Fig. 4.1
Neurovascular dysregulation and neuroprotection mechanisms of LBP. Under the challenge of external insults, e.g., oxidative stress, aging, and ischemia, LBP inhibits the dysregulation of microenvironment and improves the survival of neurons. LBP polysaccharide of Lycium barbarum, ET-1 endothelin-1, Aβ amyloid-β
4.2 Maintaining the Integrality of Blood Vessels
The neurovascular unit consists of the endothelial cells, pericytes, astrocytes, microglia, and neurons, seen as Fig. 4.2. One function of neurovascular unit is to maintain the structural integrity of the blood–brain barriers (BBB) (Desai et al. 2007).


Fig. 4.2
The structure of neurovascular unit. In the central is the blood vessel. Around the blood vessel, there are neuronal axons, astrocytic endfeets and microglia, which cross-talk with blood vessel endothelial cells and pericytes. EC endothelial cell, TJ tight junction
The BBB is critical in regulating the normal cerebral extracellular environment. Microvascular endothelium forms the first layer of BBB. The disruption of BBB resulted in the leakage of serum inflammatory protein from the circulation, which could destroy the endothelium and endothelial TJ (Farrall and Wardlaw 2009). Increased permeability of BBB can cause the intravascular fluid flowing into the brain parenchyma, resulting in perivascular edema. Edema is harmful to the supply of blood flow, contributing to the irreversible damage of brain (Kimelberg 1995). This forms a vicious cycle, which accelerates the degeneration of neurons.
The blood–retinal barrier (BRB) is an extension of the BBB. LBP could maintain the integrality of BBB and BRB, which showed in our previous studies (Mi et al. 2012b; Li et al. 2011). The effects of LBP on the protection of BBB include reducing Evans Blue (EB) extravasate, decreasing IgG leakage, upregulating the expression of occludin, and recover the integrity of BBB (Yang et al. 2012).
The BRB maintains physiological and structural balance within the retina, which contains inner and outer barriers. TJ between adjacent endothelial cells forms the inner BRB, while the outer barrier is formed by TJ between the retinal pigment epithelial (RPE) cells (Kim et al. 2013). As the extension of BBB, the permeability is a damage marker. To represent permeability, IgG leakage can be used to detect the damage of BRB (Cheung et al. 2005a). Our previous studies detected the protection of LBP on the BRB by decreasing IgG leakage (Fig. 4.3) (Mi et al. 2012b). A recent study indicated that LBP could increase the level of antiapoptotic protein Bcl-2 in epithelial cell (Song et al. 2012). RPE cells are particularly susceptible to oxidative stress (Beatty et al. 2000). Enhanced antiapoptotic protein Bcl-2 can act against oxidative stress and apoptosis (Ren et al. 2009). Thus, LBP could protect the BRB from disruption, not only for the inner but also for the outer barriers.


Fig. 4.3
Decreased leakage in LBP-fed-AOH retina at day 4 after AOH. This picture showed IgG leakages around blood vessels in AOH retina, while almost disappeared when pre-treated with LBP. Scale bar: 10 μm
4.3 Protection of Endothelial Cells and Pericytes
Endothelial cell and pericytes are main cell contents of blood vessel barriers. Pericytes are mural cells inside the blood vessel wall which are known to play key roles in regulating vascular formation, stability, function, and controlling endothelial proliferation (Hammes 2005). Under the challenge of stress from external environment, e.g., ischemia, the loss or proliferation of endothelial cells and pericytes will impact on the vascular remodeling.
Neovascularization is a kind of negative vascular remodeling, which will accrue under the condition of the loss or proliferation of endothelial cells and pericytes. Neovascularization is also a kind of blood vessel response for compensation of ischemia in tissue. However, it will become a vicious circle so that ischemia could induce the loss of endothelial cells and pericytes. In response, endothelial cells and pericytes will proliferate and aggravate the ischemia in tissue.
Thus, one finding of our previous study showed that LBP could prevent the loss of endothelial cells and pericytes at the early stage of ischemia (since in the experimental design, we fed the animal the LBP solution in advance of ischemia challenge), which played a role to prevent the disadvantaged change of vascular remodeling (Mi et al. 2012b).
4.4 Regulating the Response of Blood Vessel-Associated Astrocytes
4.4.1 Astrocytes
Astrocytes are divided into protoplasmic and fibrous types based on their morphologic characters and the distribution (Blackwood 1976; Hirano 1985; Privat and Rataboul 1987). The morphology of astrocytes are complex, different morphology of astrocytes can coexist in a given brain region (Matyash and Kettenmann 2010). Astrocytes can also express different functions in gap junction coupling, expression of transmitter receptors, membrane currents, and glutamate transporters (Matyash and Kettenmann 2010). The distribution of astrocytes is strongly determined by vasculature (Stone and Dreher 1987). Astrocytes which are closed to retinal vessels (Schnitzer 1987) will help maintain vascular integrity (Zhang and Stone 1997). Astrocytes enlarge their end-feet around blood vessels, participating in the formation of TJ of endothelial cells which could prevent BBB from damage, and also modulate the transport function of the cerebral endothelium (Montgomery 1994). Astrocytes are known to be the main target of ET-1 (Sasaki et al. 1997; Prasanna et al. 2003). Previous studies found that astrocytic ET-1 has deleterious effects on water homeostasis, cerebral edema, and BBB integrity, which contribute to severe ischemic brain injury (Lo et al. 2005). Disruption of BRB leads to the swelling of astrocytes associated with the overexpression of glial fibrillary acidic protein (GFAP) and aquaporin-4 (AQP4) under the ischemic insult (Kaur et al. 2007). Each astrocyte could form end-feet around at least one blood vessel, while blood vessels can affect the morphology of astrocytes (Bushong et al. 2002). Our previous study showed that LBP can decrease the number of proliferated astrocytes and reduce their end-foot edema around the blood vessel, which could protect the survival of neurons. Thus, LBP can induce the blood-vessel-associated astrocytes to show entirely different morphologic change. These changes included apparently more processes touching blood vessels without enlargement of their end-feet (Leung et al. 2009b; Lo et al. 2005; Yeung et al. 2009).
4.4.2 Reducing the Expression of GFAP
In normal retina, GFAP is expressed on astrocytes and the end-feet of Müller cells. When under the stress, the expression of GFAP will increase not only on astrocytes but also on the process of the Müller cells (Cheung et al. 2005b; Mori et al. 2009; Taylor et al. 2010; Hirrlinger et al. 2010). It was reported that the overexpression of GFAP could be as the indicator for the reduction of blood flow (Osborne et al. 1991). From our previous studies, LBP could reduce the expression of GFAP, which may be a possible mechanism for recovery of blood supply to the tissue (Mi et al. 2012b).
4.4.3 Decreasing the Expression of AQP4
AQP4 is a member of water channel protein family which mainly expressed in astrocytes (Asai et al. 2013) and also a marker to reflect the capability to maintain the balance of water across the wall of blood vessels and to detect the functional change of astrocytes (Nagelhus et al. 1998). AQP4 is linked to the damage of BBB, suggesting the increase of permeability (Guo et al. 2006). It was reported that overexpression of AQP4 was related to the retinal swelling and involved in the process of neuronal death (Verkman et al. 2008). Previous studies demonstrated that pretreatment with LBP could reduce the expression of AQP4 in the animal model of ischemia in retina and brain (Li et al. 2011; Mi et al. 2012b; Yang et al. 2012).
4.5 Regulating Blood-Vessel-Related Factors and the Blood Flow
4.5.1 ET-1
ET-1, synthesized in vascular endothelial cells, is a potent vasoconstrictor. ET-1 regulates blood flow mainly via its two receptors, ETA and ETB (Narayan et al. 2003). ETA is expressed on vascular smooth muscle cells and contracts blood vessels, while ETB is mainly expressed in the endothelium and astrocytes and dilates blood vessels (Narayan et al. 2003; Masaki et al. 1994). Overexpression of ET-1 results in downregulating the level of occludin, the key protein located at TJ between blood vessel endothelial cells to maintain the integrity of blood-barrier (Leung et al. 2009a). Decreased expression of occludin has been reported in middle cerebral artery occlusion (MCAO)-induced ischemic brain and retina. However, LBP-pretreatment could maintain the level of occludin among blood vessels (Yang et al. 2012; Mi et al. 2012b). Further studies showed that LBP could reduce the ET-1-ETA signal pathway while activate ET-1-ETB pathway in the retinal blood vessels through downregulating the expression of ETA and upregulating ETB (Yorio et al. 2002). Not only endothelial cells, ET-1 is considered to stimulate contraction of pericytes (Ramachandran et al. 1993). Pericytes are considered to be other contractile cells to help regulating the blood flow (Wallow and Burnside 1980). Thus, through regulating the expression of ETA and ETB receptors, LBP could modulate the blood flow of retinal blood vessels (Mi et al. 2012a, b). Thus, LBP could protect endothelial cells and pericytes via downregulating ET-1.
4.5.2 Vascular Endothelial Growth Factor (VEGF) and Transforming Growth Factor-Beta (TGF-β)
Angiogenesis is the generation of new blood vessels from the original ones, playing an important role in the pathogenesis of diabetes. VEGF and TGF-β are angiogenin mediators. The high level of glucose in blood could result in the overexpression of VEGF (Martin et al. 2003) and TGF-β (Singh et al. 2013), contributing to vascular abnormalities in diabetes. LBP was shown to have a significant effect on reducing VEGF (Tian et al. 2013) and TGF-β (Singh et al. 2013). One recent study reported that a wolfberry contented formula diet (including Rhizoma dioscorea, L. barbarum, Prunella vulgaris and hawthorn) had effects on antidiabetes (Cheng et al. 2014).
4.5.3 The Receptor for Advanced Glycation Endproducts (RAGE)
RAGE is expressed on both smooth muscle cells and endothelial cells of blood vessel (Farmer and Kennedy 2009). It was reported that one role of RAGE was to transport Aβ across BBB to the brain tissue. Thereafter, Aβ could interact with RAGE resulting in the release of ET-1 from endothelial cells (Deane et al. 2003a), and contributing to vascular inflammation and endothelial dysfunction (Harja et al. 2008). Our previous study has proved that LBP can reduce the expression of RAGE (Mi et al. 2012b), therefore to inhibit the destructive effect of RAGE to blood vessels under ischemic insults.
4.5.4 Aβ
Aβ is a ligand of RAGE, which can induce vasoconstriction, decrease cerebral flow, and enhance the effect of ET-1-induced vasoconstriction (Paris et al. 2000). The accumulation of Aβ transported from BBB is also toxic to vasculature (Zlokovic 2005). ET-1 could inhibit the cerebral blood flow (CBF) through activating Aβ-RAGE transportation across the BBB (Deane et al. 2003b). LBP could downregulate the expression of Aβ (Mi et al. 2012b) thus to inhibit the Aβ-induced vascular toxic effect (Ho et al. 2010).
4.5.5 Advanced Glycation Endproducts (AGEs)
AGE is another key ligand of RAGE. The accumulation of AGE will activate RAGE, leading to endothelial dysfunction (Xu et al. 2003). Besides, AGE-RAGE pathway could enhance the synthesis of ET-1 in the endothelial cells (Quehenberger et al. 2000). Amount of evidences showed that AGE could induce the diabetic vascular complication (Takeuchi et al. 2000; Yamagishi et al. 2002b). AGE could induce pericyte apoptosis and VEGF expression contributing to the destruction of retinal vasculature (Yamagishi et al. 2002a). Thus, accumulation of AGE and overexpression of VEGF will aggravate the disorder of the vascular system (Yokoi et al. 2005). In our previous study, it was demonstrated that LBP could downregulate the expression of AGE, which could inhibit the destructive effect of AGE to blood vessels (Mi et al. 2012b).
4.6 Blood Flow and Hemodynamics
In normal microenvironment, the neurovascular unit regulates the blood flow by the end-feet activity of astrocytes (Iadecola and Nedergaard 2007), the autoregulation of blood vessels (Flammer and Mozaffarieh 2007) and vascular activating factors, such as ET-1 (Geijssen and Greve 1995). LBP was reported to dilate blood vessels and enhance the blood flow to the kidney in hypertensive rats (Jia et al. 1998). The mechanism is related to regulating the endothelin system.
The blood supply to the eye is referred to the difference between blood pressure and ocular pressure. Nocturnal systemic hypotension increases the risk of glaucoma progression (Charlson et al. 2014). In ocular hypertension, the experimental evidence showed that the capacity of blood flow autoregulation is vulnerable to the low blood pressure. This hemodynamic mechanism plays an important role in the development of glaucoma (Wang et al. 2014). Since the blood flow to optic nerve head is more easily observed to the decrease of the blood pressure (Wang et al. 2014). Although there was no direct evidence to show L. barbarum could increase ocular blood flow (OBF) to optic nerve head, through regulating endothelin and its receptors ETA and ETB, L. barbarum may be beneficial to the blood supply of retinal neurons.
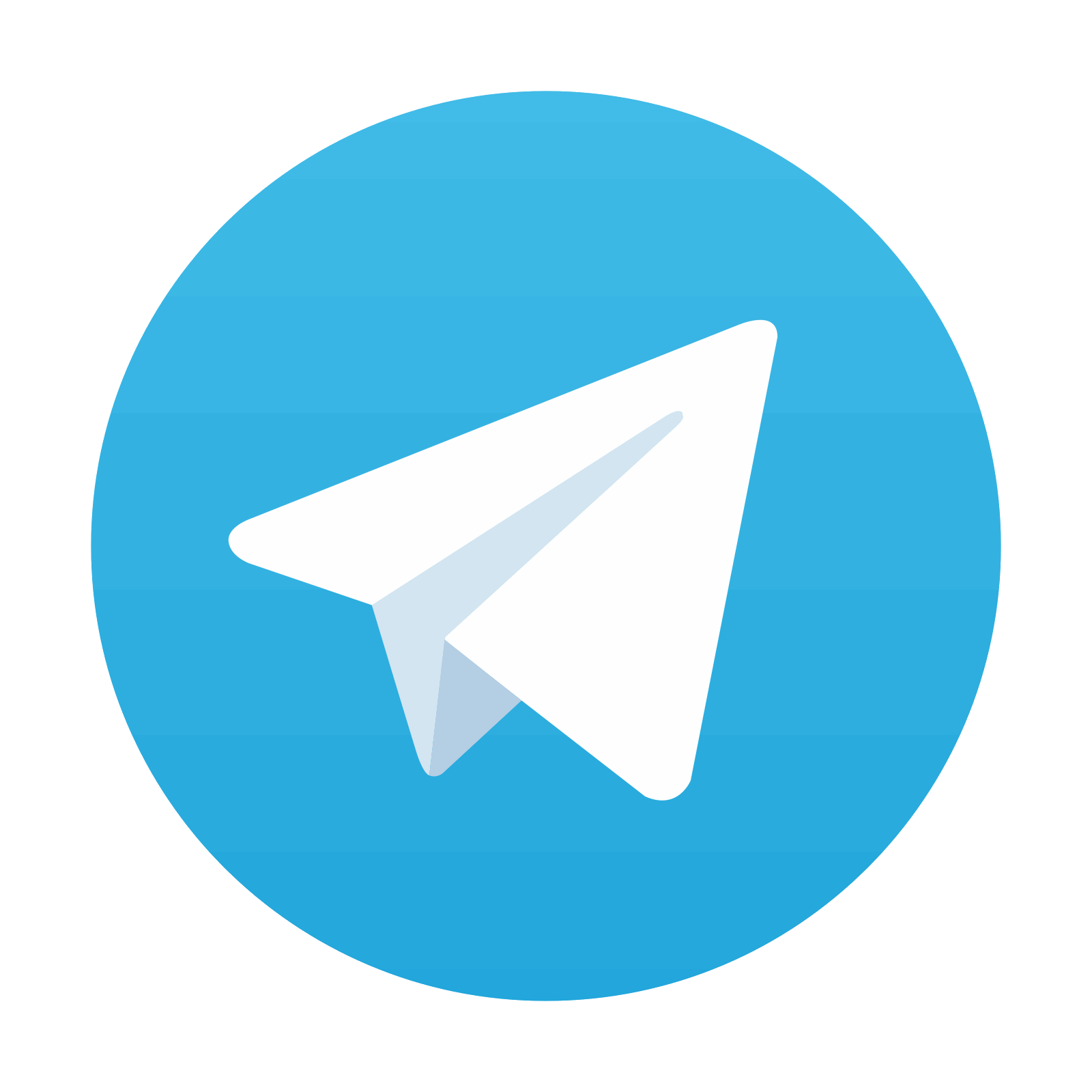
Stay updated, free articles. Join our Telegram channel

Full access? Get Clinical Tree
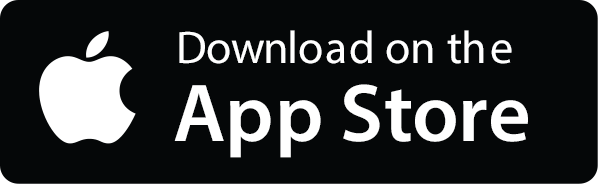
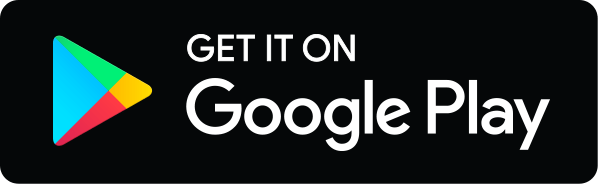