30
Conversion of Amino Acids to Specialized Products
OBJECTIVES
After studying this chapter, you should be able to:
Describe how amino acids participate in a variety of biosynthetic processes other than protein synthesis.
Outline how arginine participates in the biosynthesis of creatine, nitric oxide (NO), putrescine, spermine, and spermidine.
Indicate the contribution of cysteine and of β-alanine to the structure of coenzyme A and of cysteine to the structure of taurocholic acid.
Discuss the role of glycine in drug catabolism.
Document the role of glycine in the biosynthesis of heme, purines, creatine, and sarcosine.
Identify the reaction that converts an amino acid to the neurotransmitter histamine.
Document the role of S-adenosylmethionine as a source of methyl groups in metabolism.
Recognize the tryptophan metabolites serotonin and melatonin, and the conversion of tryptophan to tryptamine and subsequently to indole 3-acetate.
Indicate the role of tyrosine in the formation of norepinephrine, epinephrine, triiodothyronine, and thyroxine.
Illustrate the key roles of peptidyl serine, threonine and tyrosine in metabolic regulation and signal transduction pathways.
Outline the roles of glycine, arginine, and S-adenosylmethionine in the biosynthesis of creatine.
Describe the role of creatine phosphate in energy homeostasis.
Describe the formation of γ-aminobutyrate (GABA) and the rare metabolic disorders associated with defects in GABA catabolism.
BIOMEDICAL IMPORTANCE
Certain proteins contain amino acids that have been post-translationally modified to permit them to perform specific functions. Examples include the carboxylation of glutamate to form γ-carboxyglutamate, which functions in Ca2+ binding, the hydroxylation of proline for incorporation into the collagen triple helix, and the hydroxylation of lysine to hydroxylysine, whose subsequent modification and cross-linking stabilizes maturing collagen fibers. In addition to serving as the building blocks for protein synthesis, amino acids serve as precursors of biologic materials such as heme, purines, pyrimidines, hormones, neurotransmitters, and biologically active peptides. Histamine plays a central role in many allergic reactions. Neurotransmitters derived from amino acids include γ-aminobutyrate, 5-hydroxytryptamine (serotonin), dopamine, norepinephrine, and epinephrine. Many drugs used to treat neurologic and psychiatric conditions act by altering the metabolism of these neurotransmitters. Discussed below are the metabolism and metabolic roles of selected α- and non-α amino acids.
L-α-AMINO ACIDS
Alanine
Alanine serves as a carrier of ammonia and of the carbons of pyruvate from skeletal muscle to liver via the Cori cycle (see Figure 20–4), and together with glycine constitutes a major fraction of the free amino acids in plasma.
Arginine
Figure 30–1 summarizes the metabolic fates of arginine. In addition to serving as a carrier of nitrogen atoms in urea biosynthesis (see Figure 28–13), the guanidino group of arginine is incorporated into creatine, and following conversion to ornithine, its carbon skeleton becomes that of the polyamines putrescine and spermine.
FIGURE 30–1 Arginine, ornithine, and proline metabolism. Reactions with solid arrows all occur in mammalian tissues. Putrescine and spermine synthesis occurs in both mammals and bacteria. Arginine phosphate of invertebrate muscle functions as a phosphagen analogous to creatine phosphate of mammalian muscle.
The reaction catalyzed by NO synthase (Figure 30–2), a five-electron oxidoreductase with multiple cofactors, converts one nitrogen of the guanidine group of arginine to citrulline and NO, an intercellular signaling molecule that serves as a neurotransmitter, smooth muscle relaxant, and vasodilator (see Chapter 49).
FIGURE 30–2 The reaction catalyzed by nitric oxide synthase.
Cysteine
Cysteine participates in the biosynthesis of coenzyme A (see Figure 44–18) by reacting with pantothenate to form 4-phosphopantothenoyl-cysteine (Figure 30–3). Three enzyme-catalyzed reactions convert cysteine to taurine, which can displace the coenzyme A moiety of cholyl-CoA to form the bile acid taurocholic acid (see Figure 26–7). The conversion of cysteine to taurine is initiated by its oxidation to cysteine sulfinate, catalyzed by the nonheme Fe2+ enzyme cysteine dioxygenase. Decarboxylation of cysteine sulfinate by cysteine sulfinate decarboxylase forms hypotaurine, whose oxidation by hypotaurine dehydrogenase forms taurine (Figure 30–4).
FIGURE 30–3 The reaction catalyzed by phosphopantothenate cysteine ligase.
FIGURE 30–4 Conversion of cysteine to taurine. The reactions are catalyzed by cysteine dioxygenase, cysteine sulfinate decarboxylase, and hypotaurine decarboxylase, respectively.
Glycine
Metabolites and pharmaceuticals excreted as water-soluble glycine conjugates include glycocholic acid (see Chapter 26) and hippuric acid formed from the food additive benzoate (Figure 30–5). Many drugs, drug metabolites, and other compounds with carboxyl groups are excreted in the urine as glycine conjugates. Glycine is incorporated into creatine, and the nitrogen and α-carbon of glycine are incorporated into the pyrrole rings and the methylene bridge carbons of heme (see Chapter 31), and the entire glycine molecule becomes atoms 4, 5, and 7 of purines (see Figure 33–1).
FIGURE 30–5 Biosynthesis of hippurate. Analogous reactions occur with many acidic drugs and catabolites.
Histidine
Decarboxylation of histidine by the pyridoxal 5′-phosphate-dependent enzyme histidine decarboxylase forms histamine (Figure 30–6). A biogenic amine that functions in allergic reactions and gastric secretion, histamine is present in all tissues. Its concentration in the brain hypothalamus varies in accordance with a circadian rhythm. Histidine compounds present in the human body include ergothioneine, carnosine, and dietary anserine (Figure 30–7).
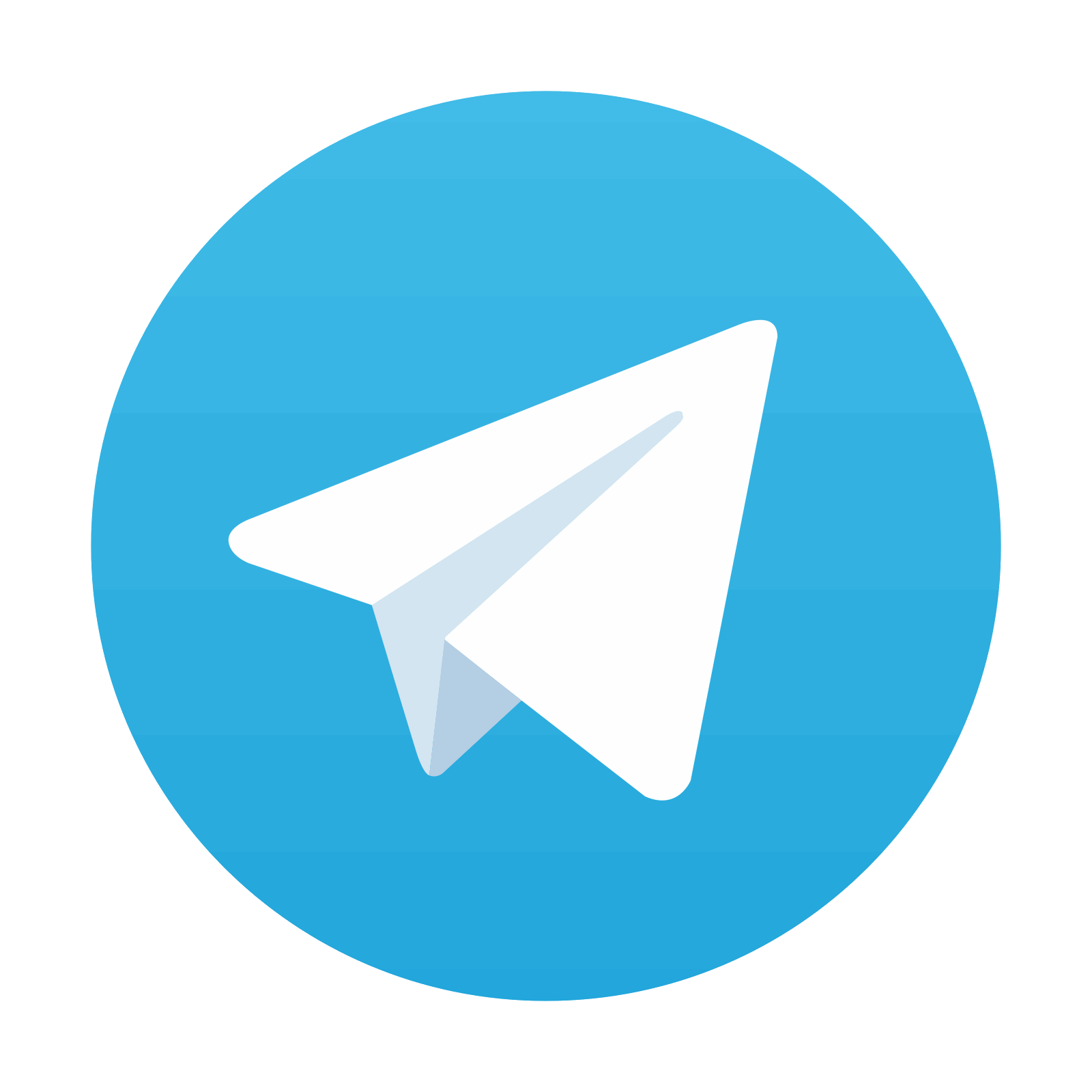
Stay updated, free articles. Join our Telegram channel

Full access? Get Clinical Tree
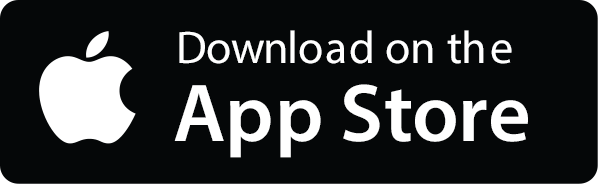
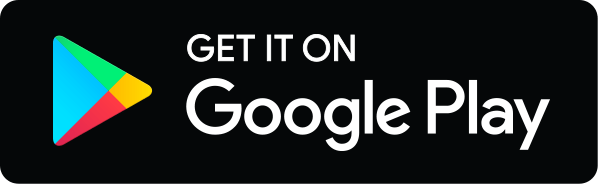