osmoreceptors
Carotid sinus
Afferent arteriole
Atria
AVP
Thirst
Sympathetic nervous system
Renin-angiotensin-aldosterone system
ANP/BNP
AVP
What is affected
Urine osmolality
Water intake
Urinary sodium excretion
Vascular tone
NOTES: See text for details. AVP = arginine vasopressin; ANP = atrial natriuretic peptide; BNP = brain natriuretic peptide.
SOURCE: Adapted from Rose BD, Black RM. Manual of Clinical Problems in Nephrology, p. 4. New York: Little Brown & Co.; 1988.
Figure 58.1. Vasopressin and the Regulation of Water Permeability in the Renal Collecting Duct. Vasopressin binds to the type 2 vasopressin receptor (V2R) on the basolateral membrane of principal cells, activates adenylyl cyclase (AC), increases intracellular cyclic adenosine monophosphatase (cAMP), and stimulates protein kinase A (PKA) activity. Cytoplasmic vesicles carrying aquaporin-2 (AQP) water channel proteins are inserted into the luminal membrane in response to vasopressin, thereby increasing the water permeability of this membrane. When vasopressin stimulation ends, water channels are retrieved by an endocytic process and water permeability returns to its low basal rate. The AQP3 and AQP4 water channels are expressed on the basolateral membrane and complete the transcellular pathway for water reabsorption. pAQP2 = phosphorylated aquaporin-2. SOURCE: From Sands JM, Bichet DG. Nephrogenic diabetes insipidus. Ann Int Med. 2006;144:186–194.
Volume status also modulates the release of AVP by the posterior pituitary, such that hypovolemia is associated with higher circulating levels of the hormone at each level of serum osmolality; hypovolemia can thus be associated with hyponatremia due to retention of ingested free water in response to increased AVP. Similarly, in the setting of reduced effective circulating volume and impaired arterial circulatory integrity, as seen in cirrhosis and heart failure, the associated neurohumoral activation leads to an increase in circulating AVP, predisposing to hyponatremia. These interactions between volume status, AVP release, and water homeostasis can lead to diagnostic confusion, which can be lessened by considering the relevant physiology (see table 58.1). A key concept in this regard is that the absolute serum [Na+] conveys no diagnostic information as to the volume status of a given patient, with hyponatremia in particular occurring at all extremes of whole-body water and Na+-Cl– content.
HYPONATREMIA
Diagnostic Approach
Hyponatremia, defined as a serum [Na+] <135 mEq/L, is a very common disorder occurring in up to 22% of hospitalized patients. This disorder is almost always the result of an increase in circulating AVP and/or increased renal sensitivity to AVP, combined with an intake of free water. A notable exception is hyponatremia due to low solute intake, as can occur with extreme vegan diets or “beer potomania,” wherein urinary solute concentrations are inadequate to support the excretion of ingested free water; the reduced capacity for renal water excretion is easily overwhelmed in these patients, leading to water retention and hyponatremia.
The underlying pathophysiology for the typical exaggerated or inappropriate vasopressin response differs in patients with hyponatremia as a function of their extracellular fluid volume. Hyponatremia is thus subdivided diagnostically into three groups, depending on clinical history and volume status: hypovolemic, euvolemic, and hypervolemic hyponatremia (see Figure 58.2). Notably, hyponatremia is frequently multifactorial, particularly when severe; clinical evaluation should examine all the possible causes for increased AVP including nausea, pain, and drugs.
Laboratory investigation of a patient with hyponatremia should always include a measurement of serum osmolality to exclude pseudohyponatremia, hyponatremia with a normal or increased plasma tonicity. Most clinical laboratories measure serum [Na+] by testing diluted samples with automated ion-sensitive electrodes, correcting for this dilution by assuming that plasma is 93% water. This correction factor can be inaccurate in patients with pseudohyponatremia due to extreme hyperlipidemia and/or hyperproteinemia, in whom serum lipid or protein makes up a greater percentage of plasma volume. The measured osmolality should also be converted to the effective osmolality (tonicity) by subtracting the measured concentration of urea (divided by 2.8 if in mg/dL); patients with hyponatremia have an effective osmolality of <275 mOsm/kg. Serum glucose should also be measured; serum [Na+] falls by ~1.4 mEq/L for every 100 mg/dL increase in glucose, due to glucose-induced water efflux from cells; this form of hyponatremia resolves with normalization of serum glucose.
Figure 58.2. The Diagnostic Approach to Hyponatremia. See text for details. SOURCE: From Kumar S, Berl T. Diseases of water metabolism. In Schrier RW (ed.). Atlas of Diseases of the Kidney, Vol. 1. New York: Wiley; 1999.
Urine electrolytes and osmolality are particularly critical tests in the initial evaluation of hyponatremia. A urine [Na+] <20 mEq is consistent with hypovolemic hyponatremia, in the clinical absence of a hypervolemic Na+-avid syndrome such as congestive heart failure (CHF) or cirrhosis (see Figure 58.2). Urine osmolality <100 mOsm/kg is suggestive of polydipsia. A urine osmolality >400 mOsm/kg indicates that AVP excess is playing a more dominant role, whereas intermediate values are more consistent with multifactorial pathophysiology (e.g., AVP excess with a component of polydipsia). Patients with hyponatremia due to decreased solute intake, as in “beer potomania,” typically have urines with [Na+] <20 mEq and urine osmolality in the range of <100 to the low 200s.
Measurement of serum uric acid should also be performed; whereas patients with SIADH-type physiology will typically be hypouricemic (serum uric acid <4 mg/dL), volume-depleted patients will often be hyperuricemic. Finally, in the right clinical setting, thyroid, adrenal, and pituitary function should also be tested. Hypothyroidism and secondary adrenal failure due to pituitary insufficiency are important causes of euvolemic hyponatremia, whereas primary adrenal failure causes hypovolemic hyponatremia. Radiological imaging should also be considered, looking for a pulmonary or CNS cause for inappropriate AVP secretion and hyponatremia; patients with a history of smoking should undergo chest CT to rule out SIADH-associated small cell lung carcinoma, which can sometimes be missed on routine chest radiographs.
Hypovolemic Hyponatremia
Hypovolemia causes marked neurohumoral activation, inducing systems such as the renin-angiotensin-aldosterone axis (RAA), the sympathetic nervous system, and circulating AVP (see table 58.1). The increase in circulating AVP helps preserve blood pressure via vascular and baroreceptor V1A receptors, and increases water reabsorption via renal V2 receptors; the latter effect can lead to hyponatremia, in the setting of increased free water intake. Nonrenal causes of hypovolemic hyponatremia include GI (vomiting, diarrhea, tube drainage, etc.) and insensible loss of Na+-Cl– (sweating, burns, respiratory tract); urine [Na+] is typically <20 mEq in these cases. These patients may be clinically classified as euvolemic, with only the reduced urine [Na+] to indicate the cause of their associated hyponatremia.
The renal causes of hypovolemic hyponatremia share an inappropriate loss of Na+-Cl– in the urine leading to volume depletion; urine [Na+] is typically >20 mEq (Figure 58.2). The associated deficiency in circulating aldosterone can lead to hyponatremia in primary adrenal insufficiency and other causes of hypoaldosteronism; hyperkalemia and hyponatremia in a hypotensive and/or hypovolemic patient with high urine [Na+] should strongly suggest this diagnosis. Salt-losing nephropathies are characterized by impaired renal tubular function and thus a reduced ability to reabsorb filtered Na+-Cl–, leading to hypovolemia and neurohumoral activation. Typical causes include reflux nephropathy, interstitial nephropathies, cisplatin-associated kidney injury, postobstructive uropathy, medullary cystic disease, and the recovery phase of acute tubular necrosis. Diuretic therapy, particularly with thiazides, causes hyponatremia via a number of mechanisms. Increased excretion of an osmotically active nonreabsorbable or poorly reabsorbable solute can also lead to volume depletion and hyponatremia; important causes include glycosuria, ketonuria, and bicarbonaturia (e.g., in proximal renal tubular acidosis, where the associated bicarbonaturia leads to loss of Na+). Finally, the syndrome “cerebral salt wasting” is a rare cause of hypovolemic hyponatremia due to inappropriate natriuresis in association with intracranial disease; causative disorders include subarachnoid hemorrhage, traumatic brain injury, craniotomy, encephalitis and meningitis. Distinction from the syndrome of inappropriate antidiuresis (SIADH) is difficult but critical for successful management, since cerebral salt wasting will typically respond to aggressive Na+-Cl– repletion.
Hypervolemic Hyponatremia
Patients with hypervolemic hyponatremia develop an increase in total body Na+-Cl– that is accompanied by a proportionately greater increase in total body water, leading to a reduced serum [Na+]. Again, the causative disorders can be separated by the effect on urine [Na+], with acute or chronic renal failure uniquely associated with an increase in urine [Na+] (see Figure 58.2); advanced renal insufficiency can reduce the ability to excrete free water, leading to hyponatremia. The pathophysiology of hyponatremia in the sodium-avid edematous disorders (CHF, cirrhosis, and nephrotic syndrome) is similar to that in hypovolemic hyponatremia except that the arterial perfusion pressure is decreased due to the specific etiologic factors, such as cardiac dysfunction in CHF. Urine [Na+] is typically very low (i.e., <10 mEq); this Na+ -avid state may be obscured by diuretic therapy depending on the timing of sample collection, schedule and choice of diuretics, and so forth. The degree of hyponatremia is an indirect index of the associated neurohumoral activation (see table 58.1) and thus an important prognostic indicator in hypervolemic hyponatremia. Management consists of treating the underlying disorder (e.g., ACE-inhibition in heart failure), Na+ restriction, diuretic therapy, and, when appropriate, H2O restriction. Vasopressin antagonists (vaptans) are effective in normalizing hyponatremia associated with both cirrhosis and CHF. However, tolvaptan is contraindicated in cirrhosis due to drug-associated liver toxicity and should not be utilized for longer than 30 days in patients with CHF. Conivaptan, a mixed V1a/V2 receptor antagonist, must also be used with caution in cirrhosis given the potential for hypotension and/or renal insufficiency due to blockade of V1a vasopressin receptors.
Euvolemic Hyponatremia
The syndrome of inappropriate antidiuresis (SIADH) is the most common cause of euvolemic hyponatremia (see table 58.2). Other causes include hypothyroidism and secondary adrenal insufficiency due to pituitary disease; whereas the deficit in circulating aldosterone in primary adrenal insufficiency causes hypovolemic hyponatremia, the predominant glucocorticoid deficiency in secondary adrenal failure leads to euvolemic hyponatremia. Common causes of SIADH include pulmonary disease (e.g., pneumonia, tuberculosis, pleural effusion) and CNS diseases (e.g., tumor, subarachnoid hemorrhage, meningitis); SIADH also occurs with malignancies, most commonly small cell lung carcinoma, and drugs—most commonly the selective serotonin reuptake inhibitors (SSRIs; see table 58.2).
The initial treatment of euvolemic hyponatremia should include treatment or withdrawal of the underlying cause if feasible and appropriate. Water restriction to <1 L/d is a cornerstone of therapy but may be ineffective or poorly tolerated; thirst is also stimulated in these patients at lower than the usual “physiological” osmolalities. Patients who fail to respond to water restriction can be treated with loop diuretics to inhibit the countercurrent mechanism and reduce urinary concentration, combined with oral salt tablets to replace diuretic-induced salt loss. Historically, oral demeclocycline has been used to treat SIADH that fails water restriction or lasix/salt tablets; this agent can, however, cause acute kidney injury and necessitates close follow-up of renal function. Vaptans are highly effective at normalizing serum [Na+] in almost all patients with SIADH. Conivaptan, the only available intravenous vaptan in the United States, can be utilized as an alternative to hypertonic saline for maintaining eunatremia in critically ill patients with SIADH. However, oral tolvaptan (currently the only available oral vaptan in the United States) should not be utilized for more than 30 days and should not used in patients with liver disease given the risk of liver toxicity. Given these limitations, therapy with tolvaptan should be reserved for SIADH patients who have serum [Na+] that remains <120 mEq or who have persistent neurological symptoms attributed to hyponatremia.
Treatment of Hyponatremia
Three important considerations guide the therapy of hyponatremia. First, the presence and/or severity of symptoms determine the urgency of therapy. Patients with acute hyponatremia (box 58.1) present with symptoms that can include headache, nausea and/or vomiting, altered mental status, seizures, obtundation, and/or death. Patients with chronic hyponatremia (present for >48 hours) are less likely to be symptomatic but may demonstrate subtle deficits in neuropsychological function including gait abnormalities and an increased risk of falls. Chronic hyponatremia also increases the risk of bony fractures due to the increased risk of falls and to a hyponatremia-associated reduction in bone density. Second, patients with chronic hypontremia are at risk for osmotic demyelination syndrome (typically central pontine myelinolysis) if serum [Na+] is corrected by >10–12 mEq within the first 24 hours and/or by >18 mEq within the first 48 hours. Brain cells in chronic hyponatremia reduce the intracellular concentration of organic osmolytes (creatine, betaine, glutamate, and taurine) to cope with hypo-osmolality. The intracellular reaccumulation of these solutes is attenuated and delayed after reestablishment of normal tonicity, leading to osmotic demyelination in the setting of overly rapid correction of hyponatremia. Third, the response of the serum [Na+] to interventions such as hypertonic saline or vasopressin antagonists can be highly unpredictable, such that frequent monitoring of serum [Na+] (every 2–4 hours) is required during therapy with these measures.
Box 58.1 CAUSES OF ACUTE HYPONATREMIA
Iatrogenic
Postoperative—premenopausal women
Hypotonic fluids with cause of ↑ vasopressin
Glycine irrigant—TURP, uterine surgery
Colonoscopy preparation
Recent institution of thiazides
Polydipsia
MDMA (“Ecstasy”) ingestion
Exercise induced
Multifactorial, e.g., thiazide and polydipsia
NOTES: MDMA = 3,4-methylenedioxymethamphetamine (“Ecstasy”); TURP = transurethral resection of the prostate.
Acute symptomatic hyponatremia can occur in several clinical settings (box 58.1). This syndrome is a medical emergency; a sudden drop in serum [Na+] can overwhelm the capacity of the brain to regulate cell volume, leading to massive cerebral edema. Notably, this may occur after relatively modest acute reductions in serum [Na+]. Premenopausal women are particularly prone to severe symptoms of acute hyponatremia; neurological consequences are comparatively rare in male patients. A critical and often overlooked complication is respiratory failure, which may be hypercapneic due to CNS depression or normocapneic due to neurogenic, noncardiogenic pulmonary edema; the associated hypoxia amplifies the impact of hyponatremic encephalopathy. Many of these patients develop hyponatremia from iatrogenic causes, including hypotonic fluids in the postoperative period, prescription of a thiazide diuretic, colonoscopy preparation, or intraoperative use of glycine irrigants. Polydipsia occurring with a cause of increased AVP may also cause acute hyponatremia, as with increased water intake in the setting of strenuous exercise (e.g., marathon-associated hyponatremia). The recreational drug Ecstasy (3,4-methylenedioxymethamphetamine, MDMA) can also cause acute hyponatremia, rapidly inducing both AVP release and increased thirst.
Treatment of acute symptomatic hyponatremia should include hypertonic saline to acutely increase serum [Na+] by 1–2 mEq/hour to a total increase of 4–6 mEq; this increase is typically sufficient to alleviate acute symptoms, after which corrective guidelines for chronic hyponatremia are appropriate (see below). A number of equations have been developed to estimate the required rate of hypertonic solution; one popular approach is to calculate a “Na+ deficit,” where the Na+ deficit = 0.6 × body weight × (target [Na+] – starting [Na+]). However, a major caveat is that the increase in serum [Na+] can be highly unpredictable during treatment with hypertonic saline, due to rapid changes in the underlying physiology; serum [Na+] should be monitored every 2–4 hours with appropriate adjustments in the rate of administered saline. In hypokalemic patients, K+-Cl– replacement can also lead to an increase in serum [Na+], given that serum [Na+] is a function of exchangeable Na+ and K+, divided by whole body water; this phenomenon can also lead to an overly rapid correction in serum [Na+] in chronic hyponatremia (see below).
The administration of supplemental oxygen and ventilatory support can also be critical in acute hyponatremia in the event that patients develop acute pulmonary edema or hypercapneic respiratory failure. Intravenous loop diuretics will help treat acute pulmonary edema and will also increase free water excretion, by interfering with the renal countercurrent multiplication mechanism. It should be emphasized that vaptans do not have a role in the management of acute symptomatic hyponatremia.
The management of chronic hyponatremia is complicated significantly by the asymmetry of the cellular response to correction of serum [Na+]. Specifically, the reaccumulation of protective organic osmolytes by brain cells is attenuated and delayed as osmolality increases after correction of hyponatremia, sometimes resulting in degenerative loss of oligodendrocytes and the “osmotic demyelination syndrome” (ODS). Overly rapid correction of hyponatremia is also associated with a disruption in integrity of the blood brain barrier, allowing the entry of immune mediators that may contribute to demyelination in ODS. The lesions of ODS classically affect the pons; clinically, patients with “central pontine myelinolysis” can present one or more days after overcorrection of hyponatremia with para- or quadraparesis, dysphagia, dysarthria, diplopia, a “locked-in syndrome,” and/or loss of consciousness. Other regions of the brain can also be involved; clinical presentation of ODS varies as a function of the extent and localization of this extrapontine myelinolysis, with the reported development of ataxia, mutism, parkinsonism, dystonia, and catatonia. “Re-lowering” of serum [Na+] after overly rapid correction can prevent or attenuate ODS. However, even appropriately “slow” correction can be associated with ODS, particularly in patients with additional risk factors; these include alcoholism, malnutrition, hypokalemia, and liver transplantation.
To reduce the risk of ODS the rate of correction should be comparatively slow in chronic hyponatremia, no greater than 10–12 mEq in the first 24 hours and no greater than 18 mEq in the first 48 hours, but preferably much less of an increase. Should patients overcorrect serum [Na+] in response to vasopressin antagonists, hypertonic saline, or isotonic saline (in chronic hypovolemic hyponatremia), hyponatremia can be safely reinduced or stabilized by the administration of the vasopressin agonist desmopressin acetate (DDAVP) and the administration of free water, typically intravenous D5W. In patients with marked initial hyponatremia, a more linear, controlled increase in serum [Na+] can be achieved by utilizing high-dose DDAVP to “clamp” vasopressin activity, in combination with a slow infusion of hypertonic saline.
HYPERNATREMIA
Hypernatremia is usually the result of a combined water and volume deficit, with losses of H2O in excess of Na+. Elderly individuals with reduced thirst and/or diminished access to fluids are at the highest risk of developing hypernatremia. Patients with hypernatremia may rarely have a central defect in hypothalamic osmoreceptor function, with a mixture of both decreased thirst and reduced AVP secretion; causes include primary or metastatic tumor, occlusion or ligation of the anterior communicating artery, trauma, hydrocephalus, and inflammation. More commonly, hypernatremia develops following the loss of water via renal or nonrenal routes, combined with a reduced intake of water. “Insensible losses” of water due to evaporation from the skin or respiratory tract may increase in the setting of fever, exercise, heat exposure, severe burns, or mechanical ventilation. Diarrhea is in turn the most common gastrointestinal cause of hypernatremia. Osmotic diarrhea and viral gastroenteritides typically generate stools with Na+ and K+ <100 mEq, thus leading to water loss and hypernatremia; secretory diarrheas typically result in isotonic stool and hypovolemia +/– hypovolemic hyponatremia.
Common causes of renal water loss include osmotic diuresis secondary to hyperglycemia, postobstructive diuresis, or drugs (e.g., mannitol). Water diuresis per se occurs in central or nephrogenic diabetes insipidus (DI). The various causes of central and nephrogenic diabetes insipidus are listed in box 58.2. Nephrogenic diabetes insipidus is most commonly due to therapy with lithium, which inhibits the renal response to AVP and can cause chronic distal tubular injury. Gestational diabetes insipidus is a rare complication of pregnancy wherein increased activity of a placental protease with “vasopressinase” activity leads to reduced circulating AVP; DDAVP is an effective therapy, given its resistance to the enzyme. Finally, the ingestion or iatrogenic administration of excess Na+ is a rare cause of hypernatremia, typically occurring with the IV administration of excess hypertonic Na+-Cl– or Na+-HCO3–.
Box 58.2 CAUSES OF DIABETES INSIPIDUS
Central diabetes insipidus
Pituitary surgery
Head trauma
Tumors
Cerebrovascular event or hypoxic encephalopathy
Infections
Idiopathic—? autoimmune
Granulomatous disease—sarcoid, histiocytosis X
Hereditary—autosomal dominant mutations in preprovasopressin/neurophysin, Wolfram syndrome
Nephrogenic diabetes insipidus
Genetic
X-linked—V2 vasopressin receptor
Autosomal recessive/dominant—aquaporin-2
Autosomal recessive—aquaporin-1 (proximal tubule and thin limb)
Drug-induced, e.g., lithium, cisplatin, democlocycline, ifosfamide, foscarnet
Hypokalemia
Hypercalcemia
Infiltrating lesions, e.g., sarcoidosis, amyloidosis
Cellular defect, e.g., after acute tubular necrosis
Gestational diabetes insipidus
Pre-existing DI
Transient DI during pregnancy
DI after delivery
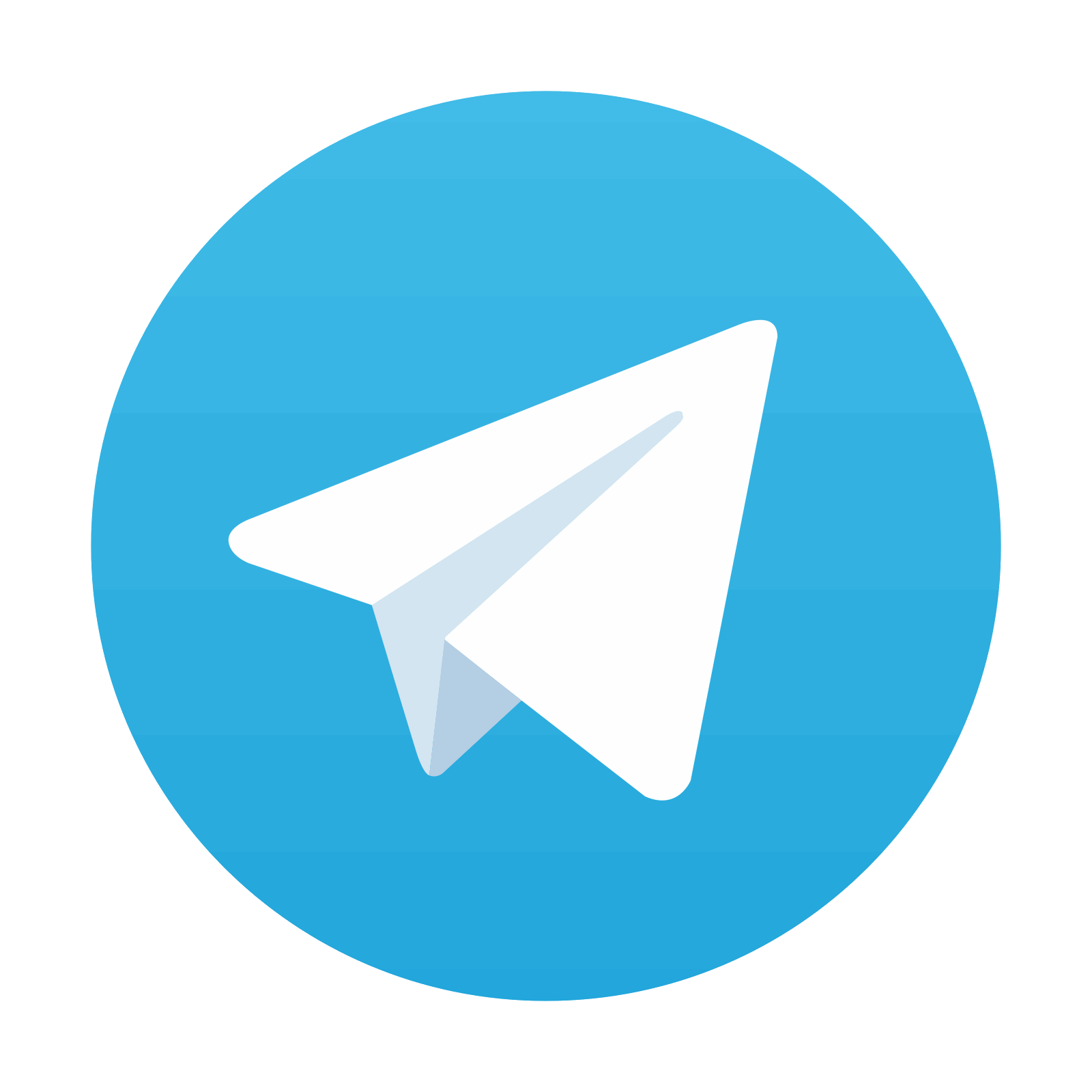
Stay updated, free articles. Join our Telegram channel

Full access? Get Clinical Tree
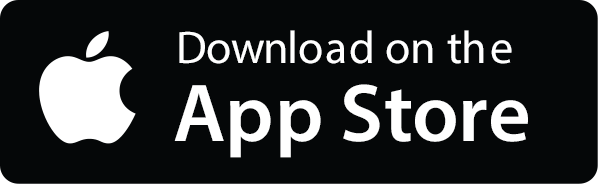
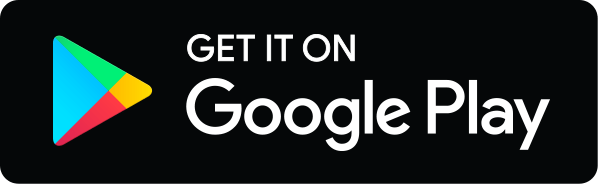