Commercial and Regulatory Development Considerations for Nanomedicines Donna Cabral-Lilly and Lawrence D. Mayer |
CONTENTS
21.2 Regulatory Guidance and Reflection Papers
21.3 Preclinical Safety Studies
21.4 Chemistry Manufacturing and Controls
21.4.2 Nanomedicine Drug Product
21.4.2.1 Characterization of Nanomedicine Drug Product: Chemical/Content Specifications
21.4.2.2 Physicochemical Characterization of Nanomedicines
21.4.2.4 Changes to Manufacturing
21.4.2.5 In Vivo Characterization of Nanomedicine Performance
21.5 Timing for Development Activities
Preclinical proof-of-concept studies typically provide the scientific rationale for further development of a drug candidate in a clinical setting and are usually conducted as basic research using good scientific practices with the goal of publication and enhancing basic knowledge in the field of study. Translating this promising research into a full drug pharmaceutical/clinical development program requires activities aimed at determining the safety of the drug product and its components, the efficacy, and most relevant to this review, the ability to relate these biological effects to specific chemical and physical attributes of the final product (Zamboni et al. 2012). These pharmaceutical/clinical development studies are based on the laws governing human and veterinary medicines, as well as the expectations of the regulatory agencies responsible for marketing approval.
For the most part, regulatory requirements for a drug delivery system–based nanomedicine are the same as those for a conventional drug of the same class. There are, however, significant additional required studies for this class of pharmaceuticals; some are general and applicable to different types of delivery systems, while others address a specific type such as for topicals or pulmonary delivery platforms.
This chapter discusses the regulatory development pathway for injectable human medicines that use a nanotechnology carrier, such as for liposomal/nanoparticle products and colloidal iron/gold conjugate products. For the purposes of this review, such nanomedicines are defined as drug-containing macromolecular particulate assemblies in the size range of 20 nm to <10 μm, with the majority of discussion topics being most relevant to intravenously administrated products with a size range of 20–200 nm. Emphasis will be placed on considerations for chemistry, manufacturing, and controls (CMC) as well as aspects of pharmacology/toxicology requirements. A general timeline, from the Investigational New Drug (IND)/Clinical Trial Application (CTA) enabling studies, through marketing application submission, is also provided.
21.2 REGULATORY GUIDANCE AND REFLECTION PAPERS
In the United States, the development and marketing of drug products is regulated by the Food and Drug Administration (FDA) whose authority is established through the Code of Federal Regulations (CFR). The sections addressing drugs for human use can be found in 21 CFR, parts 1–100, 200s, and 300s. The Center for Drug Evaluation and Research of FDA provides a comprehensive library of guidance documents on its website (www.fda.gov/Drugs) that provide procedural and technical instructions for each stage of the product development cycle. In this context, a guidance exists specific for liposomal drug products (FDA 2015), in which many of the key elements are also applicable to other “particulate” nanomedicines such as micelles, nanoparticles, dendrimers, and colloidal particles. In 2007, FDA published a report of the findings of a Nanotechnology Task Force (FDA 2007) that highlighted the need to understand the physical and chemical properties of the delivery system and its components, which will require development of new analytical methods specific for each drug product. In 2012, FDA commissioner Margaret Hamburg summarized FDA approach to regulating nanotechnology products including nanomedicines (Hamburg 2012).
Similarly, in Europe, the European Medicines Agency (EMA) held a series of meetings on the use of nanotechnologies in medicine and published a general reflection paper in 2006 (EMEA 2006). The overall considerations mirror those of the FDA, where characterization of the drug product and justification of the appropriateness of the analytical methods used are expected. EMA has also published reflection papers on four types of intravenously administered nano-based carriers including liposomes (EMA 2013b), block copolymer micelles (EMA 2013a), surface coatings (e.g., PEGylation) (EMA 2013c), and iron-based nanocolloidal products (EMA 2015).
All of the basic product characterization regulatory criteria for conventional aqueous-based injectable pharmaceutical products (e.g., content, purity/related substances, pH, sterility, endotoxin content, particulate content, and sterility) apply to nanomedicines. However, given the macromolecular particulate assembly features of nanomedicines, significant attention must also be paid to the physical disposition of the administered drug in the milieu of the particle excipients, as well as how these physical attributes affect drug release from the carrier (bioavailability), in addition to the nascent biodistribution/PK properties of the carrier itself. These attributes provide an added level of biophysical characterization that must be considered not only for regulatory (CMC) purposes but also to ensure that drug product providing reliable exposure, efficacy, and toxicology profiles can be reproducibly manufactured in a commercial setting.
Each nanomedicine drug product will be reviewed by the regulatory agencies on a case-by-case basis, since even within the nanomedicine category of injectable drugs, different classes of drug delivery carriers exhibit unique features that must be evaluated. For example, the bioavailability and plasma half-life of paclitaxel bound to albumin particles may be very different from a taxane derivative covalently linked to PEGylated-copolymer micelles. Even within a specific class of carrier, different drug products may have unique features; for example, a liposomal product may have the active agent completely encapsulated inside the internal aqueous trapped volume (e.g., Doxil®/Caelyx®), or the active agent may be imbedded in the phospholipid membrane (e.g., Ambisome®). Each type of nanomedicine will have similar types of content and impurity quality control (QC) specifications, but each will also have a very distinct “fingerprint” of biophysical attributes such as particle size distribution and morphology, surface charge, aqueous/matrix partitioning, plasma/serum particle stability, and drug release kinetics, since these features will ultimately dictate the efficacy/safety profile in vivo.
Given the diverse physical and pharmacological properties that can be designed into nanomedicines, it is recommended that developers of such products seek regulatory advice early in the development process to gain agreement on required data and to ensure that gaps do not exist that may lead to clinical hold, or long delays, in development. Mechanisms for obtaining guidance from FDA include pre-IND meetings and guidance meetings of type A (stalled development), type B (pre-IND, end of Phase 2, pre-NDA), or type C (other general guidance), among others. Such meetings can be requested for single or multiple topics where the agency will respond to questions posed and may also remind sponsors of requirements not addressed in the questions. For EMA, advice may be obtained from the Scientific Advice Working Party and/or the Innovation Task Force. More recently, FDA and EMA have begun a process where a sponsor can obtain parallel advice from the agencies. This will save time, but does not guarantee that the agencies agree on the responses to all of the questions.
21.3 PRECLINICAL SAFETY STUDIES
As with any new drug, once a candidate formulation is established that can be produced under pharmaceutically acceptable conditions, toxicology studies are required to determine if any overt safety problems exist, as well as to establish basic pharmacokinetics and a safe starting dose before first-in-man studies may begin. Normally, these studies are done in two animal species with at least one being a non-rodent mammal, and several guidelines are available to aid in study design, based on disease indication and route of administration. If an active agent is a new molecular entity (NME), additional in vitro studies to determine carcinogenicity and genotoxicity will likely also be required. For these studies, final drug product supplies should be prepared using equipment and processes that will be utilized for production of Phase 1 clinical supplies and ideally, at the same Good Manufacturing Practices (GMP) manufacturer.
All such studies must be conducted according to Good Laboratory Practices (GLPs) (see 21CFR part 58). GLP requirements include that the personnel conducting the study are qualified by education and training, facilities are adequate and maintained, equipment is qualified and calibrated, and testing facilities and controls are adequate.
Written procedures must be in place, followed, and documented to assure that the laboratory is compliant with GLP standards. The study itself must be conducted according to a preapproved protocol that describes the objective, experimental design (e.g., number of animals, dose levels, and number of doses), samples to be collected, methods of analysis, and how results will be reported. Deviations from the approved protocol must be documented and approved, and a final report is written and approved. Analytical methods that measure drug content in plasma, urine, feces, and tissues must be validated per applicable guidelines (see FDA and International Council for Harmonisation of Technical Requirements for Pharmaceuticals for Human Use [ICH] guidelines on bioanalytical testing). Complete, accurate documentation is required, and records and samples are retained to at least the extent required in the regulations. A quality assurance unit reviews the study documents and certifies compliance with GLP standards. Full reports and summaries are submitted to FDA with the initial IND application, while only the summaries are submitted in the CTA in Canada and European countries.
For intravenous (i.v.) nanomedicine drug products, a delivery vehicle control arm is typically included in the preclinical animal studies to demonstrate the safety of the carrier itself. This is particularly important if the delivery vehicle is composed of novel excipients or exhibits physical structures that have not been tested previously in man. In such cases, more extensive toxicity studies may be warranted where chronic dosing for cumulative and/or reproductive toxicities are evaluated, as well as immunogenic toxicity effects. Dose-escalation (low, medium, high) toxicity assessments using single administration help to determine the dose to use for subsequent multiple-dose studies. Data obtained include observations of animal condition, weight, blood chemistries, plasma levels of active agent and known metabolites over time, immune reactions, tissue distribution, and full necropsy. The starting dose for the first-in-man trial (Phase 0 or Phase 1) is typically determined from the highest dose level that shows no adverse events in the most sensitive animal species.
Drug delivery carriers can often contain excipients for which no information on the safety of the compounds exists. It is likely that, at a minimum, separate carcinogenicity and genotoxicity studies will be required for each novel excipient. Drug carriers that are nonbiodegradable, as the intact carrier or components of a carrier, are of particular concern since they may remain in the body for long periods of time with the potential for adverse cumulative effects. Multiple-dose studies may be required, designed to show rates and sites of accumulation, as well as studies addressing immunogenicity, cumulative toxicity, dose dependence of toxicity, dose dependence of immunogenicity, and routes of elimination. Excipients never before administered to man may need long-term data in a suitable animal model, before human clinical studies can begin.
21.4 CHEMISTRY MANUFACTURING AND CONTROLS
The last 10–15 years have seen significant advances in technologies used to manufacture and test drug delivery–based nanomedicine products. Manufacturing equipment is now controlled by microprocessors capable of balancing temperature, pressure, shear forces, and rates of addition. Rapid mixing of multiple incoming streams of components can be achieved using readily available mixers with little or no modification. Most are also capable of clean-in-place and steam-in-place, thereby greatly reducing the risk of contamination from previous batches, other products, and microbes. On the testing side, liquid chromatography has progressed where separations of complex mixtures can be achieved in minutes and yield precise and accurate quantitation. In-line, real-time methods for characteristics such as pH and particle size are in development that will allow for more continuous processes. These advances have allowed the formulation and production of very complex drug carrier systems. Regulatory agencies are working diligently to keep up with advances as demonstrated by the nanotechnology initiatives for both FDA and EMA. Each agency relies on the innovator/sponsor to understand, explain, and justify every aspect of the new drug product and manufacturing process, and each product is evaluated individually with a high level of stringency. The required CMC information is separated into two broad categories: (1) drug substance and (2) nanomedicine drug product, which are described next.
The regulatory requirements for the active pharmaceutical ingredient (API) in a medicine are the same whether the API is a totally novel compound (NME), a derivative of an existing API, or an unmodified existing API. Who is responsible for providing this information is dependent on which category applies to the API.
An active agent is considered an NME if it has never been approved by a regulatory agency. The sponsor is required to supply all information on the compound, including details on the synthesis, isolation, purification, raw materials, in-process controls, final product testing, specifications, and stability during storage. Full characterization of the molecule and its synthesis/purification is required. For example, for a small molecule, NMR, FTIR, mass spectrometry, and other such methods are used to elucidate the chemical structure. In the case of biological (protein) products, the amino acid sequence, glycosylation, and other modifications must be determined and secondary and tertiary structures provided as they are known. Beyond this characterization, each lot of API must be tested per preapproved specifications that list the attribute and its acceptance criteria. It is expected that the acceptance criteria will be wide at the beginning of development (e.g., at Phase 1) but will be refined as more experience with the manufacturing and thus data become available, with final acceptance criteria set when the marketing application is filed. Specifications should always include the following attributes: description (color, physical form), identification (often one of the characterization tests such as IR), assay, and impurities (inorganic, organic, solvents, and metal contaminates).
Physicochemical properties (e.g., melting point, refractive index), particle size, and polymorphic form should be included as appropriate. Manufacturing must be done in compliance with current GMP expectations, and the quality assurance unit must confirm, in writing, compliance with these standards. This confirmation, called a Certificate of Compliance or Certificate of Conformance, is in addition to full testing per preapproved specifications and acceptance criteria where results are tabulated in a Certificate of Analysis.
When using a drug delivery carrier, it is often necessary to modify a known drug substance in order to stably incorporate it into the carrier in the final drug product. Prodrugs are such a case where, for example, a water-soluble active agent is attached to a long-chain fatty acid through a labile linker, to make the API more hydrophobic. The prodrug would then reside in a hydrophobic core of a micellar-type nanoparticle. Alternatively, the active agent might be linked to a long-chain polymer that covers the outside of the nanoparticle. From a regulatory perspective, the prodrug is considered an NME and is subject to all of the same controls as described for a novel compound.
The active agent, however, may be purchased commercially, and information on the synthesis and stability of this now starting material may be obtained from the commercial manufacturer, who preferably will have a drug master file (DMF) established with the regulatory agencies. Characterization of the prodrug should include data to support a proposed pathway to generate the active agent. Questions to answer include as follows: What bond gets hydrolyzed first? Does the linker stay with the “pro” portion? Under what conditions does the hydrolysis take place (e.g., low pH, or is an enzyme needed)? What is the known safety profile of the “pro” portion, linker, and hydrolysis intermediates? The answers to these questions will determine what additional safety studies are required.
The most straightforward regulatory path is for a commercially available drug substance that is already approved for use in a generic drug product. A novel delivery system is usually designed in this case to improve safety or enhance efficacy, and the API can be incorporated without modification. The information on manufacturing scheme, controls, characterization, and validation may be provided in a DMF submitted by the vendor and referenced, with permission, by the drug product sponsor. The sponsor is still ultimately responsible for the quality of the API and is expected to have quality agreements in place, and conduct routine audits of the manufacturer, but does not have to duplicate the information in the DMF.
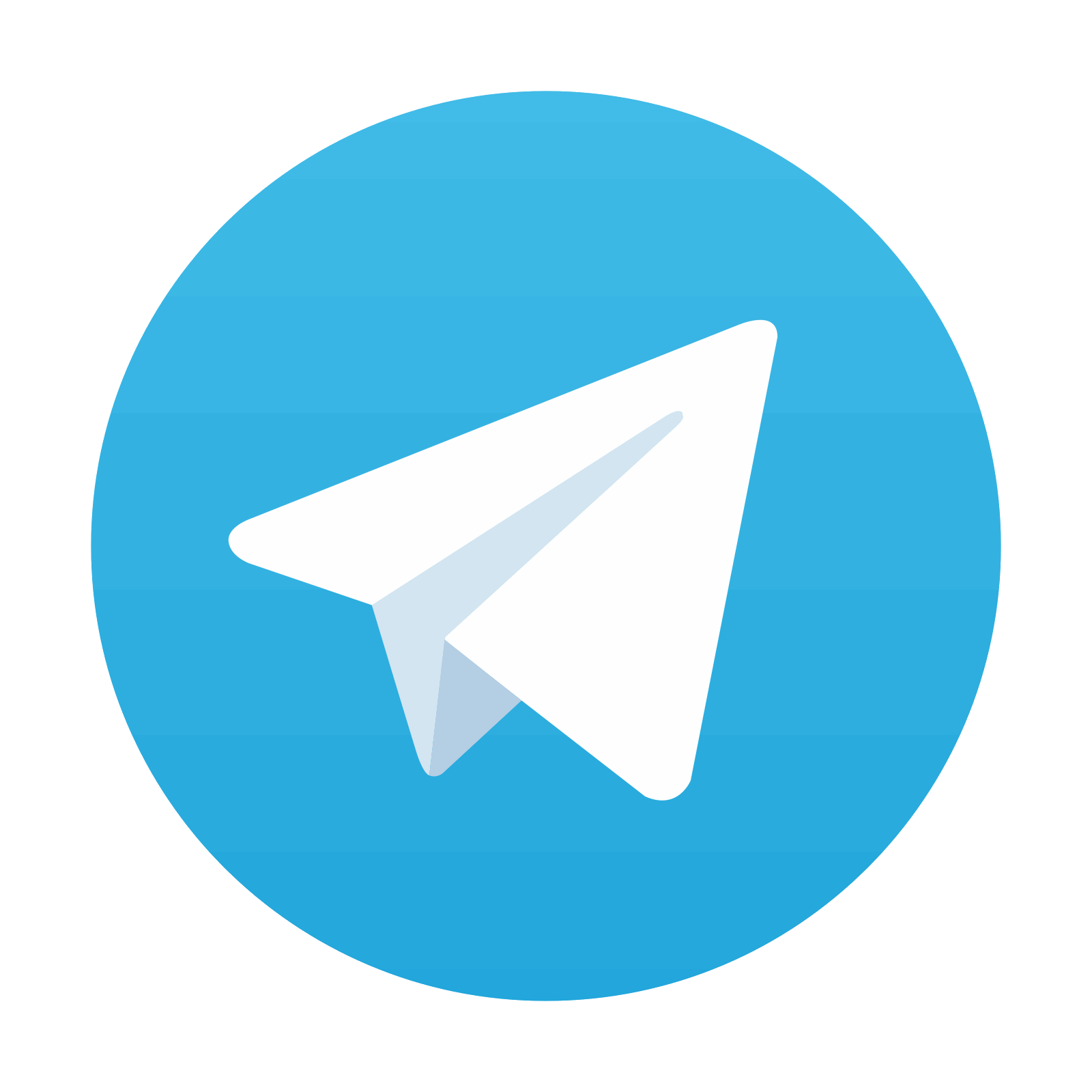
Stay updated, free articles. Join our Telegram channel

Full access? Get Clinical Tree
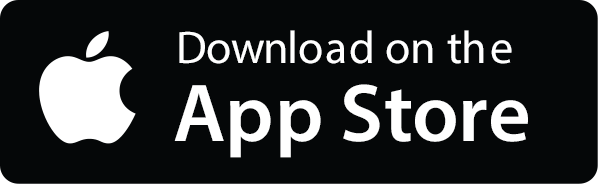
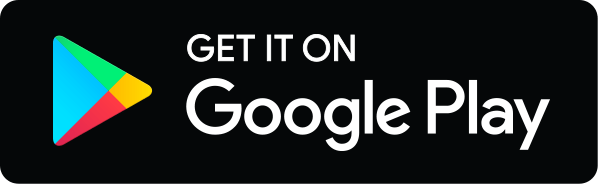