5′ fashion by the concerted activities of the degradosome-associated exoribonuclease PNPase and RhlB RNA helicase or by degradosome-independent 3′ 5′ exoribonucleases, such as RNase II and RNase R.12,13,14,15,16, Other endoribonucleases also contribute to mRNA degradation, including RNase G, RNase I, and RNase III.17,18,19 Most of these RNases cannot degrade to the single nucleotide, resulting in short RNA fragments that are further broken down by the enzyme Oligoribonuclease (Orn; 3′
5′ exoribonuclease).20 Additionally, the endoribonuclease RNase P is known to cleave mRNA transcripts that contain riboswitches and can cleave near stem-loop structures within E. coli mRNAs.21,22,23 Resulting cleavage products contain a 5′ loop structure that acts to stabilize select transcripts.23 RNase E, Orn, and RNase P are essential enzymes in the Gram-negative model organism E. coli, thus they may be good antibiotic drug discovery targets.24,25,26
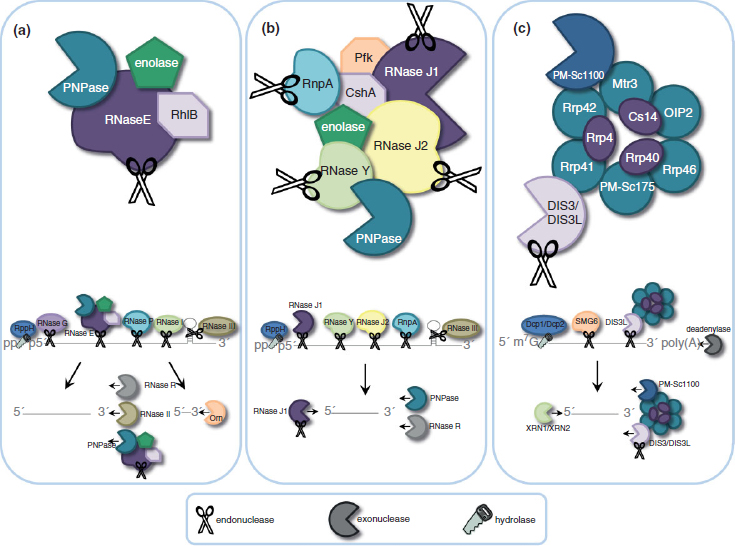
FIGURE 1 | Mechanisms of mRNA decay. (a) Model of mRNA degradosome and degradation pathways in Escherichia coli. The E. coli degradosome includes RNA helicase B (RhlB), enolase, polynucleotide phosphorylase (PNPase), and RNase E. Initiation of mRNA decay occurs with the internal cleavage by RNase E. This cleavage favors 5′ monophosphorylated transcripts, which is achieved through the action of RppH. Degradosome-independent endoribonucleases RNase G, RNase P, and RNase I cleave single-stranded RNA (ssRNA), while RNase III recognizes and cleaves double-stranded RNA secondary structures (dsRNA). Resulting cleavage products are further digested by the degradosome PNPase or by the action of RNase R and RNase II in a 3′ 5′ manner into fragments that are degraded into single nucleotides by the 3′
5′ endoribonuclease Orn. (b) Proposed model of mRNA degradosome-like complex in Staphylococcus aureus. The Gram-positive degradosome-like complex includes RNase J1, RNase J2, RNase Y (also known as CvfA and YmdA), enolase, RNA helicase (CshA), PNPase, phosphofructokinase (Pfk), and RnpA. In Gram-positive bacteria, internal cleavage by RNase J1 initiates mRNA degradation. RNase J1 preferentially cleaves 5′ monophosphorylated mRNA molecules that have been stripped of pyrophosphate by RppH. Other members of the degradosome, including RNase Y, RNase J2, and RnpA, also cleave transcripts endonucleolytically. Secondary dsRNA structures are recognized and cleaved by the endoribonuclease RNase III. Resulting RNA pieces are then degraded in a 3′
5′ fashion by the degradosome member PNPase and the degradosome-independent RNase R. Fragments are then broken down in the 5′
3′ direction by RNase J1. (c) The human exosome contains two structures: a ring structure composed of Rrp41, Rrp42, Mtr3, OIP2, Rrp46, and PM-Scl75, and a cap structure containing Rrp4, Rrp40, and Csl4. These exosome core components associate with the 3′
5′ exoribonucleases DIS3 and PM-Scl100 in the nucleus, or the dual endo- and 3′
5′ exo-ribonuclease DIS3L in the cytoplasm. Degradation of mRNA is initiated by removal of the poly(A) tail by deadenylase activity, decapping of the 5′ end by the Dcp1/Dcp2 complex, or by the endoribonucleolytic activity of the exosome-associated cytoplasmic DIS3L. The resulting mRNA is then vulnerable to 3′
5′ degradation by the exosome-associated PM-Scl100, DIS3, or DIS3L, and 5′
3′ decay by the exoribonucleases XRN2 (nucleus) or XRN1 (cytoplasm).
Researchers have long speculated that mRNA degradation within Gram-positive bacteria is also mediated by an RNA degradosome. However, the absence of an RNase E ortholog has thwarted efforts to identify this complex. Only recently, studies have revealed that Bacillus subtilis and Staphylococcus aureus are indeed capable of forming degradosome-like complexes consisting of at least eight subunits, including RNase J1, RNase J2, RNase Y (also known as CvfA and YmdA), enolase, RNA helicase (CshA), PNPase, phosphofructokinase (Pfk), and the protein component of the ribonucleoprotein complex RNase P, RnpA (Figure 1(b)).27,28 Current studies have begun to unravel the mechanism(s) by which components of the Gram-positive degradosome subunits contribute to mRNA decay. Those studies have predominantly focused on understanding RNA degradation in the Gram-positive model organism, B. subtilis, where RNase J1, which exhibits endo- and 5′ 3′ exo-ribonuclease activities, is likely to initiate degradation. Internal cleavage is thought to be initiated by RNase J1 and resulting fragments are subsequently exonucleolytically digested by RNase J1 in the 5′
3′ direction in concert with PNPase and CshA in the 3′
5′ direction.27,28,29 Like RNase E, RNase J1 preferentially cleaves 5′ monophosphorylated mRNA, and an RppH equivalent (BsRppH or YtkD) has been identified in B. subtilis.30 Another essential component of the B. subtilis degradosome-like complex is RNase Y, an endoribonuclease that contributes to bulk RNA degradation and has also been hypothesized to be the functional equivalent to the E. coli RNase E.31,32 B. subtilis transcripts are also degraded by a combination of other endoribonucleases, such as RNase J2, and the degradosome-independent RNase III.33 Additionally, RNase P which is a ribonucleoprotein complex composed of an RNA subunit (RnpB) and a protein subunit (RnpA) affects the mRNA turnover properties of specific B. subtilis transcripts.21,34,35 Studies in S. aureus have revealed that the protein component of RNase P, RnpA, affects bulk cellular mRNA turnover, as RnpA-depleted cells show increased mRNA stability, suggesting that RnpA acts to globally destabilize transcripts, albeit through an unknown mechanism.36 RnpA may be an essential member of the degradosome-like complex in both B. subtilis and S. aureus.28
When comparing the Gram-positive B. subtilis and S. aureus mRNA degradosomes, the components are conserved, however the interactions between their subunits vary, and they have different physiological characteristics. For instance, B. subtilis RNase Y is an essential enzyme, but it is not required for S. aureus viability; conversely, RNase J2 is an essential S. aureus gene but allelic deletions in B. subtilis are not lethal.37,38,39 Thus, while the overarching mechanisms by which these two Gram-positive bacteria degrade RNA molecules may be conserved, the subunits’ behaviors and properties are likely to differ. In that regard, given that the latter is a life-threatening human pathogen, the essential components of the S. aureus degradosome may serve as the more practical and effective targets for antibiotic development. S. aureus RNase J1, RNase J2, and RnpA, are essential members of the organism’s RNA degradation apparatus and may represent antibiotic targets.
Eukaryotic cells are highly specialized and compartmentalized, and as such, individual steps in the mRNA degradation pathway occur in distinct locations within the cell and are carried out by correspondingly unique combinations of RNases.9,10 Human mRNA is synthesized, 5′ m7G capped, and 3′ polyadenylated within the nucleus. Degradation of the mRNA molecule can occur within the nucleus or at any point during or after transport to the cytoplasm by endonucleolytic cleavage, 5′ decapping (decapping enzymes Dcp1/Dcp2), and/or removal of the 3′ poly(A) tail by a variety of unique deadenylases (PAN2–PAN3, CCR4–NOT, and poly(A)-specific ribonuclease (PARN)), each of which have varying roles in mRNA deadenylation among eukaryotes.10 These events in turn create substrates that are susceptible to 5′ 3′ exoribonucleases, such as XRN2 (nucleus) and XRN1 (cytoplasm), or decay in the 3′
5′ direction via the exosome (Figure 1(c)).10 The core of the exosome contains two distinct structures formed by nine subunits and is thought to be essential within humans.40,41 The RNA-binding cap structure (Rrp4, Rrp40, and Csl4) recognizes the mRNA substrate and passes it through the hexameric ring composed of PM-Scl75 (Rrp45), Rrp41, Rrp42, Mtr3, OIP2 (Rrp43), and Rrp46.40,42 Although the ring structure possesses conserved exoribonuclease domains (similar to bacterial PNPase and RNase PH), it has lost the ability to directly cleave RNA.9 Instead, the exosome coordinates the assembly of 3′
5′ exoribonucleases PM-Scl100 (Rrp6) and DIS3 in the nucleus, or the 3′
5′ exo- and endo-ribonuclease DIS3L in the cytoplasm, and as a unit with these RNases, degrades mRNA.41,43,44,45,46 Although the exosome accounts for the majority of mRNA turnover, internal cleavage of the transcript can also occur via endoribonucleases (SMG6), ribozymes, and the RNA interference pathway, each of which produces substrates that are susceptible to 5′
3′ and 3′
5′ exoribonucleases.6
EXPLOITING ESSENTIAL mRNA TURNOVER MACHINERY IN BACTERIA
As previously mentioned, ideal antibiotics exhibit broad-spectrum antimicrobial activity against an expansive repertoire of bacterial pathogens and obviously must not be toxic to the host. In that regard, even a superficial understanding of the bacterial and host mRNA turnover pathways illustrates that many of the essential RNases involved in bacterial mRNA decay act via endonucleolytic cleavage, while eukaryotic decay occurs predominantly in an exonucleolytic fashion. Thus, development of agents that inhibit essential bacterial endonucleases would prevent bacterial proliferation and would be less likely to affect human mRNA turnover processes. Accordingly, Table 1 provides a comparison of the known RNases for each of the ESKAPE bacterial pathogens. Separated by Gram-stain categorization, the table compares the amino acid conservation of each RNase across pathogens (percent identity listed in parentheses), its essentiality (if known), as well as the percent predicted amino acid identity of each RNase to orthologous human enzymes. A survey of these data brings to light several observations. First, there is no RNase antibiotic-development target that is essential, highly conserved across each of the ESKAPE pathogens, and also lacks similarity to human enzymes. Second, subdividing the ESKAPE pathogens based on very granular evolutionary boundaries, such as cell wall composition (Gram-staining), provides several putative RNase therapeutic targets that are essential, have low similarity to human proteins, and are well conserved across Gram-negative (Table 1(A)) or Gram-positive (Table 1(B)) organisms. Thus, one could ostensibly develop antimicrobials targeting these RNases; such agents may not be broad spectrum in the strictest sense, rather they would likely be efficacious across pathogenic species belonging to a given Gram-stain-defined boundary. There may be advantages to this approach, as the concept of targeting a subset of bacteria has been predicted to more beneficial than ‘broad-spectrum’ antibiotics. For instance, narrow-spectrum agents may spare the host’s normal bacterial flora and reduce selective pressure, thereby minimizing the development of resistance.4 In that regard, antimicrobial agents that target essential RNases may provide a perfect blend of broadly exhibiting efficacy against a Gram-stain-specific set of bacterial pathogens and avoiding the side effects of truly ‘broad spectrum’ agents. Table 1 indicates that three essential RNases are conserved across ESKAPE pathogens belonging to a given Gram-stain designation, each of which also exhibits limited or no sequence and/or functional conservation to members of the human RNA turnover machinery. These RNases may represent excellent antibiotic targets and include the Gram-negative RNase E, the Gram-positive RNase J1, and the protein component of RNase P, RnpA, found in both Gram types.
TABLE 1 | ESKAPE Pathogen Ribonucleases
As stated above, RNase E is thought to play a key role in mediating Gram-negative bacterial mRNA degradation. As the central component of the degradosome, it is essential for mRNA turnover, yet it is also required for rRNA and tRNA processing.12,62,63 The enzyme is also well conserved across Gram-negative bacteria (Table 1(A)). Furthermore, when comparing E. coli RNase E to the human genome, there is no significant amino acid homology to human proteins. Thus a small molecule inhibitor of RNase E would presumably exhibit antimicrobial activity against a repertoire of Gram-negative bacterial pathogens with no predicted human toxicity.
Two Gram-positive RNases with considerable potential as antimicrobial development targets are RNase J1 and the protein component of RNase P (RnpA), both of which are essential components of the S. aureus degradosome-like complex (Table 1(B)). RNase J1 is hypothesized to be the functional analog to the E. coli RNase E,37 which in addition to its endonucleolytic activity, is also a 5′ 3′ exoribonuclease able to degrade down to single nucleotides.64,65 RNase J1 is similar to RNase J2 in sequence and activity, however the essentiality of RNase J2 varies among bacterial species, suggesting that RNase J1 is the better target for antimicrobial development. RNase P is a ubiquitous ribonucleoprotein whose composition differs between host and pathogen.35 Bacterial RNase P is composed of one protein (RnpA) and one RNA subunit (RnpB), whereas human nuclear RNase P contains an RNA component and up to ten different protein subunits (Rpp14, Rpp20, Rpp21, Rpp25, Rpp29, Rpp30, Rpp38, Rpp40, Pop1, and Pop5) that do not share significant amino acid similarity to bacterial RnpA.66
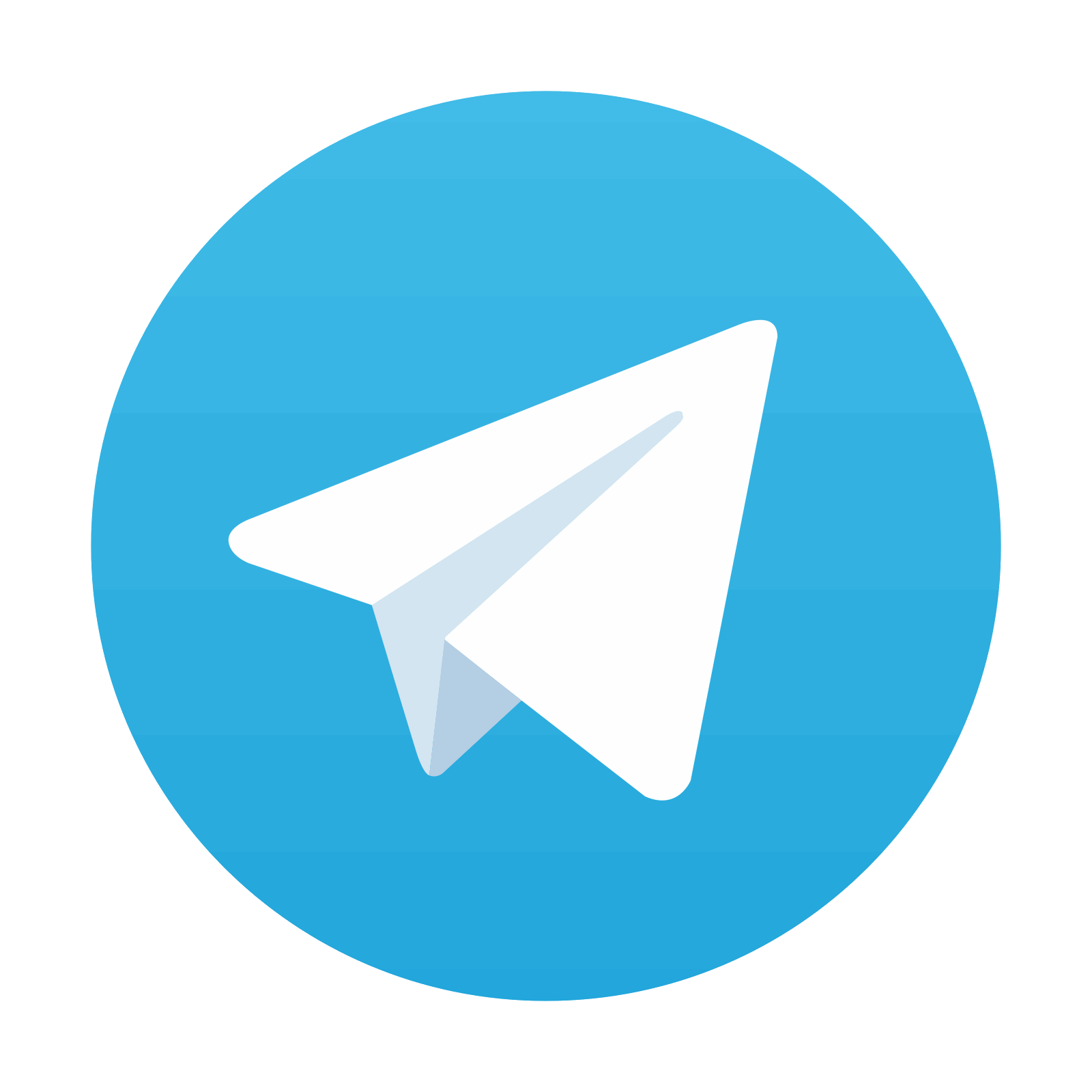
Stay updated, free articles. Join our Telegram channel

Full access? Get Clinical Tree
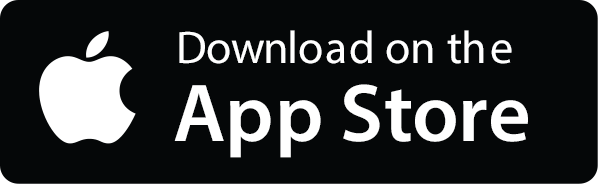
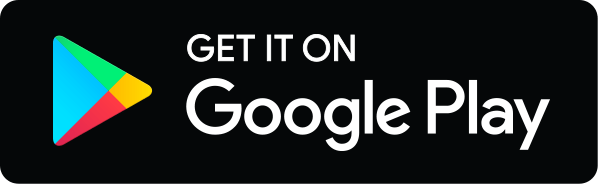