Drug Delivery to the Central Nervous System |
CONTENTS
15.1.1 Anatomy and Physiology of the BBB: Implications for CNS Drug Delivery
15.1.2 Transport Mechanisms across the BBB
15.1.2.2 Carrier-Mediated Transport
15.1.3 Overview of Approaches to CNS Delivery
15.2 Exploiting Natural Transport Mechanisms
15.2.3 Low-Density Lipoprotein Receptor-Related Protein 1
15.2.4 Adsorptive-Mediated Transcytosis
15.2.4.1 Cell-Penetrating Peptides
15.3 Nanotechnologies for CNS Delivery
15.3.3 Dendrimers for CNS Delivery
15.4 Invasive Approaches to CNS Delivery
15.4.1 Intracerebroventricular Drug Infusion
15.4.3 Convection-Enhanced Delivery
15.5 Intranasal Administration for Brain Delivery
Treatments for disorders of the central nervous system (CNS) are currently limited because of the difficulty in successfully delivering CNS therapeutics to the brain. The CNS is protected by a barrier known as the blood–brain barrier (BBB), which regulates brain homeostasis. The BBB restricts access of blood-borne compounds to brain cells, only allowing the entry of molecules that are essential for adequate brain function. This comprehensive gatekeeper action not only ensures the maintenance of a precisely regulated microenvironment for reliable neuronal signaling, but also presents a substantial barrier to the access of therapeutic drug molecules to brain tissue.
Successful delivery of therapeutics to the CNS would be beneficial in the treatment of a wide range of conditions (Table 15.1). Furthermore, an ageing population has meant the incidence of CNS diseases of “old age,” such as dementia, Alzheimer’s disease, stroke, and Parkinson’s disease, has increased significantly.
TABLE 15.1
Disorders of the Central Nervous System
CNS Condition | Example |
Vascular disorders | Stroke and transient ischemic attack |
Infections | Meningitis and encephalitis |
Brain cancer | Primary and metastatic |
Functional disorders | Epilepsy and neuralgia |
Degenerative disorders | Alzheimer’s disease Parkinson’s disease Amyotrophic lateral sclerosis Multiple sclerosis |
Psychiatric disorders | Depression, mania, anxiety |
Although considerable research effort has been directed toward developing new CNS drugs for these conditions, the associated delivery problems has meant that promising new drug candidates have failed to realize their therapeutic potential. Successful CNS drug delivery and targeting strategies could therefore considerably expand the treatment possibilities currently available for a wide range of CNS pathologies. Improved drug delivery to the brain would also improve the therapeutic profile of existing CNS drugs, allowing them to be administered at lower doses, thereby reducing adverse and toxic effects.
This chapter begins with a description of the anatomical and physiological constraints afforded by the BBB and the implications therein for the successful delivery of CNS therapeutics. This is followed by a discussion of the most promising strategies currently under investigation to achieve effective CNS transport.
15.1.1 ANATOMY AND PHYSIOLOGY OF THE BBB: IMPLICATIONS FOR CNS DRUG DELIVERY
Brain capillaries are lined with specialized endothelial cells, which serve to limit the entry of xenobiotics into the brain microenvironment. These endothelial cells constitute the major physical barrier of the BBB (Brightman 1977; Patel et al. 2012). Tight junction complexes between brain capillary endothelial cells form a high-resistance barrier, which inhibits paracellular transport (i.e., drug transport between the cells; Figure 15.1b). This is in marked contrast to endothelial capillaries of the peripheral circulation that lack tight junctions between the endothelial cells and thus allow the relatively free exchange of substances between cells and tissues (Figure 15.1b). Furthermore, brain endothelial cells possess no fenestrae (openings) for the passage of molecules, again in contrast to the peripheral endothelium. Phagocytic cells known as pericytes, located on the brain side of the endothelium, share a common basement membrane with the endothelial cells and further complement the physical barrier (Figure 15.1b). Further components of the neurovascular unit are star-shaped glial cells called astrocytes. Astrocytic foot processes approach the endothelial cells to a distance of about 20 nm and secrete chemicals that maintain the unique selective permeability characteristics of the capillary endothelial cells.
This anatomical barrier is compounded by several physiological mechanisms, which further limit the transport of molecules from the blood to the brain. Brain endothelial cells
• Demonstrate minimal pinocytotic transport
• Have a high level of intracellular metabolic enzyme activity, which can degrade an active pharmaceutical ingredient (API) as it moves through the endothelial cell in transit to the brain parenchyma
• Express high levels of active efflux transport proteins, including P-glycoprotein (P-gp), multidrug resistance–associated proteins (MRPs), and breast cancer resistance protein (BCRP, ABCG2). The pumps are expressed at the luminal membrane of the brain capillary, so that drugs that have gained entry into the cell can be expelled back into the circulation (Figure 15.1c).
15.1.2 TRANSPORT MECHANISMS ACROSS THE BBB
Transport routes and mechanisms across epithelial barriers are described extensively in Chapter 4 (Section 4.3) and are equally applicable here for endothelial transport. To summarize here, the paracellular route is not normally possible across brain capillaries, due to the presence of tight junctions between the cells that form a tight physical barrier, as described earlier. Transcellular transport (i.e., through the cells) is possible via a variety of mechanisms, considered in turn.
The majority of CNS therapeutics already on the market or in clinical trials comprise small (<400 Da), lipophilic molecules, which passively diffuse across the BBB down their concentration gradient. The rate of transcellular diffusion is governed by Fick’s first law (Chapter 4, Equation 4.1). Many drugs are too large, ionized, and hydrophilic to meet these physicochemical requirements and so cannot gain access to the CNS. Furthermore, many drugs that are able to enter the cell by transcellular passive are also substrates for efflux pumps. For example, many HIV-protease inhibitors and chemotherapeutics exhibit a high degree of lipophilicity but do not access the brain, which has been attributed to efflux pumps actively exporting them back into the systemic circulation after their initial entry. A further limitation to simple diffusional transport is that drugs that diffuse into endothelial cells may subsequently be vulnerable to the degradative enzymes localized within the cells.
15.1.2.2 Carrier-Mediated Transport
Certain small, hydrophilic molecules, vital for brain function, such as amino acids, glucose, and nucleosides, use specific transporters expressed on the luminal and basolateral sides of the endothelial cells for brain entry. This carrier-mediated transport (CMT) involves stereospecific transporter proteins that mediate solute transport in the order of milliseconds. Some of these carriers have been widely studied. For example, glucose, necessary for cellular energy, enters the brain via CMT on the BBB GLUT1 glucose transporter. Large, neutral amino acids, required for brain protein and neurotransmitter synthesis, enter the brain via the BBB L-type amino acid transporter 1 (BBB LAT1).
Receptor-mediated endocytosis (RME) is a highly selective type of endocytosis, by which the brain can specifically take up large, hydrophilic ligands, including transferrin (Tf), albumin, insulin, insulin growth factor, low-density lipoprotein, and ceruloplasmin (see also Chapter 4, Section 4.3.2.3). The receptors for RME are integral membrane proteins that bind specific substrates. Ligand binding triggers the membrane to invaginate, forming a clathrin-coated vesicle that contains the receptor–ligand complex. Fusion with an endosome, and then lysosomal degradation, may follow. Alternatively, in receptor-mediated transcytosis (RMT), the endocytic vesicles move across the cell and undergo exocytosis on the opposite side, discharging their cargo into the brain. In contrast to RMT, which is triggered by a specific interaction with receptors expressed on cerebral endothelial cells, adsorptive-mediated transcytosis (AMT) facilitates vesicular delivery across the BBB via a nonspecific mechanism of electrostatic attraction. Peptides and proteins with a basic isoelectric point (“cationic” proteins), bind initially to the luminal plasma membrane, mediated by electrostatic interactions with anionic sites on the membrane. Adsorption triggers endocytosis/transcytosis, again facilitated by the clathrin-mediated system.
15.1.3 OVERVIEW OF APPROACHES TO CNS DELIVERY
CNS drug delivery research is focused on a number of key areas, summarized here and discussed in detail in the following sections:
1. Physiological approaches: Exploiting natural transport mechanisms. Many of the physiological transport systems that the brain uses to take up nutrients and other essential molecules can be exploited for enhancing CNS drug delivery.
2. Pharmaceutical approaches: Using nanotechnologies, such as liposomes, nanoparticles (NPs), and dendrimers, as drug carriers for CNS delivery.
3. Surgical approaches: Drugs can be introduced directly into the brain via surgical techniques.
4. Nose-to-brain delivery: A direct transport route exists from the nose to the brain, via the olfactory nerve. Therefore, a drug given intranasally can gain access to the brain without having to surmount the BBB.
5. Disruption of the BBB: The integrity of the BBB can be transiently disrupted via osmotic, ultrasonic, and chemical means, thereby temporarily allowing drugs to breach the barrier.
15.2 EXPLOITING NATURAL TRANSPORT MECHANISMS
Physiological approaches exploit the natural transport mechanisms of brain endothelium for the uptake of nutrients and other essential molecules. These mechanisms have been described in Section 15.1.2 and include CMT and RMT pathways. A drug molecule that is structurally similar to an endogenous substrate can be taken up the corresponding transporter. For example, the drug L-dopa is an effective therapeutic in the treatment of Parkinson’s disease because it can enter the brain via CMT, using the BBB LAT1 system described earlier (Wade and Katzman 1975). Any drug molecule can, in principle at least, be chemically modified so that it resembles the structure of an endogenous substrate, for uptake via the appropriate carrier. However, there is obviously a limit to the degree of chemical modification that can be carried out on a drug before its therapeutic activity is comprised.
Rather than focusing on the drug per se, an alternative approach is to conjugate a drug with a natural substrate of a transporter system, so that the drug–substrate conjugate can be taken up via the transporter. Monoclonal antibodies (mAbs) can also be raised against a specific transporter receptor, to form a drug–mAb conjugate for subsequent uptake. This conjugation strategy has been developed extensively by Pardridge and coworkers (Pardridge 2002, 2003, 2006), who coined the eloquent term “molecular Trojan horse” to evoke how a drug may surreptitiously gain entry to the CNS, under the cover of a natural substrate/mAb. Similarly, a nontransportable peptide can be conjugated to a BBB transport vector to form a so-called “chimeric peptide,” capable of BBB transit (Coloma et al. 2000; Pardridge 1989).
CMT pathways, as described in Section 15.1.2.2, are used physiologically for the uptake of small, hydrophilic molecules (such as glucose and amino acids), so this pathway has quite limited capacity for the uptake of large drug molecules. In contrast, RMT pathways exist for the CNS uptake of much larger molecules, such as insulin, therefore exploiting RMT pathways has much wider applicability for CNS drug delivery. Research in this area has focused in particular on targeting three different RMT receptors (Chen and Lihong 2012), described here in turn.
The insulin receptor (IR) is a tetrameric structure comprising two a chains that project into the plasma compartment of the brain capillaries and contain the binding site for insulin, and two β chains that contain tyrosine kinase activity and project into the intracellular compartment. Insulin per se cannot be used as a delivery vector, as the resulting drug–insulin conjugate could cause hypoglycemia. Research has instead focused on using a mAb to the IR as a targeting vector. A mouse mAb has been developed, designated mAb 83-14, which binds to an epitope contained within amino acids 469–592 of the α chain of the IR. The ability of mAb 83-14 to undergo transcytosis has been demonstrated in rhesus monkeys. Following a single i.v. dose, 4% of the Ab was delivered to the brain, whereas no brain uptake was observed for controls (Pardridge et al. 1995). Genetically-engineered forms of the human IR mAb have been now developed, and research in this area is ongoing.
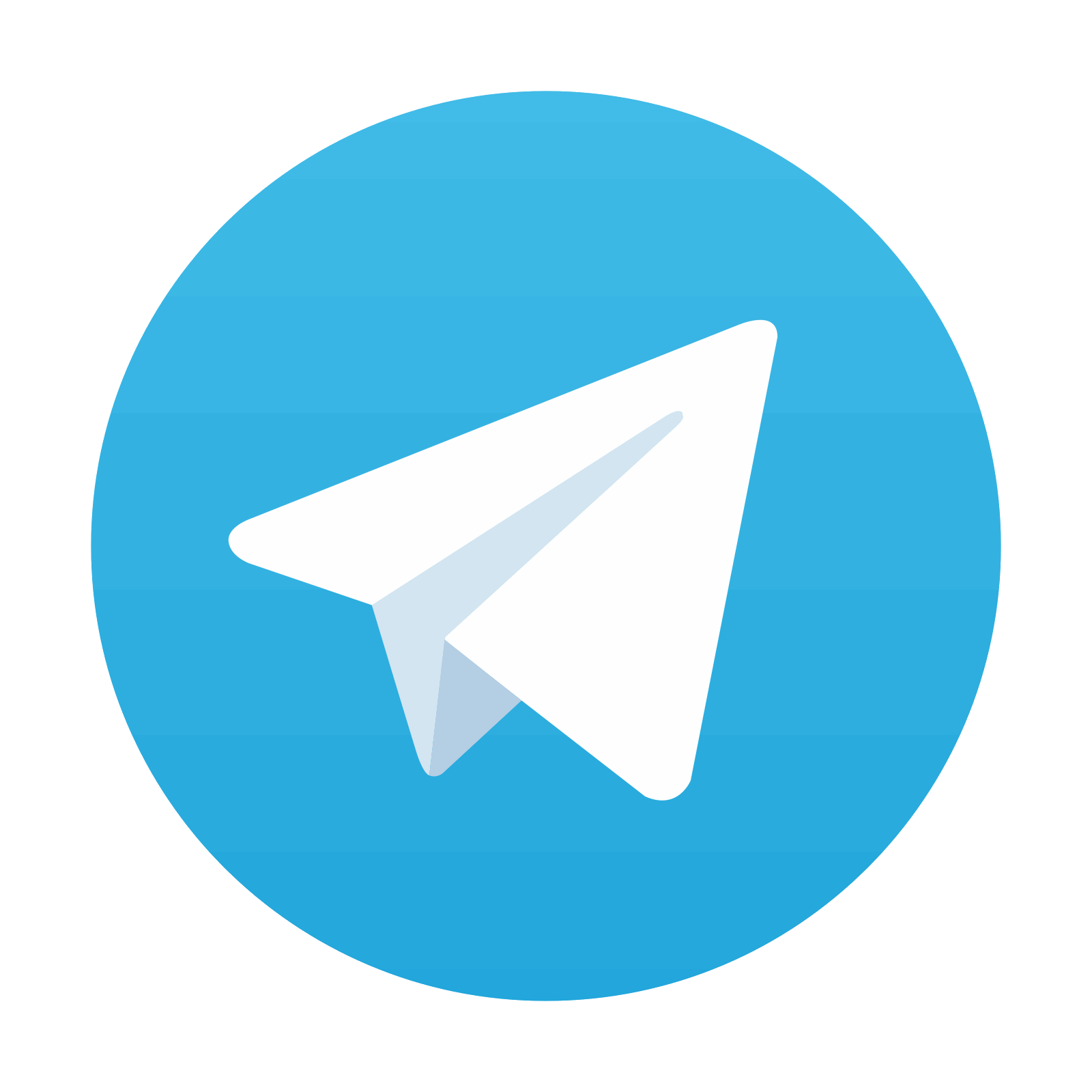
Stay updated, free articles. Join our Telegram channel

Full access? Get Clinical Tree
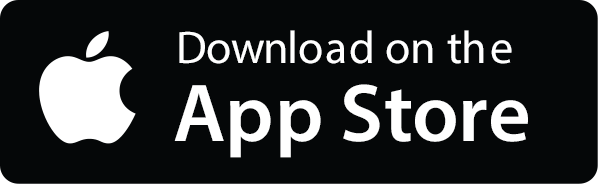
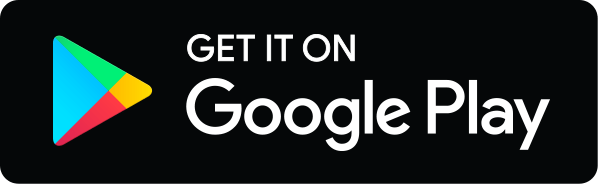