FIGURE 415e-3 Factors released by the adipocyte that can affect peripheral tissues. IL-6, interleukin 6; PAI, plasminogen activator inhibitor; RBP4, retinal binding protein 4; TNF, tumor necrosis factor.
ETIOLOGY OF OBESITY
Although the molecular pathways regulating energy balance are beginning to be illuminated, the causes of obesity remain elusive. In part, this reflects the fact that obesity is a heterogeneous group of disorders. At one level, the pathophysiology of obesity seems simple: a chronic excess of nutrient intake relative to the level of energy expenditure. However, due to the complexity of the neuroendocrine and metabolic systems that regulate energy intake, storage, and expenditure, it has been difficult to quantitate all the relevant parameters (e.g., food intake and energy expenditure) over time in human subjects.
Role of Genes Versus Environment Obesity is commonly seen in families, and the heritability of body weight is similar to that for height. Inheritance is usually not Mendelian, however, and it is difficult to distinguish the role of genes and environmental factors. Adoptees more closely resemble their biologic than adoptive parents with respect to obesity, providing strong support for genetic influences. Likewise, identical twins have very similar BMIs whether reared together or apart, and their BMIs are much more strongly correlated than those of dizygotic twins. These genetic effects appear to relate to both energy intake and expenditure. Currently, identified genetic variants, both common and rare, account for less than 5% of the variance of body weight.
Whatever the role of genes, it is clear that the environment plays a key role in obesity, as evidenced by the fact that famine prevents obesity in even the most obesity-prone individual. In addition, the recent increase in the prevalence of obesity in the United States is far too rapid to be due to changes in the gene pool. Undoubtedly, genes influence the susceptibility to obesity in response to specific diets and availability of nutrition. Cultural factors are also important—these relate to both availability and composition of the diet and to changes in the level of physical activity. In industrial societies, obesity is more common among poor women, whereas in underdeveloped countries, wealthier women are more often obese. In children, obesity correlates to some degree with time spent watching television. Although the role of diet composition in obesity continues to generate controversy, it appears that high-fat diets may, when combined with simple, rapidly absorbed carbohydrates, promote obesity. Specific genes are likely to influence the response to specific diets, but these genes are largely unidentified.
Additional environmental factors may contribute to the increasing obesity prevalence. Both epidemiologic correlations and experimental data suggest that sleep deprivation leads to increased obesity. Changes in gut microbiome with capacity to alter energy balance are receiving experimental support from animal studies, and a possible role for obesigenic viral infections continues to receive sporadic attention.
Specific Genetic Syndromes For many years, obesity in rodents has been known to be caused by a number of distinct mutations distributed through the genome. Most of these single-gene mutations cause both hyperphagia and diminished energy expenditure, suggesting a physiologic link between these two parameters of energy homeostasis. Identification of the ob gene mutation in genetically obese (ob/ob) mice represented a major breakthrough in the field. The ob/ob mouse develops severe obesity, insulin resistance, and hyperphagia, as well as efficient metabolism (e.g., it gets fat even when ingesting the same number of calories as lean litter mates). The product of the ob gene is the peptide leptin, a name derived from the Greek root leptos, meaning thin. Leptin is secreted by adipose cells and acts primarily through the hypothalamus. Its level of production provides an index of adipose energy stores (Fig. 415e-4). High leptin levels decrease food intake and increase energy expenditure. Another mouse mutant, db/db, which is resistant to leptin, has a mutation in the leptin receptor and develops a similar syndrome. The ob gene is present in humans where it is also expressed in fat. Several families with morbid, early-onset obesity caused by inactivating mutations in either leptin or the leptin receptor have been described, thus demonstrating the biologic relevance of the leptin pathway in humans. Obesity in these individuals begins shortly after birth, is severe, and is accompanied by neuroendocrine abnormalities. The most prominent of these is hypogonadotropic hypogonadism, which is reversed by leptin replacement in the leptin-deficient subset. Central hypothyroidism and growth retardation are seen in the mouse model, but their occurrence in leptin-deficient humans is less clear. Mutations in the leptin or leptin receptor genes do not play a prominent role in common forms of obesity.
FIGURE 415e-4 The physiologic system regulated by leptin. Rising or falling leptin levels act through the hypothalamus to influence appetite, energy expenditure, and neuroendocrine function and through peripheral sites to influence systems such as the immune system.
Mutations in several other genes cause severe obesity in humans (Table 415e-1); each of these syndromes is rare. Mutations in the gene encoding proopiomelanocortin (POMC) cause severe obesity through failure to synthesize α-MSH, a key neuropeptide that inhibits appetite in the hypothalamus. The absence of POMC also causes secondary adrenal insufficiency due to absence of adrenocorticotropic hormone (ACTH), as well as pale skin and red hair due to absence of α-MSH. Proenzyme convertase 1 (PC-1) mutations are thought to cause obesity by preventing synthesis of α-MSH from its precursor peptide, POMC. α-MSH binds to the type 4 melanocortin receptor (MC4R), a key hypothalamic receptor that inhibits eating. Heterozygous loss-of-function mutations of this receptor account for as much as 5% of severe obesity. Loss of function of MRAP2, a protein required for normal MC4R signaling, has been found in rare cases of severe obesity. These six genetic defects define a pathway through which leptin (by stimulating POMC and increasing α-MSH) restricts food intake and limits weight (Fig. 415e-5). The results of genomewide association studies to identify genetic loci responsible for obesity in the general population have so far been disappointing. More than 40 replicated loci linked to obesity have been identified, but together they account for less than 3% of interindividual variation in BMI. The most replicated of these is a gene named FTO, which is of unknown function, but like many of the other recently described candidates, is expressed in the brain. Because the heritability of obesity is estimated to be 40–70%, it is likely that many more loci remain to be identified. It is possible that epistatic interactions between causative loci or unknown gene-environment interactions explain the poor success at identifying causal loci.
SELECTED OBESITY GENES IN HUMANS AND MICE |
FIGURE 415e-5 A central pathway through which leptin acts to regulate appetite and body weight. Leptin signals through proopiomelanocortin (POMC) neurons in the hypothalamus to induce increased production of α-melanocyte-stimulating hormone (α-MSH), requiring the processing enzyme PC-1 (proenzyme convertase 1). α-MSH acts as an agonist on melanocortin-4 receptors to inhibit appetite, and the neuropeptide AgRp (Agouti-related peptide) acts as an antagonist of this receptor. Mutations that cause obesity in humans are indicated by the solid green arrows.
In addition to these human obesity genes, studies in rodents reveal several other molecular candidates for hypothalamic mediators of human obesity or leanness. The tub gene encodes a hypothalamic peptide of unknown function; mutation of this gene causes late-onset obesity. The fat gene encodes carboxypeptidase E, a peptide-processing enzyme; mutation of this gene is thought to cause obesity by disrupting production of one or more neuropeptides. AgRP is coexpressed with NPY in arcuate nucleus neurons. AgRP antagonizes α-MSH action at MC4 receptors, and its overexpression induces obesity. In contrast, a mouse deficient in the peptide MCH, whose administration causes feeding, is lean.
A number of complex human syndromes with defined inheritance are associated with obesity (Table 415e-2). Although specific genes have limited definition at present, their identification will likely enhance our understanding of more common forms of human obesity. In the Prader-Willi syndrome, a multigenic neurodevelopmental disorder, obesity coexists with short stature, mental retardation, hypogonadotropic hypogonadism, hypotonia, small hands and feet, fish-shaped mouth, and hyperphagia. Most patients have reduced expression of imprinted paternally inherited genes encoded in the 15q11-13 chromosomal region. Reduced expression of Snord116, a small nucleolar RNA highly expressed in hypothalamus, may be an important cause of defective hypothalamic function in this disorder. Bardet-Biedl syndrome (BBS) is a genetically heterogeneous disorder characterized by obesity, mental retardation, retinitis pigmentosa, diabetes, renal and cardiac malformations, polydactyly, and hypogonadotropic hypogonadism. At least 12 genetic loci have been identified, and most of the encoded proteins form two multiprotein complexes that are involved in ciliary function and microtubule-based intracellular transport. Some evidence suggests that mutations might disrupt leptin receptor trafficking in key hypothalamic neurons, causing leptin resistance.
A COMPARISON OF SYNDROMES OF OBESITY—HYPOGONADISM AND MENTAL RETARDATION |
Other Specific Syndromes Associated with Obesity • CUSHING’S SYNDROME Although obese patients commonly have central obesity, hypertension, and glucose intolerance, they lack other specific stigmata of Cushing’s syndrome (Chap. 406). Nonetheless, a potential diagnosis of Cushing’s syndrome is often entertained. Cortisol production and urinary metabolites (17OH steroids) may be increased in simple obesity. Unlike in Cushing’s syndrome, however, cortisol levels in blood and urine in the basal state and in response to corticotropin-releasing hormone (CRH) or ACTH are normal; the overnight 1-mg dexamethasone suppression test is normal in 90%, with the remainder being normal on a standard 2-day low-dose dexamethasone suppression test. Obesity may be associated with excessive local reactivation of cortisol in fat by 11β-hydroxysteroid dehydrogenase 1, an enzyme that converts inactive cortisone to cortisol.
HYPOTHYROIDISM The possibility of hypothyroidism should be considered, but it is an uncommon cause of obesity; hypothyroidism is easily ruled out by measuring thyroid-stimulating hormone (TSH). Much of the weight gain that occurs in hypothyroidism is due to myxedema (Chap. 405).
INSULINOMA Patients with insulinoma often gain weight as a result of overeating to avoid hypoglycemic symptoms (Chap. 420). The increased substrate plus high insulin levels promote energy storage in fat. This can be marked in some individuals but is modest in most.
CRANIOPHARYNGIOMA AND OTHER DISORDERS INVOLVING THE HYPOTHALAMUS Whether through tumors, trauma, or inflammation, hypothalamic dysfunction of systems controlling satiety, hunger, and energy expenditure can cause varying degrees of obesity (Chap. 402). It is uncommon to identify a discrete anatomic basis for these disorders. Subtle hypothalamic dysfunction is probably a more common cause of obesity than can be documented using currently available imaging techniques. Growth hormone (GH), which exerts lipolytic activity, is diminished in obesity and is increased with weight loss. Despite low GH levels, insulin-like growth factor (IGF) I (somatomedin) production is normal, suggesting that GH suppression may be a compensatory response to increased nutritional supply.
Pathogenesis of Common Obesity Obesity can result from increased energy intake, decreased energy expenditure, or a combination of the two. Thus, identifying the etiology of obesity should involve measurements of both parameters. However, it is difficult to perform direct and accurate measurements of energy intake in free-living individuals; and the obese, in particular, often underreport intake. Measurements of chronic energy expenditure are possible using doubly labeled water or metabolic chamber/rooms. In subjects at stable weight and body composition, energy intake equals expenditure. Consequently, these techniques allow assessment of energy intake in free-living individuals. The level of energy expenditure differs in established obesity, during periods of weight gain or loss, and in the pre- or postobese state. Studies that fail to take note of this phenomenon are not easily interpreted.
There is continued interest in the concept of a body weight “set point.” This idea is supported by physiologic mechanisms centered around a sensing system in adipose tissue that reflects fat stores and a receptor, or “adipostat,” that is in the hypothalamic centers. When fat stores are depleted, the adipostat signal is low, and the hypothalamus responds by stimulating hunger and decreasing energy expenditure to conserve energy. Conversely, when fat stores are abundant, the signal is increased, and the hypothalamus responds by decreasing hunger and increasing energy expenditure. The recent discovery of the ob gene, and its product leptin, and the db gene, whose product is the leptin receptor, provides important elements of a molecular basis for this physiologic concept (see above).
What Is the Status of Food Intake in Obesity? (Do the Obese Eat More Than the Lean?) This question has stimulated much debate, due in part to the methodologic difficulties inherent in determining food intake. Many obese individuals believe that they eat small quantities of food, and this claim has often been supported by the results of food intake questionnaires. However, it is now established that average energy expenditure increases as individuals get more obese, due primarily to the fact that metabolically active lean tissue mass increases with obesity. Given the laws of thermodynamics, the obese person must therefore eat more than the average lean person to maintain their increased weight. It may be the case, however, that a subset of individuals who are predisposed to obesity have the capacity to become obese initially without an absolute increase in caloric consumption.
What Is the State of Energy Expenditure in Obesity? The average total daily energy expenditure is higher in obese than lean individuals when measured at stable weight. However, energy expenditure falls as weight is lost, due in part to loss of lean body mass and to decreased sympathetic nerve activity. When reduced to near-normal weight and maintained there for a while, (some) obese individuals have lower energy expenditure than (some) lean individuals. There is also a tendency for those who will develop obesity as infants or children to have lower resting energy expenditure rates than those who remain lean. The physiologic basis for variable rates of energy expenditure (at a given body weight and level of energy intake) is essentially unknown.
Another component of thermogenesis, called nonexercise activity thermogenesis (NEAT), has been linked to obesity. It is the thermogenesis that accompanies physical activities other than volitional exercise such as the activities of daily living, fidgeting, spontaneous muscle contraction, and maintaining posture. NEAT accounts for about two-thirds of the increased daily energy expenditure induced by overfeeding. The wide variation in fat storage seen in overfed individuals is predicted by the degree to which NEAT is induced. The molecular basis for NEAT and its regulation is unknown.
Leptin in Typical Obesity The vast majority of obese persons have increased leptin levels but do not have mutations of either leptin or its receptor. They appear, therefore, to have a form of functional “leptin resistance.” Data suggesting that some individuals produce less leptin per unit fat mass than others or have a form of relative leptin deficiency that predisposes to obesity are at present contradictory and unsettled. The mechanism for leptin resistance, and whether it can be overcome by raising leptin levels or combining leptin with other treatments in a subset of obese individuals, is not yet established. Some data suggest that leptin may not effectively cross the blood-brain barrier as levels rise. It is also apparent from animal studies that leptin-signaling inhibitors, such as SOCS3 and PTP1b, are involved in the leptin-resistant state.
PATHOLOGIC CONSEQUENCES OF OBESITY
(See also Chap. 416) Obesity has major adverse effects on health. Obesity is associated with an increase in mortality, with a 50–100% increased risk of death from all causes compared to normal-weight individuals, mostly due to cardiovascular causes. Obesity and overweight together are the second leading cause of preventable death in the United States, accounting for 300,000 deaths per year. Mortality rates rise as obesity increases, particularly when obesity is associated with increased intraabdominal fat (see above). Life expectancy of a moderately obese individual could be shortened by 2–5 years, and a 20- to 30-year-old male with a BMI >45 may lose 13 years of life. It is likely that the degree to which obesity affects particular organ systems is influenced by susceptibility genes that vary in the population.
Insulin Resistance and Type 2 Diabetes Mellitus Hyperinsulinemia and insulin resistance are pervasive features of obesity, increasing with weight gain and diminishing with weight loss (Chap. 422). Insulin resistance is more strongly linked to intraabdominal fat than to fat in other depots. Molecular links between obesity and insulin resistance in fat, muscle, and liver have been sought for many years. Major factors include: (1) insulin itself, by inducing receptor downregulation; (2) free fatty acids that are increased and capable of impairing insulin action; (3) intracellular lipid accumulation; and (4) several circulating peptides produced by adipocytes, including the cytokines TNF-α and IL-6, RBP4, and the “adipokines” adiponectin and resistin, which have altered expression in obese adipocytes and can modify insulin action. Additional mechanisms are obesity-linked inflammation, including infiltration of macrophages into tissues including fat, and induction of the endoplasmic reticulum stress response, which can bring about resistance to insulin action in cells. Despite the prevalence of insulin resistance, most obese individuals do not develop diabetes, suggesting that diabetes requires an interaction between obesity-induced insulin resistance and other factors such as impaired insulin secretion (Chap. 417). Obesity, however, is a major risk factor for diabetes, and as many as 80% of patients with type 2 diabetes mellitus are obese. Weight loss and exercise, even of modest degree, increase insulin sensitivity and often improve glucose control in diabetes.
Reproductive Disorders Disorders that affect the reproductive axis are associated with obesity in both men and women. Male hypogonadism is associated with increased adipose tissue, often distributed in a pattern more typical of females. In men whose weight is >160% ideal body weight (IBW), plasma testosterone and sex hormone–binding globulin (SHBG) are often reduced, and estrogen levels (derived from conversion of adrenal androgens in adipose tissue) are increased (Chap. 411). Gynecomastia may be seen. However, masculinization, libido, potency, and spermatogenesis are preserved in most of these individuals. Free testosterone may be decreased in morbidly obese men whose weight is >200% IBW.
Obesity has long been associated with menstrual abnormalities in women, particularly in women with upper body obesity (Chap. 412). Common findings are increased androgen production, decreased SHBG, and increased peripheral conversion of androgen to estrogen. Most obese women with oligomenorrhea have polycystic ovarian syndrome (PCOS), with its associated anovulation and ovarian hyperandrogenism; 40% of women with PCOS are obese. Most nonobese women with PCOS are also insulin-resistant, suggesting that insulin resistance, hyperinsulinemia, or the combination of the two are causative or contribute to the ovarian pathophysiology in PCOS in both obese and lean individuals. Increasing evidence supports a role for adipokines in mediating a link between obesity and the reproductive dysfunction of PCOS. In obese women with PCOS, weight loss or treatment with insulin-sensitizing drugs often restores normal menses. The increased conversion of androstenedione to estrogen, which occurs to a greater degree in women with lower body obesity, may contribute to the increased incidence of uterine cancer in postmenopausal women with obesity.
Cardiovascular Disease The Framingham Study revealed that obesity was an independent risk factor for the 26-year incidence of cardiovascular disease in men and women (including coronary disease, stroke, and congestive heart failure). The waist-to-hip ratio may be the best predictor of these risks. When the additional effects of hypertension and glucose intolerance associated with obesity are included, the adverse impact of obesity is even more evident. The effect of obesity on cardiovascular mortality in women may be seen at BMIs as low as 25. Obesity, especially abdominal obesity, is associated with an atherogenic lipid profile; with increased low-density lipoprotein cholesterol, very-low-density lipoprotein, and triglyceride; and with decreased high-density lipoprotein cholesterol and decreased levels of the vascular protective adipokine adiponectin (Chap. 421). Obesity is also associated with hypertension. Measurement of blood pressure in the obese requires use of a larger cuff size to avoid artifactual increases. Obesity-induced hypertension is associated with increased peripheral resistance and cardiac output, increased sympathetic nervous system tone, increased salt sensitivity, and insulin-mediated salt retention; it is often responsive to modest weight loss.
Pulmonary Disease Obesity may be associated with a number of pulmonary abnormalities. These include reduced chest wall compliance, increased work of breathing, increased minute ventilation due to increased metabolic rate, and decreased functional residual capacity and expiratory reserve volume. Severe obesity may be associated with obstructive sleep apnea and the “obesity hypoventilation syndrome” with attenuated hypoxic and hypercapnic ventilatory responses. Sleep apnea can be obstructive (most common), central, or mixed and is associated with hypertension. Weight loss (10–20 kg) can bring substantial improvement, as can major weight loss following gastric bypass or restrictive surgery. Continuous positive airway pressure has been used with some success.
Hepatobiliary Disease Obesity is frequently associated with nonalcoholic fatty liver disease (NAFLD), and this association represents one of the most common causes of liver disease in industrialized countries. The hepatic fatty infiltration of NAFLD progresses in a subset to inflammatory nonalcoholic steatohepatitis (NASH) and more rarely to cirrhosis and hepatocellular carcinoma. Steatosis typically improves following weight loss, secondary to diet or bariatric surgery. The mechanism for the association remains unclear. Obesity is associated with enhanced biliary secretion of cholesterol, supersaturation of bile, and a higher incidence of gallstones, particularly cholesterol gallstones (Chap. 369). A person 50% above IBW has about a sixfold increased incidence of symptomatic gallstones. Paradoxically, fasting increases supersaturation of bile by decreasing the phospholipid component. Fasting-induced cholecystitis is a complication of extreme diets.
Cancer Obesity is associated with increased risk of several cancer types, and in addition can lead to poorer treatment outcomes and increased cancer mortality. Obesity in males is associated with higher mortality from cancer of the esophagus, colon, rectum, pancreas, liver, and prostate; obesity in females is associated with higher mortality from cancer of the gallbladder, bile ducts, breasts, endometrium, cervix, and ovaries. Some of the latter may be due to increased rates of conversion of androstenedione to estrone in adipose tissue of obese individuals. Other possible mechanistic links may involve hormones, growth factors, and cytokines whose levels are linked to nutritional state, including insulin, leptin, adiponectin, and IGF-I, as well as activation of signaling pathways linked to both obesity and cancer. It has been estimated that obesity accounts for 14% of cancer deaths in men and 20% in women in the United States.
Bone, Joint, and Cutaneous Disease Obesity is associated with an increased risk of osteoarthritis, no doubt partly due to the trauma of added weight bearing, but potentially linked as well to activation of inflammatory pathways that could promote synovial pathology. The prevalence of gout may also be increased (Chap. 395). One of the skin problems associated with obesity is acanthosis nigricans, manifested by darkening and thickening of the skinfolds on the neck, elbows, and dorsal interphalangeal spaces. Acanthosis reflects the severity of underlying insulin resistance and diminishes with weight loss. Friability of skin may be increased, especially in skinfolds, enhancing the risk of fungal and yeast infections. Finally, venous stasis is increased in the obese.
416 | Evaluation and Management of Obesity |
More than 66% of U.S. adults are categorized as overweight or obese, and the prevalence of obesity is increasing rapidly in most of the industrialized world. Children and adolescents also are becoming more obese, indicating that the current trends will accelerate over time. Obesity is associated with an increased risk of multiple health problems, including hypertension, type 2 diabetes, dyslipidemia, obstructive sleep apnea, nonalcoholic fatty liver disease, degenerative joint disease, and some malignancies. Thus, it is important for physicians to identify, evaluate, and treat patients for obesity and associated comorbid conditions.
EVALUATION
Physicians should screen all adult patients for obesity and offer intensive counseling and behavioral interventions to promote sustained weight loss. The five main steps in the evaluation of obesity, as described below, are (1) a focused obesity-related history, (2) a physical examination to determine the degree and type of obesity, (3) assessment of comorbid conditions, (4) determination of fitness level, and (5) assessment of the patient’s readiness to adopt lifestyle changes.
The Obesity-Focused History Information from the history should address the following seven questions:
• What factors contribute to the patient’s obesity?
• How is the obesity affecting the patient’s health?
• What is the patient’s level of risk from obesity?
• What does the patient find difficult about managing weight?
• What are the patient’s goals and expectations?
• Is the patient motivated to begin a weight management program?
• What kind of help does the patient need?
Although the vast majority of cases of obesity can be attributed to behavioral factors that affect diet and physical activity patterns, the history may suggest secondary causes that merit further evaluation. Disorders to consider include polycystic ovarian syndrome, hypothyroidism, Cushing’s syndrome, and hypothalamic disease. Drug-induced weight gain also should be considered. Common causes include medications for diabetes (insulin, sulfonylureas, thiazolidinediones); steroid hormones; psychotropic agents; mood stabilizers (lithium); antidepressants (tricyclics, monoamine oxidase inhibitors, paroxetine, mirtazapine); and antiepileptic drugs (valproate, gabapentin, carbamazepine). Other medications, such as nonsteroidal anti-inflammatory drugs and calcium channel blockers, may cause peripheral edema but do not increase body fat.
The patient’s current diet and physical activity patterns may reveal factors that contribute to the development of obesity and may identify behaviors to target for treatment. This type of historic information is best obtained by the combination of a questionnaire and an interview.
Body Mass Index (BMI) and Waist Circumference Three key anthropometric measurements are important in evaluating the degree of obesity: weight, height, and waist circumference. The BMI, calculated as weight (kg)/height (m)2 or as weight (lbs)/height (inches)2 × 703, is used to classify weight status and risk of disease (Tables 416-1 and 416-2). BMI provides an estimate of body fat and is related to disease risk. Lower BMI thresholds for overweight and obesity have been proposed for the Asia-Pacific region since this population appears to be at risk for glucose and lipid abnormalities at lower body weights.
BODY MASS INDEX (BMI) |
CLASSIFICATION OF WEIGHT STATUS AND DISEASE RISK |
Excess abdominal fat, assessed by measurement of waist circumference or waist-to-hip ratio, is independently associated with a higher risk for diabetes mellitus and cardiovascular disease. Measurement of the waist circumference is a surrogate for visceral adipose tissue and should be performed in the horizontal plane above the iliac crest (Table 416-3).
ETHNIC-SPECIFIC CUTPOINT VALUES FOR WAIST CIRCUMFERENCE |
Physical Fitness Several prospective studies have demonstrated that physical fitness, reported by questionnaire or measured by a maximal treadmill exercise test, is an important predictor of all-cause mortality rate independent of BMI and body composition. These observations highlight the importance of taking a physical activity and exercise history during examination as well as emphasizing physical activity as a treatment approach.
Obesity-Associated Comorbid Conditions The evaluation of comorbid conditions should be based on presentation of symptoms, risk factors, and index of suspicion. For all patients, a fasting lipid panel should be performed (total, low-density lipoprotein, and high-density lipoprotein cholesterol and triglyceride levels) and a fasting blood glucose level and blood pressure determined. Symptoms and diseases that are directly or indirectly related to obesity are listed in Table 416-4. Although individuals vary, the number and severity of organ-specific comorbid conditions usually rise with increasing levels of obesity. Patients at very high absolute risk include those with the following: established coronary heart disease; presence of other atherosclerotic diseases, such as peripheral arterial disease, abdominal aortic aneurysm, and symptomatic carotid artery disease; type 2 diabetes; and sleep apnea.
OBESITY-RELATED ORGAN SYSTEMS REVIEW |
Assessing the Patient’s Readiness to Change An attempt to initiate lifestyle changes when the patient is not ready usually leads to frustration and may hamper future weight-loss efforts. Assessment includes patient motivation and support, stressful life events, psychiatric status, time availability and constraints, and appropriateness of goals and expectations. Readiness can be viewed as the balance of two opposing forces: (1) motivation, or the patient’s desire to change; and (2) resistance, or the patient’s resistance to change.
A helpful method to begin a readiness assessment is to use the motivational interviewing technique of “anchoring” the patient’s interest and confidence to change on a numerical scale. With this technique, the patient is asked to rate—on a scale from 0 to 10, with 0 being not so important (or confident) and 10 being very important (or confident)—his or her level of interest in and confidence about losing weight at this time. This exercise helps establish readiness to change and also serves as a basis for further dialogue.
TREATMENT | OBESITY |
THE GOAL OF THERAPY
The primary goals of treatment are to improve obesity-related comorbid conditions and to reduce the risk of developing future comorbidities. Information obtained from the history, physical examination, and diagnostic tests is used to determine risk and develop a treatment plan (Fig. 416-1). The decision of how aggressively to treat the patient and which modalities to use is determined by the patient’s risk status, expectations, and available resources. Not all patients who are deemed obese by BMI alone need to be treated, as exemplified by the concepts of obesity paradox or the metabolically healthy obese. However, patients who present with obesity-related comorbidities and who would benefit from weight loss intervention should be managed proactively. Therapy for obesity always begins with lifestyle management and may include pharmacotherapy or surgery, depending on BMI risk category (Table 416-5). Setting an initial weight-loss goal of 8–10% over 6 months is a realistic target.
FIGURE 416-1 Algorithm for the treatment of obesity. This algorithm applies only to assessment for overweight and obesity and subsequent decisions based on that assessment. It does not reflect initial overall assessment for other conditions that the physician may wish to perform. BMI, body mass index; Hx, history. (From the National, Heart, Lung, and Blood Institute: Clinical guidelines on the identification, evaluation, and treatment of overweight and obesity in adults: The evidence report. Washington, DC, US Department of Health and Human Services, 1998.)
A GUIDE TO OPTING FOR TREATMENT FOR OBESITY |
LIFESTYLE MANAGEMENT
Obesity care involves attention to three essential elements of lifestyle: dietary habits, physical activity, and behavior modification. Because obesity is fundamentally a disease of energy imbalance, all patients must learn how and when energy is consumed (diet), how and when energy is expended (physical activity), and how to incorporate this information into their daily lives (behavioral therapy). Lifestyle management has been shown to result in a modest (typically 3–5 kg) weight loss when compared with no treatment or usual care.
Diet Therapy The primary focus of diet therapy is to reduce overall calorie consumption. Guidelines from the National Heart, Lung, and Blood Institute recommend initiating treatment with a calorie deficit of 500–1000 kcal/d compared with the patient’s habitual diet. This reduction is consistent with a goal of losing ~1–2 lbs per week. The calorie deficit can be instituted through dietary substitutions or alternatives. Examples include choosing smaller portion sizes, eating more fruits and vegetables, consuming more whole-grain cereals, selecting leaner cuts of meat and skimmed dairy products, reducing consumption of fried foods and other foods with added fats and oils, and drinking water instead of sugar-sweetened beverages. It is important that dietary counseling remain patient centered and that the goals set be practical, realistic, and achievable.
The macronutrient composition of the diet will vary with the patient’s preference and medical condition. The 2010 U.S. Department of Agriculture Dietary Guidelines for Americans (Chap. 95e), which focus on health promotion and risk reduction, can be applied to treatment of overweight or obese patients. The recommendations include maintaining a diet rich in whole grains, fruits, vegetables, and dietary fiber; consuming two servings (8 oz) of fish high in omega 3 fatty acids per week; decreasing sodium intake to <2300 mg/d; consuming 3 cups of milk (or equivalent low-fat or fat-free dairy products) per day; limiting cholesterol intake to <300 mg/d; and keeping total fat intake at 20–35% of daily calories and saturated fat intake at <10% of daily calories. Application of these guidelines to specific calorie goals can be found on the website www.choosemyplate.gov. The revised Dietary Reference Intakes for Macronutrients released by the Institute of Medicine recommends that 45–65% of calories come from carbohydrates, 20–35% from fat, and 10–35% from protein. The guidelines also recommend daily fiber intake of 38 g (men) and 25 g (women) for persons over 50 years of age and 30 g (men) and 21 g (women) for those under age 50.
Since portion control is one of the most difficult strategies for patients to manage, the use of pre-prepared products such as meal replacements is a simple and convenient suggestion. Examples include frozen entrees, canned beverages, and bars. Use of meal replacements in the diet has been shown to result in a 7–8% weight loss.
Numerous randomized trials comparing diets of different macronutrient composition (e.g., low-carbohydrate, low-fat, Mediterranean) have shown that weight loss depends primarily on reduction of total caloric intake and adherence to the prescribed diet, not the specific proportions of carbohydrate, fat, and protein in the diet. The macronutrient composition will ultimately be determined by the patient’s taste preferences, cooking style, and culture. However, the patient’s underlying medical problems are also important in guiding the recommended dietary composition. The dietary prescription will vary according to the patient’s metabolic profile and risk factors. A consultation with a registered dietitian for medical nutrition therapy is particularly useful in considering patient preference and treatment of comorbid diseases.
Another dietary approach to consider is based on the concept of energy density, which refers to the number of calories (i.e., amount of energy) a food contains per unit of weight. People tend to ingest a constant volume of food regardless of caloric or macronutrient content. Adding water or fiber to a food decreases its energy density by increasing weight without affecting caloric content. Examples of foods with low-energy density include soups, fruits, vegetables, oatmeal, and lean meats. Dry foods and high-fat foods such as pretzels, cheese, egg yolks, potato chips, and red meat have a high-energy density. Diets containing low-energy-dense foods have been shown to control hunger and thus to result in decreased caloric intake and weight loss.
Occasionally, very low-calorie diets (VLCDs) are prescribed as a form of aggressive dietary therapy. The primary purpose of a VLCD is to promote a rapid and significant (13- to 23-kg) short-term weight loss over a 3- to 6-month period. The proprietary formulas designed for this purpose typically supply ≤800 kcal, 50–80 g of protein, and 100% of the recommended daily intake for vitamins and minerals. According to a review by the National Task Force on the Prevention and Treatment of Obesity, indications for initiating a VLCD include the involvement of well-motivated individuals who are moderately to severely obese (BMI, >30 kg/m2), have failed at more conservative approaches to weight loss, and have a medical condition that would be immediately improved with rapid weight loss. These conditions include poorly controlled type 2 diabetes, hypertriglyceridemia, obstructive sleep apnea, and symptomatic peripheral edema. The risk for gallstone formation increases exponentially at rates of weight loss >1.5 kg/week (3.3 lb/week). Prophylaxis against gallstone formation with ursodeoxycholic acid (600 mg/d) is effective in reducing this risk. Because of the need for close metabolic monitoring, VLCDs usually are prescribed by physicians specializing in obesity care.
Physical Activity Therapy Although exercise alone is only moderately effective for weight loss, the combination of dietary modification and exercise is the most effective behavioral approach for the treatment of obesity. The most important role of exercise appears to be in the maintenance of the weight loss. The 2008 Physical Activity Guidelines for Americans (www.health.gov/paguidelines) recommend that adults should engage in 150 min of moderate-intensity or 75 min a week of vigorous-intensity aerobic physical activity per week, performed in episodes of at least 10 min and preferably spread throughout the week. Focusing on simple ways to add physical activity into the normal daily routine through leisure activities, travel, and domestic work should be suggested. Examples include walking, using the stairs, doing housework and yard work, and engaging in sports. Asking the patient to wear a pedometer or accelerometer to monitor total accumulation of steps or kcal expended as part of the activities of daily living is a useful strategy. Step counts are highly correlated with activity level. Studies have demonstrated that lifestyle activities are as effective as structured exercise programs for improving cardiorespiratory fitness and weight loss. A high level of physical activity (>300 min of moderate-intensity activity per week) is often needed to lose weight and sustain weight loss. These exercise recommendations are daunting to most patients and need to be implemented gradually. Consultation with an exercise physiologist or personal trainer may be helpful.
Behavioral Therapy Cognitive behavioral therapy is used to help change and reinforce new dietary and physical activity behaviors. Strategies include self-monitoring techniques (e.g., journaling, weighing, and measuring food and activity); stress management; stimulus control (e.g., using smaller plates, not eating in front of the television or in the car); social support; problem solving; and cognitive restructuring to help patients develop more positive and realistic thoughts about themselves. When recommending any behavioral lifestyle change, the patient should be asked to identify what, when, where, and how the behavioral change will be performed. The patient should keep a record of the anticipated behavioral change so that progress can be reviewed at the next office visit. Because these techniques are time-consuming to implement, their supervision is often undertaken by ancillary office staff, such as a nurse-clinician or registered dietitian.
PHARMACOTHERAPY
Adjuvant pharmacologic treatments should be considered for patients with a BMI ≥30 kg/m2 or—for patients who have concomitant obesity-related diseases and for whom dietary and physical activity therapy has not been successful—a BMI ≥27 kg/m2. When an antiobesity medication is prescribed, patients should be actively engaged in a lifestyle program that provides the strategies and skills needed to use the drug effectively, since such support increases total weight loss.
Medications for obesity have traditionally fallen into two major categories: appetite suppressants (anorexiants) and gastrointestinal fat blockers. Appetite-suppressing medications have primarily targeted three monoamine receptor systems in the hypothalamus: noradrenergic, dopaminergic, and serotonergic receptors. Two new appetite suppressants were approved by the U.S. Food and Drug Administration (FDA) in 2012: lorcaserin and phentermine/topiramate (PHEN/TPM) extended release. Gastrointestinal fat blockers reduce the absorption of selective macronutrients, such as fat, from the gastrointestinal tract.
Centrally Acting Anorexiant Medications Anorexiants affect satiety (the absence of hunger after eating) and hunger (the biologic sensation that prompts eating). By increasing satiety and decreasing hunger, these agents help patients reduce caloric intake without a sense of deprivation. The target site for the actions of anorexiants is the ventromedial and lateral hypothalamic regions in the central nervous system (Chap. 415e). The biologic effect of these agents on appetite regulation is produced by augmentation of the neurotransmission of three monoamines: norepinephrine; serotonin (5-hydroxytryptamine, or 5-HT); and, to a lesser degree, dopamine. The classic sympathomimetic adrenergic agents (benzphetamine, phendimetrazine, diethylpropion, mazindol, and phentermine) function by stimulating norepinephrine release or by blocking its reuptake. Among the anorexiants, phentermine has been the most commonly prescribed; there is limited long-term data on its effectiveness. A 2002 review of six randomized, placebo-controlled trials of phentermine for weight control found that patients lost 0.6–6.0 additional kilograms of weight over 2–24 weeks of treatment. The most common side effects of the amphetamine-derived anorexiants are restlessness, insomnia, dry mouth, constipation, and increased blood pressure and heart rate.
PHEN/TPM is a combination drug that contains a catecholamine releaser (phentermine) and an anticonvulsant (topiramate). Topiramate is approved by the FDA as an anticonvulsant for the treatment of epilepsy and for the prophylaxis of migraine headaches. Weight loss was identified as an unintended side effect of topiramate during clinical trials for epilepsy. The mechanism responsible for weight loss is uncertain but is thought to be mediated through the drug’s modulation of γ-aminobutyric acid receptors, inhibition of carbonic anhydrase, and antagonism of glutamate. PHEN/TPM has undergone two 1-year pivotal randomized, placebo-controlled, double-blind trials of efficacy and safety: EQUIP and CONQUER. In a third study, SEQUEL, 78% of CONQUER participants continued to receive their blinded treatment for an additional year. All participants received diet and exercise counseling. Participant numbers, eligibility, characteristics, and weight loss outcomes are displayed in Table 416-6. Intention-to-treat 1-year placebo-subtracted weight loss for the PHEN/TPM 15-mg/92-mg dose was 9.3% and 8.6%, respectively, in the EQUIP and CONQUER trials. Clinical and statistical dose-dependent improvements were seen in selected cardiovascular and metabolic outcome measurements that were related to the weight loss. The most common adverse events experienced by the drug-randomized group were paresthesias, dry mouth, constipation, dysgeusia, and insomnia. Because of an increased risk of congenital fetal oral-cleft formation from topiramate, the FDA approval of PHEN/TPM stipulated a Risk Evaluation and Mitigation Strategies requirement to educate prescribers about the need for active birth control among women of childbearing age and a contraindication for use during pregnancy.
CLINICAL TRIALS FOR WEIGHT LOSS MEDICATIONSa |
Lorcaserin is a selective 5-HT2C receptor agonist with a functional selectivity ~15 times that of 5-HT2A receptors and 100 times that of 5-HT2B receptors. This selectivity is important, since the drug-induced valvulopathy documented with two other serotonergic agents that were removed from the market—fenfluramine and dexfenfluramine—was due to activation of the 5-HT2B receptors expressed on cardiac valvular interstitial cells. By activating the 5-HT2C receptor, lorcaserin is thought to decrease food intake through the pro-opiomelanocortin system of neurons.
Lorcaserin has undergone two randomized, placebo-controlled, double-blind trials for efficacy and safety. Participants were randomized to receive lorcaserin (10 mg bid) or placebo in the BLOOM study and to receive lorcaserin (10 mg bid or qd) or placebo in the BLOSSOM study. All participants received diet and exercise counseling. Participant numbers, eligibility, characteristics, and weight loss outcomes are displayed in Table 416-6. Overweight or obese subjects had at least one coexisting condition (hypertension, dyslipidemia, cardiovascular disease, impaired glucose tolerance, or sleep apnea)—medical conditions that are commonly seen in the office setting. Intention-to-treat 1-year placebo-subtracted weight loss was 3.6% and 3.0%, respectively, in the BLOOM and BLOSSOM trials. Echocardiography was performed at the screening visit and at scheduled time points over the course of the studies. There was no difference in the development of FDA-defined valvulopathy between drug-treated and placebo-treated participants at 1 year or 2 years. Modest statistical improvements consistent with the weight loss were seen in selected cardiovascular and metabolic outcome measurements. The most common adverse events experienced by the drug group were headache, dizziness, and nausea.
In approving both PHEN/TPM and lorcaserin, the FDA introduced a new provision with important clinical relevance: a prescription trial period to assess effectiveness. Response to both medications should be assessed after 3 months of treatment. For lorcaserin, the medication should be discontinued if the patient has not lost at least 5% of body weight by that point. For PHEN/TPM, if the patient has not lost at least 3% of body weight at 3 months, the clinician can either escalate the dose and reassess progress at 6 months or discontinue treatment entirely.
Peripherally Acting Medications Orlistat (XenicalTM) is a synthetic hydrogenated derivative of a naturally occurring lipase inhibitor, lipostatin, that is produced by the mold Streptomyces toxytricini. This drug is a potent, slowly reversible inhibitor of pancreatic, gastric, and carboxylester lipases and phospholipase A2, which are required for the hydrolysis of dietary fat into fatty acids and monoacylglycerols. Orlistat acts in the lumen of the stomach and small intestine by forming a covalent bond with the active site of these lipases. Taken at a therapeutic dose of 120 mg tid, orlistat blocks the digestion and absorption of ~30% of dietary fat. After discontinuation of the drug, fecal fat content usually returns to normal within 48–72 h.
Multiple randomized, double-blind, placebo-controlled studies have shown that, after 1 year, orlistat produces a weight loss of ~9–10%, whereas placebo recipients have a 4–6% weight loss. Because orlistat is minimally (<1%) absorbed from the gastrointestinal tract, it has no systemic side effects. The drug’s tolerability is related to the malabsorption of dietary fat and the subsequent passage of fat in the feces. Adverse gastrointestinal effects, including flatus with discharge, fecal urgency, fatty/oily stool, and increased defecation, are reported in at least 10% of orlistat-treated patients. These side effects generally are experienced early, diminish as patients control their dietary fat intake, and only infrequently cause patients to withdraw from clinical trials. When taken concomitantly, psyllium mucilloid is helpful in controlling orlistat-induced gastrointestinal side effects. Because serum concentrations of the fat-soluble vitamins D and E and β-carotene may be reduced by orlistat treatment, vitamin supplements are recommended to prevent potential deficiencies. Orlistat was approved for over-the-counter use in 2007.
Antiobesity Drugs in Development Two additional medications are currently in development. Bupropion and naltrexone (ContraveTM)—a dopamine and norepinephrine reuptake inhibitor and an opioid receptor antagonist, respectively—are theoretically combined to dampen the motivation/reinforcement that food brings (dopamine effect) and the pleasure/palatability of eating (opioid effect). In the COR-1 randomized, double-blind, placebo-controlled trial, 1742 enrolled participants, who were 18–65 years of age and had BMIs of 30–45 kg/m2, were randomized to receive naltrexone (16 mg/d) plus bupropion (360 mg/d), naltrexone (32 mg/d) plus bupropion (360 mg/d), or placebo. Mean change in body weight for the three groups was 5.0%, 6.1%, and 1.3%, respectively. The most common adverse events were nausea, headache, constipation, dizziness, vomiting, and dry mouth. However, the FDA rejected the drug in 2011 because of cardiovascular concerns and concluded that a large-scale study of the long-term cardiovascular effects of naltrexone would be needed before approval could be considered.
Liraglutide, a glucagon-like peptide 1 receptor agonist currently approved for the treatment of type 2 diabetes, has independent weight loss effects via hypothalamic neural activation causing appetite suppression. In a double-blind, placebo-controlled trial, 564 adults with BMIs of 30–40 kg/m2 were randomized to receive once-daily SC liraglutide (1.2, 1.8, 2.4, or 3.0 mg), placebo, or open-label orlistat (120 mg tid) for 1 year. The liraglutide and placebo recipients were switched to 2.4 mg of liraglutide during the second year and then to 3.0 mg for an additional year. One-year placebo-subtracted mean weight loss was 5.8 kg for liraglutide and 3.8 kg more than those on orlistat. The most common side effects were nausea, vomiting, and change in bowel habits.
SURGERY
Bariatric surgery (Fig. 416-2) can be considered for patients with severe obesity (BMI, ≥40 kg/m2) or for those with moderate obesity (BMI, ≥35 kg/m2) associated with a serious medical condition. Weight loss surgeries have traditionally been classified into three categories on the basis of anatomic changes: restrictive, restrictive malabsorptive, and malabsorptive. More recently, however, the clinical benefits of bariatric surgery in achieving weight loss and alleviating metabolic comorbidities have been attributed largely to changes in the physiologic responses of gut hormones and in adipose tissue metabolism. Metabolic effects resulting from bypassing the foregut include altered responses of ghrelin, glucagon-like peptide 1, peptide YY3-36, and oxyntonodulin. Additional effects on food intake and body weight control may be attributed to changes in vagal signaling. The loss of fat mass, particularly visceral fat, is associated with multiple metabolic, adipokine, and inflammatory changes that include improved insulin sensitivity and glucose disposal; reduced free fatty acid flux; increased adiponectin levels; and decreased interleukin 6, tumor necrosis factor α, and high-sensitivity C-reactive protein levels.
FIGURE 416-2 Bariatric surgical procedures. Examples of operative interventions used for surgical manipulation of the gastrointestinal tract. A. Laparoscopic adjustable gastric banding. B. Laparoscopic sleeve gastrectomy. C. The Roux-en-Y gastric bypass. D. Biliopancreatic diversion with duodenal switch. E. Biliopancreatic diversion. (From ML Kendrick, GF Dakin: Mayo Clin Proc 815:518, 2006; with permission.)
Restrictive surgeries limit the amount of food the stomach can hold and slow the rate of gastric emptying. Laparoscopic adjustable gastric banding is the prototype of this category. The first banding device, the LAP-BAND, was approved for use in the United States in 2001 and the second, the REALIZE band, in 2007. In contrast to previous devices, these bands have diameters that are adjustable by way of their connection to a reservoir that is implanted under the skin. Injection of saline into the reservoir and removal of saline from the reservoir tighten and loosen the band’s internal diameter, respectively, thus changing the size of the gastric opening. The mean percentage of total body weight lost at 5 years is estimated at 20–25%. In laparoscopic sleeve gastrectomy, the stomach is restricted by stapling and dividing it vertically, removing ~80% of the greater curvature, and leaving a slim banana-shaped remnant stomach along the lesser curvature. Weight loss after this procedure is superior to that after laparoscopic adjustable gastric banding.
The three restrictive-malabsorptive bypass procedures combine the elements of gastric restriction and selective malabsorption. These procedures are Roux-en-Y gastric bypass, biliopancreatic diversion, and biliopancreatic diversion with duodenal switch (Fig. 416-2). Roux-en-Y is the most commonly undertaken and most accepted bypass procedure. It may be performed with an open incision or by laparoscopy.
These procedures generally produce a 30–35% average total body weight loss that is maintained in nearly 60% of patients at 5 years. In general, mean weight loss is greater after the combined restrictive-malabsorptive procedures than after the restrictive procedures. Significant improvement in multiple obesity-related comorbid conditions, including type 2 diabetes, hypertension, dyslipidemia, obstructive sleep apnea, quality of life, and long-term cardiovascular events, has been reported. A meta-analysis of controlled clinical trials comparing bariatric surgery versus no surgery showed that surgery was associated with a reduced odds ratio (OR) risk of global mortality (OR = 0.55), cardiovascular death (OR = 0.58), and all-cause mortality (OR = 0.70).
Among the observed improvements in comorbidities, the prevention and treatment of type 2 diabetes resulting from bariatric surgery has garnered the most attention. Fifteen-year data from the Swedish Obese Subjects study demonstrated a marked reduction (i.e., by 78%) in the incidence of type 2 diabetes development among obese patients who underwent bariatric surgery. Several randomized controlled studies have shown greater weight loss and more improved glycemic control at 1 and 2 years among surgical patients than among patients receiving conventional medical therapy. A retrospective cohort study of more than 4000 adults with diabetes found that, overall, 68.2% of patients experienced an initial complete type 2 diabetes remission within 5 years after surgery. However, among these patients, one-third redeveloped type 2 diabetes within 5 years. The rapid improvement seen in diabetes after restrictive-malabsorptive procedures is thought to be due to surgery-specific, weight-independent effects on glucose homeostasis brought about by alteration of gut hormones.
The mortality rate from bariatric surgery is generally <1% but varies with the procedure, the patient’s age and comorbid conditions, and the experience of the surgical team. The most common surgical complications include stomal stenosis or marginal ulcers (occurring in 5–15% of patients) that present as prolonged nausea and vomiting after eating or inability to advance the diet to solid foods. These complications typically are treated by endoscopic balloon dilation and acid suppression therapy, respectively. For patients who undergo laparoscopic adjustable gastric banding, there are no intestinal absorptive abnormalities other than mechanical reduction in gastric size and outflow. Therefore, selective deficiencies are uncommon unless eating habits become unbalanced. In contrast, the restrictive-malabsorptive procedures carry an increased risk for micronutrient deficiencies of vitamin B12, iron, folate, calcium, and vitamin D. Patients with restrictive-malabsorptive procedures require lifelong supplementation with these micronutrients.
417 | Diabetes Mellitus: Diagnosis, Classification, and Pathophysiology |
Diabetes mellitus (DM) refers to a group of common metabolic disorders that share the phenotype of hyperglycemia. Several distinct types of DM are caused by a complex interaction of genetics and environmental factors. Depending on the etiology of the DM, factors contributing to hyperglycemia include reduced insulin secretion, decreased glucose utilization, and increased glucose production. The metabolic dysregulation associated with DM causes secondary pathophysiologic changes in multiple organ systems that impose a tremendous burden on the individual with diabetes and on the health care system. In the United States, DM is the leading cause of end-stage renal disease (ESRD), nontraumatic lower extremity amputations, and adult blindness. It also predisposes to cardiovascular diseases. With an increasing incidence worldwide, DM will be likely a leading cause of morbidity and mortality in the future.
CLASSIFICATION
DM is classified on the basis of the pathogenic process that leads to hyperglycemia, as opposed to earlier criteria such as age of onset or type of therapy (Fig. 417-1). There are two broad categories of DM, designated type 1 and type 2 (Table 417-1). However, there is increasing recognition of other forms of diabetes in which the pathogenesis is better understood. These other forms of diabetes may share features of type 1 and/or type 2 DM. Both type 1 and type 2 DM are preceded by a phase of abnormal glucose homeostasis as the pathogenic processes progress. Type 1 DM is the result of complete or near-total insulin deficiency. Type 2 DM is a heterogeneous group of disorders characterized by variable degrees of insulin resistance, impaired insulin secretion, and increased glucose production. Distinct genetic and metabolic defects in insulin action and/or secretion give rise to the common phenotype of hyperglycemia in type 2 DM and have important potential therapeutic implications now that pharmacologic agents are available to target specific metabolic derangements. Type 2 DM is preceded by a period of abnormal glucose homeostasis classified as impaired fasting glucose (IFG) or impaired glucose tolerance (IGT).
FIGURE 417-1 Spectrum of glucose homeostasis and diabetes mellitus (DM). The spectrum from normal glucose tolerance to diabetes in type 1 DM, type 2 DM, other specific types of diabetes, and gestational DM is shown from left to right. In most types of DM, the individual traverses from normal glucose tolerance to impaired glucose tolerance to overt diabetes (these should be viewed not as abrupt categories but as a spectrum). Arrows indicate that changes in glucose tolerance may be bidirectional in some types of diabetes. For example, individuals with type 2 DM may return to the impaired glucose tolerance category with weight loss; in gestational DM, diabetes may revert to impaired glucose tolerance or even normal glucose tolerance after delivery. The fasting plasma glucose (FPG), the 2-h plasma glucose (PG) after a glucose challenge, and the hemoglobin A1c (HbA1c) for the different categories of glucose tolerance are shown at the lower part of the figure. These values do not apply to the diagnosis of gestational DM. Some types of DM may or may not require insulin for survival. *Some use the term increased risk for diabetes or intermediate hyperglycemia (World Health Organization) rather than prediabetes. (Adapted from the American Diabetes Association, 2014.)
ETIOLOGIC CLASSIFICATION OF DIABETES MELLITUS |
Abbreviation: MODY, maturity-onset diabetes of the young.
Source: Adapted from American Diabetes Association: Diabetes Care 37(Suppl 1):S14, 2014.
Two features of the current classification of DM merit emphasis from previous classifications. First, the terms insulin-dependent diabetes mellitus (IDDM) and non-insulin-dependent diabetes mellitus (NIDDM) are obsolete. Because many individuals with type 2 DM eventually require insulin treatment for control of glycemia, the use of the term NIDDM generated considerable confusion. A second difference is that age or treatment modality is not a criterion. Although type 1 DM most commonly develops before the age of 30, an autoimmune beta cell destructive process can develop at any age. It is estimated that between 5 and 10% of individuals who develop DM after age 30 years have type 1 DM. Although type 2 DM more typically develops with increasing age, it is now being diagnosed more frequently in children and young adults, particularly in obese adolescents.
OTHER TYPES OF DM
Other etiologies for DM include specific genetic defects in insulin secretion or action, metabolic abnormalities that impair insulin secretion, mitochondrial abnormalities, and a host of conditions that impair glucose tolerance (Table 417-1). Maturity-onset diabetes of the young (MODY) and monogenic diabetes are subtypes of DM characterized by autosomal dominant inheritance, early onset of hyperglycemia (usually <25 years; sometimes in neonatal period), and impaired insulin secretion (discussed below). Mutations in the insulin receptor cause a group of rare disorders characterized by severe insulin resistance.
DM can result from pancreatic exocrine disease when the majority of pancreatic islets are destroyed. Cystic fibrosis–related DM is an important consideration in that patient population. Hormones that antagonize insulin action can also lead to DM. Thus, DM is often a feature of endocrinopathies such as acromegaly and Cushing’s disease. Viral infections have been implicated in pancreatic islet destruction but are an extremely rare cause of DM. A form of acute onset of type 1 diabetes, termed fulminant diabetes, has been noted in Japan and may be related to viral infection of islets.
GESTATIONAL DIABETES MELLITUS
Glucose intolerance developing during pregnancy is classified as gestational diabetes mellitus (GDM). Insulin resistance is related to the metabolic changes of late pregnancy, and the increased insulin requirements may lead to IGT or diabetes. GDM occurs in ~7% (range 1–14%) of pregnancies in the United States; most women revert to normal glucose tolerance postpartum but have a substantial risk (35–60%) of developing DM in the next 10–20 years. The International Association of the Diabetes and Pregnancy Study Groups and the American Diabetes Association (ADA) recommend that diabetes diagnosed at the initial prenatal visit should be classified as “overt” diabetes rather than GDM. With the rising rates of obesity, the number of women being diagnosed with GDM or overt diabetes is rising worldwide.
EPIDEMIOLOGY AND GLOBAL CONSIDERATIONS
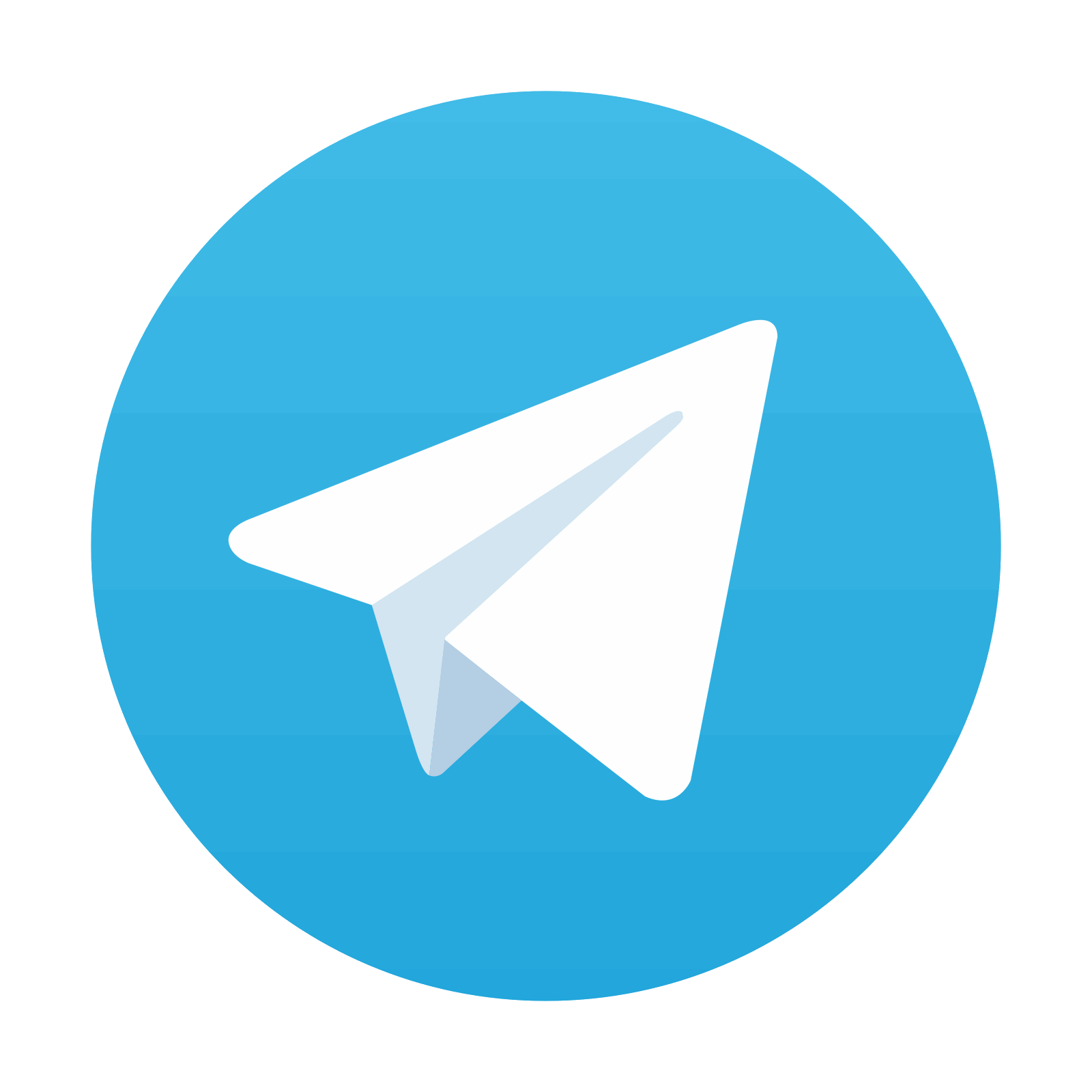
Stay updated, free articles. Join our Telegram channel

Full access? Get Clinical Tree
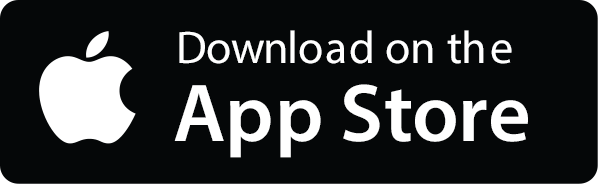
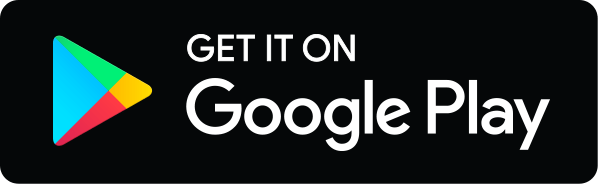