Control of Food Intake and Appetite1
Syed Sufyan Hussain
Akila De Silva
Stephen Robert Bloom
1Abbreviations: 2-AG, 2-arachidonoylglycerol; α-MSH, α-melanocyte-stimulating hormone; AEA, anandamide; AgRP, Agouti-related peptide; AMP, adenosine monophosphate; AP, area postrema; ARC, arcuate nucleus; ATP, adenosine triphosphate; BDNF, brain-derived neurotrophic factor; CART, cocaine- and amphetamine-regulated transcript; CB1, cannabinoid receptor type 1; CCK, cholecystokinin; CNTF, ciliary neurotrophic factor; DMN, dorsomedial nucleus; DVC, dorsal vagal complex; DVN, dorsal motor nucleus of the vagus; GHS-R, growth hormone secretagogue receptor; GLP-1, glucagon-like peptide-1; LCFA, long-chain fatty acids; LepR, leptin receptor; LHA, lateral hypothalamic area; MC3R, melanocortin receptor 3; MC4R, melanocortin receptor 4; MCH, melanin-concentrating hormone; NPY, neuropeptide Y; NTS, nucleus of the tractus solitarius; OFC, orbitofrontal cortex; OXA, orexin A; OXB, orexin B; OXM, oxyntomodulin; PFA, perifornical area; POMC, pro-piomelanocortin; PP, pancreatic polypeptide; PVN, paranucleus; PYY, peptide YY; TRH, thyrotropin-releasing hormone; VMN, ventromedial nucleus.
Traditionally, a distinction has been made between homeostatic and nonhomeostatic control of appetite and food intake. Homeostatic control refers to the alteration in consumption of food that follows sensing of energy balance. Following a meal, changes in circulating concentration of nutrients, in addition to activation of signaling pathways from the gut, lead to a reduction in subsequent feeding. Furthermore, adiposity signals that form long-term markers of energy balance also comprise important homeostatic controls of food intake. Appropriately matching food intake with metabolic need is achieved by the interaction of these inputs with key central neural appetite circuits.
Across species, food intake is also driven by factors other than basic physiologic or homeostatic need. Food appearance, flavor, timing and location of meals, and social, cultural, emotional, and economic influences affect food intake by nonhomeostatic mechanisms. Furthermore, nonhomeostatic control of food intake is modulated by hedonic mechanisms, reward pathways, and previous experience with food (mnemonic pathways).
Our understanding of the overall control of food intake and appetite has expanded, leading to the modern viewpoint that the distinction between homeostatic and nonhomeostatic pathways is, in fact, less rigid. Indeed, investigators recognize that nonhomeostatic pathways themselves modulate homeostatic pathways and lead to a network of hormonal and neural communication upstream of the overall outcome of feeding (Fig. 44.1).
CENTRAL CONTROLS OF FOOD INTAKE AND APPETITE
Coordination by the Hypothalamus
The hypothalamus is widely recognized as the “gate keeper” in the control of food intake and appetite. Peripheral signals of energy balance may act directly on the hypothalamus to control food intake, and this forms one important focus of current appetite research. Historically, the lateral hypothalamus was thought of as the “hunger center,” and the medial hypothalamus was the “satiety center.” This concept was based on lesioning experiments in animals; damage to the lateral hypothalamus produced anorexia, whereas damage to the medial hypothalamus led to hyperphagia (1). Although this view still largely holds true, it has been
refined by our enhanced understanding of the role of individual nuclei within the hypothalamus and communication among them. The presence of a network of communication among the gut, pancreas, adipose tissue, brainstem, and hypothalamus to signal energy balance is also well established. Additionally, further communication exists between the hypothalamus and higher cortical centers pertaining to food memory and rewarding aspects of food, with resulting overall coordinated control of food intake.
refined by our enhanced understanding of the role of individual nuclei within the hypothalamus and communication among them. The presence of a network of communication among the gut, pancreas, adipose tissue, brainstem, and hypothalamus to signal energy balance is also well established. Additionally, further communication exists between the hypothalamus and higher cortical centers pertaining to food memory and rewarding aspects of food, with resulting overall coordinated control of food intake.
Role of the Brainstem
The brainstem has a well-established role in the sensing of energy balance and modulation of food intake. Within the brainstem, the dorsal vagal complex (DVC) is the main organ responsible for facilitating the communication between peripheral signals of food intake and hypothalamic nuclei (2). The DVC consists of the nucleus of the tractus solitarius (NTS), the area postrema (AP), and the dorsal motor nucleus of the vagus (DVN). Vagal nerve afferents carry sensory information relaying hunger and satiety from the gut directly to the NTS. Transection of these gut sensory vagal nerve afferents results in increased meal size and duration (3). The absence of a complete blood-brain barrier in the AP also allows the brainstem to receive metabolic signals of energy balance (e.g., hormones and nutrients carried by the blood) directly.
The brainstem is then able to process these sensory inputs and relay them to the hypothalamus and higher cortical centers. In keeping with this, it is well established that neural projections are present from the brainstem to the hypothalamus (4). Efferent pathways also descend from the hypothalamus to the DVN (5). The DVN modulates efferent vagal nerve activity in the gastrointestinal tract and can alter gastric emptying, gastric motility, and pancreatic secretions. This function points to a role for the brainstem in modulating feeding-related activity as well as sensing energy balance.
Hypothalamic Nuclei Implicated in the Control of Food Intake
The arcuate nucleus (ARC) is thought to be the main hypothalamic area controlling food intake. It lies adjacent to the third ventricle and close to the median eminence, where an incomplete blood-brain barrier is thought to allow peripheral signals to gain access to the central nervous system. In mice, lesions of the ARC result in hyperphagia and obesity (6). Within the ARC, two groups of neurons are pivotal in regulating food intake. One group of neurons contains neuropeptide Y (NPY), and most of these also contain
Agouti-related peptide (AgRP). Activation of these neurons enhances food intake (i.e., these neurons are orexigenic). The second group is formed by neurons containing pro-opiomelanocortin (POMC) and cocaine- and amphetamineregulated transcript (CART). Activation of these neurons reduces food intake (i.e., these neurons are anorexigenic).
Agouti-related peptide (AgRP). Activation of these neurons enhances food intake (i.e., these neurons are orexigenic). The second group is formed by neurons containing pro-opiomelanocortin (POMC) and cocaine- and amphetamineregulated transcript (CART). Activation of these neurons reduces food intake (i.e., these neurons are anorexigenic).
Axons from NPY/AgRP and POMC/CART, neurons project from the ARC to other areas of the hypothalamus such as the paraventricular nucleus (PVN). Destruction of the PVN leads to hyperphagia and obesity in rats (7). In addition to the PVN, axons from the ARC also project to the ventromedial nucleus (VMN), dorsomedial nucleus (DMN), lateral hypothalamic area (LHA), and perifornical area (PFA) to modulate food intake.
Neuropeptides Implicated in the Control of Food Intake
Neuropeptide Y
NPY is the most powerful central stimulant of appetite, and most neurons expressing NPY are found within the ARC. Approximately 90% of NPY neurons coexpress AgRP. Central administration of NPY enhances food intake in rats (8). Furthermore, repeated daily injections of NPY into the hypothalamus result in chronic hyperphagia and weight gain in these animals (9). Conversely, ablation of NPY/AgRP neurons in mice leads to reduced body weight via reduced food intake (10). Of the six identified NPY receptors, the Y1 and Y5 receptors seem to mediate the orexigenic effect of NPY. Additionally, there appears to be local inhibition of anorexigenic POMC neurons in the ARC (11). NPY/AgRP neurons project within the hypothalamus from the ARC, to nuclei including the PVN, DMN, and LHA. In the PVN, direct stimulation of Y1 and Y5 receptors is thought to occur to increase food intake, in addition to inhibiting anorexigenic pathways by AgRP.
Agouti-Related Peptide
Pro-opiomelanocortin and Melanocortins
POMC is the precursor of α-melanocyte-stimulating hormone (α-MSH). α-MSH is produced by cleavage of POMC and binds to the G-protein-coupled melanocortin receptor 4 (MC4R), which is highly represented in the hypothalamus, particularly in the PVN. α-MSH binding to the MC4R acts to reduce food intake (13). Consistent with this, mice lacking all POMC-derived peptides are hyperphagic and obese (14), as are MC4R knockout mice (15). In humans, nearly 100 different mutations of the MC4R gene are responsible for more than 5% of cases of morbid nonsyndromic obesity, and these individuals are hyperphagic (16). Furthermore, homozygous mutations in the POMC gene in humans result in early-onset obesity (17).
Diet-induced obesity in rats leads to up-regulation of POMC, followed by a reduction in food intake. This resultant anorexia is reversed by central administration of an MC4R antagonist (13). This finding highlights the role of POMC and melanocortins in responding to a state of positive energy balance by inducing anorexia. In contrast to the established role of MC4R, the role of melanocortin receptor 3 (MC3R) on food intake is less clear. Although MC3R-deficient mice show increased fat mass, administration of selective MC3R agonists appears not to alter food intake (18).
Cocaine- and Amphetamine-Regulated Transcript
CART is coexpressed by most POMC neurons in the ARC. Central intracerebroventricular administration of CART reduces food intake in rats whereas injection of CART antiserum does the opposite (19). In humans, a mutation of the CART gene that causes severe obesity has been described (20). The role of CART may vary in different brain regions, because CART injected directly into the ARC actually leads to an increase in food intake in fasted rats (21).
Hypothalamic Releasing Hormones
Corticotropin-releasing hormone and thyrotropin-releasing hormone (TRH) are expressed in PVN neurons. When these two hormones are administered centrally in rats, both inhibit food intake (22). TRH expression in the PVN is mediated by α-MSH and inhibited by NPY and AgRP (23), a finding consistent with the action of these peptides on food intake.
Orexins
Orexin A and B (OXA and OXB) activate G-protein-coupled receptors to increase food intake. OXA is more potent than OXB and is expressed in neurons of the DMN, PFA, and LHA, with additional projections to the NTS in the brainstem (24). In rats, central administration of an orexin antagonist inhibits feeding (25).
Melanin-Concentrating Hormone
Melanin-concentrating hormone (MCH) is an orexigenic signal expressed in neurons located in the LHA. Infusion of MCH in rats increases food intake and body weight (26). MCH knockout mice are resistant to diet-induced obesity (27). Two MCH receptors have been identified in humans whereas only one has been identified so far in rodents. MCH receptor knockout mice are resistant to diet-induced obesity (28).
Brain-Derived Neurotrophic Factor
Brain-derived neurotrophic factor (BDNF) is highly expressed in the VMN and acts via MC4R signaling to reduce food intake (29). Administration of BDNF into the lateral ventricles reduces food intake and body weight in rodents (30). Consistent with the foregoing, selective deletion of BDNF in the VMN and DMN of mice results in hyperphagia and obesity (31). ARC POMC neurons are thought to project to VMN BDNF neurons, thus activating them to lead to a reduction in food intake (29).
Ciliary Neurotrophic Factor
Ciliary neurotrophic factor (CNTF) is a cytokine expressed in several motor neuron populations. It induces an anorexigenic effect and weight loss, probably by inhibiting the expression and release of NPY in the hypothalamus (32). CNTF-mediated weight loss persists even after the cessation of treatment (33), a finding implying that it may alter the “set-point” of energy balance by inducing long-term changes in synaptic function.
The major hypothalamic nuclei and peptides implicated in the central control of food intake are shown in Figure 44.2.
Central Neurotransmitters Controlling Appetite and Food Intake
Neurotransmitters such as serotonin, norepinephrine, and dopamine act on central circuits to modulate appetite and food intake. Serotonin, produced in the dorsal raphe nucleus, reduces food intake and body weight (13). Norepinephrine, produced in the DVC and locus coeruleus, has differing effects on food intake depending on which of its receptors is stimulated; the action of norepinephrine on α2 receptors stimulates food intake, whereas its action on α1, β2, and β3 receptors reduces food intake (34).
In view of the foregoing findings, serotonin agonists (e.g., fenfluramine and dexfenfluramine) and serotonin and norepinephrine reuptake inhibitors (e.g., sibutramine) have been used as antiobesity agents. Although effective in reducing body weight, fenfluramine and dexfenfluramine have been withdrawn from the market because of serious cardiovascular side effects. Similarly, sibutramine, which was a treatment for obesity for several years, has been withdrawn because of serious cardiovascular side effects.
Dopamine appears to inhibit food intake in the ARC and LHA, whereas it has orexigenic action in the VMN (34). In addition to differing effects in different brain sites, dopamine exerts opposite effects on appetite, depending on which dopaminergic receptor subtype is stimulated. For example, the action of dopamine on D1 and D2 receptors reduces food intake, whereas stimulation of the D5 receptor is associated with reward pathways (35).
Hedonic Mechanisms and Corticolimbic Pathways Controlling Appetite and Food Intake
The word “hedonic” relates to pleasant (or unpleasant) sensations. It is apparent that visual, smell, and taste signals can override satiety signals to maintain food intake despite neutral or even positive energy balance. For example, the sweet taste of certain foods is associated with positive emotions that motivate animals to find food and continue consumption. These sensory signals are conveyed from visual, smell, and taste receptors to the NTS in the brainstem and are then relayed to corticolimbic reward centers implicated in appetite regulation. These sites include the hippocampus, amygdala, nucleus accumbens, ventral pallidum, ventral
tegmental area, and prefrontal cortex. Dopamine, serotonin, opioids, and norepinephrine have been implicated as important neurotransmitters involved in signaling within this network. The administration of an opioid µ-receptor agonist into the nucleus accumbens in fed rats elicits preferential intake of high-fat foods rather than carbohydrates (36).
tegmental area, and prefrontal cortex. Dopamine, serotonin, opioids, and norepinephrine have been implicated as important neurotransmitters involved in signaling within this network. The administration of an opioid µ-receptor agonist into the nucleus accumbens in fed rats elicits preferential intake of high-fat foods rather than carbohydrates (36).
Communication between reward centers and the hypothalamus (thought of as the main homeostatic controller of food intake, as discussed previously) culminates in overall coordination of food intake. Administration of an opioid µ-receptor agonist into the nucleus accumbens increases orexin neuron expression in the hypothalamus (37). Histochemical studies have also demonstrated connections between the cerebral cortex and MCH and orexin neurons in the LHA (38). Furthermore, in rats trained to associate the availability of food with the presentation of a food cup (resulting in conditioned food intake even when they are satiated), elimination of amygdala-hypothalamus connections completely abolishes this conditioned food intake (39). The preceding experiments highlight the importance of connections between homeostatic and nonhomeostatic centers controlling food intake.
Mnemonic Representations of Experience with Food
Past experience with specific foods forms an important contributor to continued consumption (if the previous experience was pleasant) or early cessation of intake (if the previous experience was unpleasant) regardless of satiety and energy balance. This phenomenon is referred to as conditioned preference or conditioned aversion, respectively. Evidence points to an important role for the orbitofrontal cortex (OFC), an area that receives converging sensory input in the nonhomeostatic control of food intake. The OFC is in contact with other cortical reward areas, such as the prefrontal, insular, perirhinal, entorhinal, and anterior cingulated cortices. Furthermore, the OFC communicates with the hippocampus and amygdala. Collectively, this group of brain regions is thought to be important in the generation and maintenance of a working memory for food experiences (40).
Endocannabinoids
Endocannabinoids have been shown to produce a dosedependent orexigenic effect (41). This effect is thought to occur via modulation of reward circuitry. The two main endocannabinoids in the brain are anandamide (AEA), derived from membranous phospholipids, and 2-arachidonoylglycerol (2-AG), derived from triglycerides. These substances are secreted by postsynaptic neurons and act in retrograde fashion, binding to cannabinoid receptor type 1 (CB1) receptors on presynaptic nerve terminals to inhibit the release of neurotransmitters.
CB1 receptors are colocalized with dopamine D1 and D2 receptors in the rat limbic forebrain; furthermore, dopamine receptor antagonists reduce the orexigenic effect of cannabinoid administration (42).
Endocannabinoids may also act directly on the hypothalamus to exert their orexigenic effect. In rodents, hypothalamic levels of 2-AG increase during fasting and then return to baseline after the animals are fed (43). Administration of AEA into the hypothalamic VMN results in hyperphagia that is reversible with administration of a CB1 receptor antagonist (44). Orexigenic neurons in the LHA express functional CB1 receptors (45), and stimulation of these receptors augments the OXA pathway (46) in addition to orexigenic MCH neurons (47) and NPY neurons (48).
Manipulation of the endocannabinoid system formed a therapeutic strategy in the treatment of obesity, namely, by using the CB1 receptor antagonist rimonabant. However, because of unacceptable and sometimes dangerous effects on mood, rimonabant was withdrawn from the market. The communications among peripheral inputs, the brainstem, hypothalamus, and higher brain centers in the control of appetite is shown in Figure 44.3.
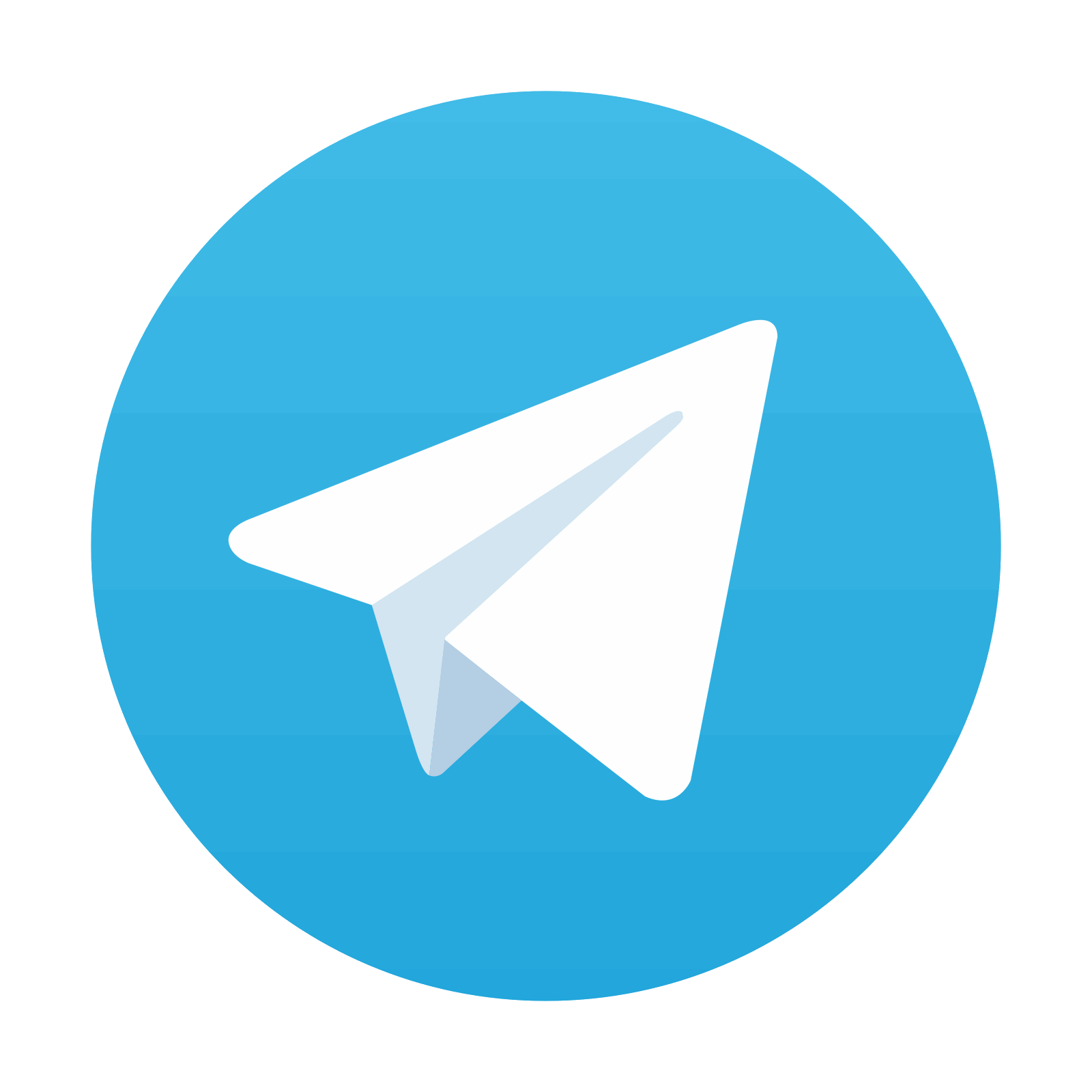
Stay updated, free articles. Join our Telegram channel

Full access? Get Clinical Tree
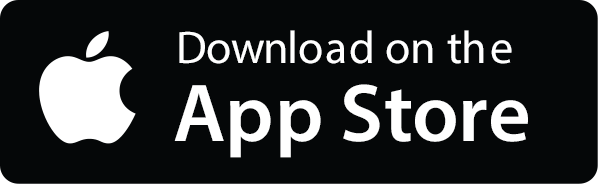
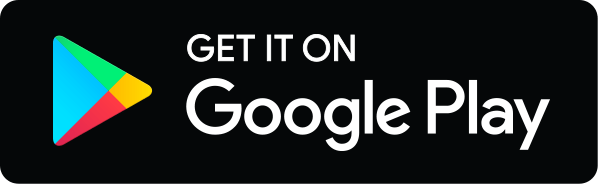