemical nature, radio catabolites accumulated in the liver may be trapped inside hepatocytes or excreted into bile. This hepatobiliary excretion results in an increased background in the lower abdomen. Consequently, the behavior of a radiolabeled probe in excretory organs plays an important role in clinical performance and contributes to different imaging and therapy applications [33]. Increased uptake in the excretory organs generally indicates an undesirable elevated intake in healthy tissues. It has also been shown those labeled molecules smaller than 60 kDa are associated with high tumor retention of radioactivity but accompanied by a high accumulation of radioactivity in kidneys, because 60 kDa is the cutoff for glomerular filtration, and smaller proteins are mostly reabsorbed by the kidney [34, 35].
12.4 Affibody-based Molecular Imaging Techniques
12.4.1 Radionuclide Imaging
Two types of radionuclide imaging are in current use. One type is single-photon imaging, which includes SPECT and planar γ-camera imaging, and the other type is PET. Both planar imaging and SPECT are based on a γ camera, but in SPECT, the γ camera rotates around the target, avoiding the interfering radioactivity between the area of interest and the target [9, 36]. The molecular agents, which are clinically used for cancer imaging, are labeled with radionuclides such as 99mTc and 111In and imaged using a γ camera. PET imaging has developed rapidly in recent years. It enables better resolution and more accurate quantification compared with single-photon detection [36]. PET utilizes randomized detection of the pair of photons formed after positron annihilation and emitted by radionuclides. PET combined with computed tomography (CT) or magnetic resonance imaging (MRI) provides multimodality information and detailed anatomical fusion images with a higher degree of accuracy for both bone and soft tissue. The most frequently used PET probe is 18F-fluorodeoxyglucose (18F-FDG), which is used to assess glucose metabolism, e.g., in the brain and heart. Tumors often have a high metabolic activity and 18F-FDG uptake, which promotes tumor imaging using this probe [37, 38]. It has been reported that 18F-FDG-PET provides valuable diagnostic information and helps in the staging of lung cancer [37]. A limitation is the non-specific uptake in inflammatory sites, which may create a false-positive result [39]. In summary, PET imaging is superior to γ camera in terms of the imaging properties of resolution, sensitivity, and quantification. However, SPECT may currently be the most widely used molecular imaging technique because of the greater availability of SPECT diagnostic devices and the lower cost of the radiotracers [9].
Protein scaffolds have been used as a platform for molecular probe development for various imaging modalities, especially for SPECT and PET. Currently, Affibody molecules are the dominant protein scaffolds used in imaging translation. Affibody scaffolds typically exhibit high serum stability, fast and good uptake in target tissues, low uptake in other normal tissues and kidney clearance. A variety of HER2 Affibody (ZHER2) molecules constituted a large Affibody family have been reported [2, 40]. They have been labeled with 99mTc and 111In and used for planar scintigraphy and SPECT imaging. Several radiolabeled Affibody proteins, such as 99mTc-ZHER2:2395-C, 99mTc-maEEE-ZHER2:342, and 111
, provide easy radiolabeling and high serum stability. High-imaging contrast at 1 h post-injection (pi) was also demonstrated in vivo in a SKOV3 tumor model [40–42]. Among the numerous HER2 Affibody-based probes, 99mTc-ZHER2:2395-C has the highest tumor-to-blood ratio, high kidney accumulation, moderate liver retention, and low uptakes in all other organs [42]. In a LS174T colon cancer tumor model with moderate HER2 expression compared to SKOV3 tumor model, 99mTc-ZHER2:2395-C still exhibits good imaging properties in terms of the tumor-to-blood ratio (121 ± 24 at 4 h after injection) [42].

18F-FBO-ZHER2:477 and 18F-FBEM-ZHER2:342, the 18F-labeled HER2 targeting Affibody molecules, have also been labeled with site-specific methods [15]. These probes offer rapid uptake and high and specific accumulation as demonstrated by biodistribution and small animal PET imaging studies in HER2 overexpressing SKOV3 tumors. In addition, 18F-FBO-ZHER2:477 shows significantly higher tumor-to-normal tissue (blood, muscle, liver, and lung) ratios than18F-FBO-(ZHER2:477)2 at all the time points investigated in SKOV3 tumor-bearing mice. At 3 h after injection, the tumor-to-blood and tumor-to-muscle ratios of18F-FBO-ZHER2:477 are 12.72 ± 2.94 and 28.03 ± 8.28, respectively [37]. Relatively fast clearance from the kidneys has also been observed in these probes, demonstrating that 18F-labeled HER2 targeting Affibody molecules are promising new type of HER2 PET probe. More importantly, one of the HER2-targeting Affibody molecules, 18F-FBZM-ZHER2:342 can detect and quantify HER2 expression changes through PET imaging. 18F-FBZM-ZHER2:342 enhanced the accuracy of HER2 targeted probes in tumor molecular imaging [43]. Other HER2-targeting probes, such as 124I-, 64Cu-labeled ZHER2, and optical dye (such as Alexa-750)-labeled ZHER2-ABD fusion proteins, have also been evaluated for PET imaging in a SKOV3 tumor model [43, 44]. The imaging contrast is excellent for tumors observed at 6–24 h pi, and most of probe is cleared out by 24 h pi except from the kidney [44].
The EGFR-targeted Affibody molecules (ZEGFR) have also been developed for molecular imaging. 111In-Bz-DTPA-ZEGFR:1907 and 111In-Bz-DTPA-(ZEGFR:1907)2 have exhibited significantly higher contrast in EGFR-positive tumors than in negative ones [45]. More interestingly, 111In-Bz-DTPA-ZEGFR:1907 presents results similar to those of radiolabeled HER2 Affibody-based probes in vivo, such as quick tumor uptake, specific targeting, and high accumulations and rapid clearance from the kidneys [45, 46]. 64Cu-labled ZEGFR:1907 has also been evaluated with PET in an A431 tumor model. This probe exhibits good contrast in tumors with target-positive expression, and it displays fast clearance through the liver and kidneys at an early time point p.i. Another more clinical relevant probe, 18F-labeled Ac-Cys-ZEGFR:1907 has been developed and this novel protein scaffold–based PET probes able to image EGFR overexpressing tumors. It can also differentiate tumors with high and low EGFR expression in vivo and hold high promise for future clinical translation (Fig. 12.4a) [47]. Lastly, therapeutic agent, 177Lu-DO3A-HSA-ZEGFR:1907, has been prepared and show as a potential radiopharmaceutical for radionuclide therapy of EGFR-expressing head and neck carcinomas (Fig. 12.4b) [48].
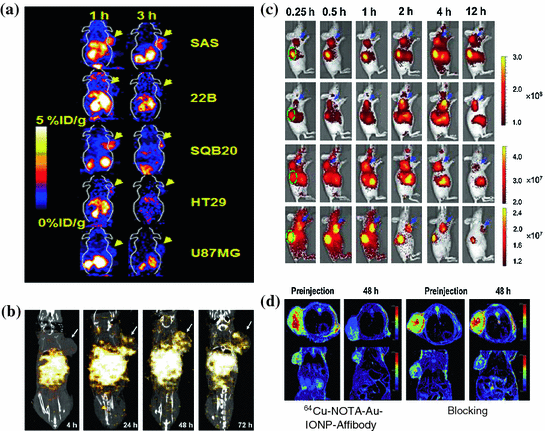
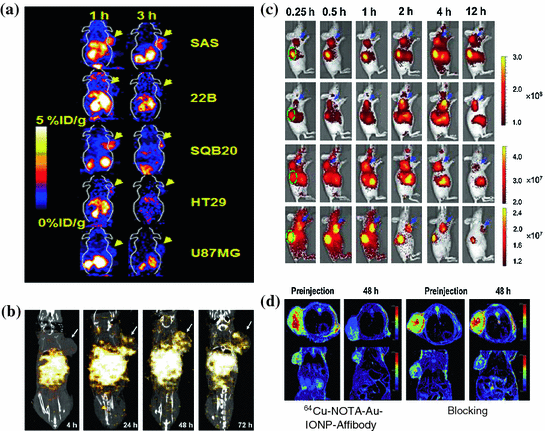
Fig. 12.4
Representative multimodality imaging using Affibody-based probes. a 18F-FBEM-Cys-ZEGFR: 1907 PET of EGFR overexpression xenograft models. b SPECT/CT imaging of a mouse bearing SAS tumor xenograft at 4, 24, 48, and 72 h after administration of 177Lu-DO3A-HSA-ZEGFR:1907. Arrows indicate the location of tumors. White color indicates the highest radioactivity accumulation. c In vivo fluorescence imaging of subcutaneous A431 tumor-bearing nude mice at 0.25, 0.5, 1, 2, 4, and 12 h after injection of 0.5 nmol Cy5.5-ZEGFR:1907 (1), Alexa680-ZEGFR:1907 (2), SR680-ZEGFR:1907 (3), or 800CW-ZEGFR:1907 (4). Arrows in blue color and ovals in green color indicate the location of tumors and kidneys, respectively. d In vivo T2-weighted MR images of A431 tumor-bearing mice acquired before and at 48 h after injection of 64Cu-NOTA-Au-IONP-Affibody and blocking dose of Affibody. (Reproduced with permission from (a) [47] copyright 2012 Society of Nuclear Medicine and Molecular Imaging, Inc. (b) [48] copyright 2012 SBIC (c) [53] copyright 2012 American Chemical Society (d) Ref [55]. Copyright 2013 Elsevier Ltd)
12.4.2 Optical Imaging
SPECT and PET involve the use of highly ionizing materials for imaging, and the imaging systems are also expensive and complicated to use. In addition, production of some short half-life PET radionuclides such as 18F requires a cyclotron. Therefore, nuclear imaging can usually only be set up in large clinical or research facilities. In contrast, optical imaging is simple to set up, safe, and does not require dedicated instruments. Optical imaging uses charge coupled device (CCD) cameras to collect the signals originating from fluorescent and bioluminescent probes [49–51]. Because of its many favorable properties including cheap, wide availability, non-ionization and minimal invasiveness, optical imaging presents an attractive option for tumors imaging and therapeutic monitoring. Recently, an Affibody-based, HER2-specific fluorescence probe was introduced to monitor the change of the target signal in the process of treatment in vivo of the receptors [52]. Subcutaneous tumor xenografts, which express different levels of HER2, were imaged with a NIR fluorescence small animal imaging system and linearly depends on HER2 expression as measured by an ELISA assay ex vivo for the same tumor, at several times post-injection of the probe [52]. This work suggests that optical imaging, combined with mathematical modeling, may monitor the molecular expression and then further allow non-invasive monitoring of HER2 expression in vivo using an Affibody-based optical probe. Moreover, several ZEGFR:1907 based Affibody optical probes have been prepared through conjugation the ZEGFR:1907 with different optical dyes. EGFR-positive tumors can be clearly delineated using these four probes [53] (Fig. 12.4c). These works have laid down solid foundation for using Affibody-based probes for tumor optical imaging and image-guided surgery.
12.4.3 MRI
MRI provides high spatial resolution and excellent soft-tissue contrast without any radiation when compared with PET and SPECT, making it one of the best modalities for soft-tissue imaging and tumor diagnosis. MRI can be further combined with appropriate imaging contrast agents for certain molecular targeted diagnosis. It also provides multiplane capabilities and high-quality 3D anatomic data. A nanoparticle combining the biotinylatedanti-HER2 Affibody molecules and streptavidin functionalized super paramagnetic iron oxide (SPIO) has been reported to image HER2-positive SKOV-3 tumor [54]. The nanoparticles bind specifically to HER2 after the administration of the nanoconstruct, and the tumor could be clearly imaged using a gradient-echo sequence on MRI [54]. In another report, 64Cu-NOTA-Au-IONP-ZEGFR:1907 has been used for EGFR-targeted MRI imaging when the Affibody molecules combined with gold iron oxide nanoparticle [55] (Fig. 12.4d). These studies demonstrate that combined Affibody-IO can be used for targeted in vivo tumor MRI.
12.5 Application of Affibodies in Biomedical Research
12.5.1 Affibody Molecule-based Tumor Imaging
The use of molecular imaging in clinics depends on its accuracy, which includes the sensitivity and specificity of the imaging agent. A good imaging agent should be sensitive to the target. The transport path, such as blood circulation or local diffusion in tissues, is also important and contributes to accumulation or retention in the target tissue [8, 56]. The sensitivity of the molecular imaging agent is mainly determined by the contrast between the radioactivity concentration in the tumor and normal healthy tissues. Therefore, an imaging agent is desired to be of small size and soluble to allow for rapid tumor tissue penetration and accumulation, and it should also bind specifically to a molecular target with minimal non-specific binding. Moreover, molecular targeted agents should be cleared quickly from the blood and other background organs by kidney excretion [56–58]. Rapid clearance is important in providing a good imaging contrast in a short period of time and minimizing clinical protocols. Other factors influencing the accuracy of imaging agents are the target expression level, the binding and retention of the probe to the target, the vascularization of tumor, and the device used to optimize resolution and sensitivity [56, 58]. Affibody-based probes possess many desirable features and thus have been extensively studied for imaging several important tumor targets in pre-clinical and clinical studies.
ErbB receptors are involved in the development of many human cancers, such as breast, prostate, head and neck and colon cancer [59, 60]. It has been further reported that alterations of ErbB receptors showed more aggressive disease with a poor prognosis in patients [60–62]. EGFR (also known as ErbB1 or HER1), ErbB2 (or HER2), ErbB3 (or HER3), and ErbB4 (or HER4) are four members of the ErbB family of Receptor Tyrosine Kinases (RTKs) [16, 63–65]. All ErbB receptors have three common parts: an extracellular ligand-binding domain, a single membrane—spanning region and a cytoplasmic protein TK (tyrosine kinase) domain [16, 63–65]. The expression of the ErbB receptor can be found in epithelial, mesenchymal and neuronal origin, and the expression of the ligands physiologically controls the activation of the ErbB receptors [66]. Importantly, it has been found that the expression of RTKs, EGFR, and HER2 is up-regulated in various solid tumors. These biomarkers usually correlate with the tumor cells proliferation and metastasis and therefore serve as important targets for tumor molecular imaging. Monitoring their expression could potentially facilitate targeted therapy or monitor the therapeutic response [67].
12.5.1.1 HER2
The HER2 receptor has been widely investigated for cancer targeted therapy. A humanized IgG1mAb, herceptin (trastuzumab), has been widely used for the treatment of metastatic breast cancer patients who showed over-expressed HER2 [68–72]. It thus important to develop HER2 imaging agent for the purpose of patient prognosis and stratification for personalized therapy. Anti-HER2 antibody derivatives have been used for HER2 targeted imaging using the modality of PET [73] and a gamma-camera [73]. However, it requires long time (days) to achieve good imaging contrast. High-affinity Affibody molecules have been extensively explored for HER2 targeting in many pre-clinical studies and a pilot clinical study [14]. Particularly, one of anti-HER2 Affibody variants, ZHER2:342, exhibit several beneficial properties, including high stability and affinity, good solubility, and good tumor uptake, making it a promising protein for further development. Orlova et al. describe that 125I-labelled ZHER2:342 exhibit a tumor uptake of approximately 9 % ID/g at 4 h pi and a ratio (tumor/muscle) of 38:1 in SKOV-3 tumor models in vivo [74]. It also exhibits higher contrast in tumors 6 h pi in γ-camera imaging. For SPECT imaging, 111In-benzyl-DTPA-ZHER2:342 exhibits specificity to the HER2-positive-expressing cell line SKOV-3 [75, 76]. High tumor uptake has been found at 4 h after the injection of 111In-benzyl-DTPA-ZHER2:342 SKOV-3 tumors in vivo [75, 76]. Moreover, it can be cleared out quickly from blood, and the tumor/blood ratio is approximately 100:1 at 4 h pi. High uptake is observed in the kidneys and ascribed to the properties of 111In [41].
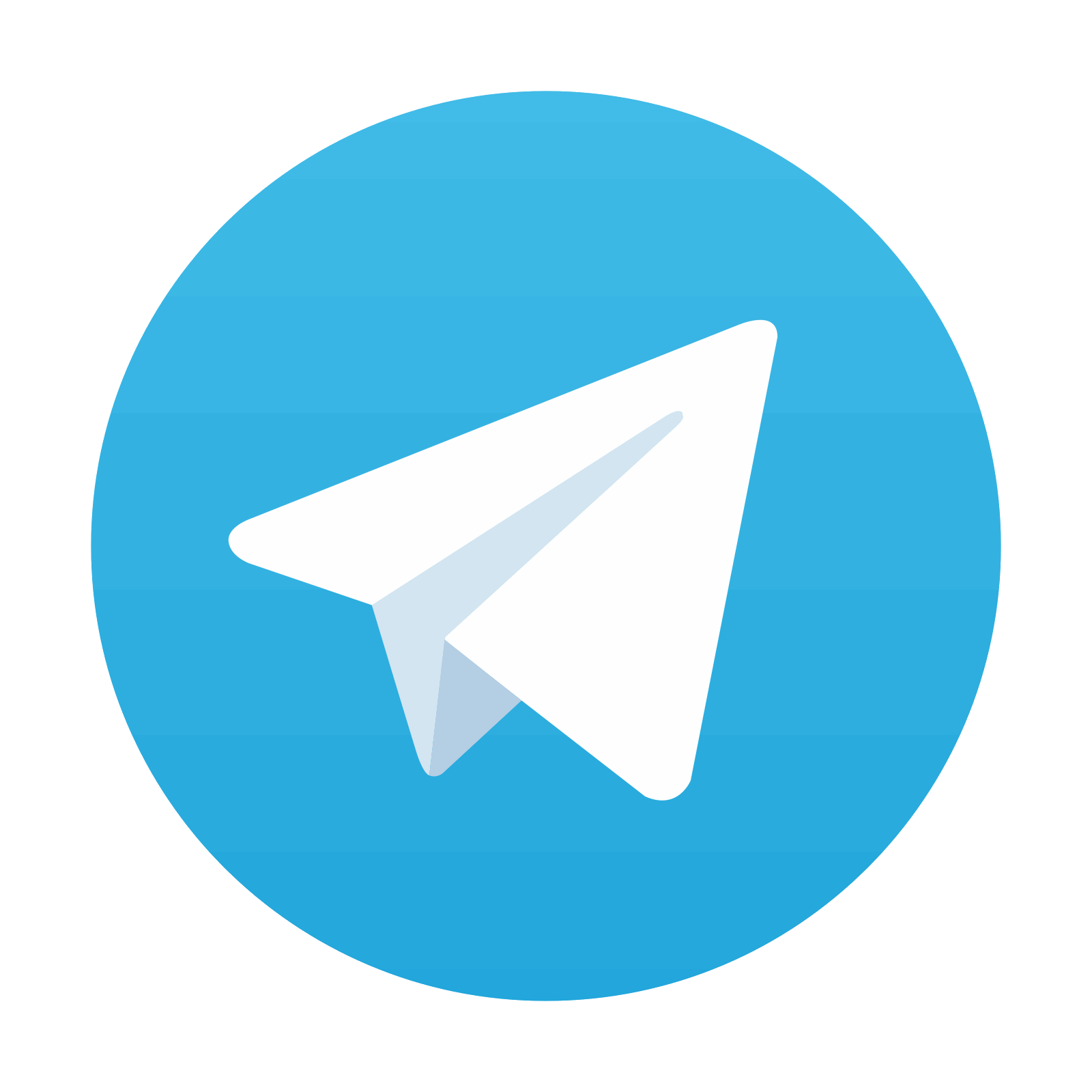
Stay updated, free articles. Join our Telegram channel

Full access? Get Clinical Tree
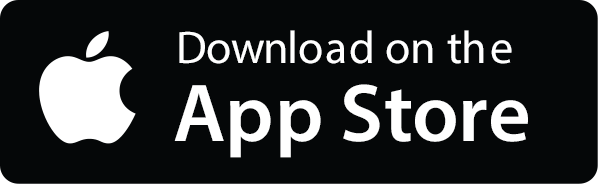
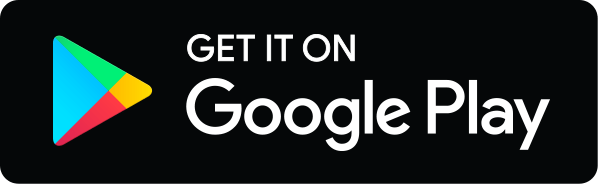