Chapter 12 Clinical reasoning and biomedical knowledge
implications for teaching
Health science curricula worldwide are undergoing significant structural changes that are likely to shape the practice of the health sciences for decades to come. The role of biomedical knowledge in clinical medicine is one of the focal issues in this transformation. Basic science knowledge reflects a subset of biomedical knowledge, although the two terms are often used interchangeably. There are many competing views and assumptions concerning the role of biomedical knowledge and its proper place in a health science curriculum. In this chapter we consider some of these arguments in the context of empirical evidence from cognitive studies in medicine. The role of basic science knowledge is a subject of considerable debate in medical education. It is generally accepted that basic science or biomedical knowledge provides a foundation upon which clinical knowledge can be built. However, its precise role in medical reasoning is controversial (Norman 2000). Biomedical knowledge has undergone a dramatic transformation over the past 30 years, presenting unique and formidable challenges to medical education (Association of American Medical Colleges (AAMC) 2004). There is considerable uncertainty concerning the relationship between basic science conceptual knowledge and the clinical practice of physicians (e.g. Woods et al 2005). There continues to be a dramatic increase in the volume of medical knowledge, especially in cellular and molecular biology (Shaywitz et al 2000). In the past, medical schools have typically responded by adding the new content to existing courses, increasing the number of lectures and textbook readings (D’eon & Crawford 2005). This has changed somewhat as clinical courses have become more routine in the first two years of medical school (AAMC 2004). In addition, basic science courses are increasingly competing with new curricular demands and objectives, for example to improve professionalism and patient-centred care (AAMC 2006).
THE FUTURE ROLE OF BASIC SCIENCE KNOWLEDGE
There have been increasing expressions of dissatisfaction with basic science teaching in medicine. It has been argued that substantial parts of the basic science in medical schools are irrelevant to the future needs of practitioners (Neame 1984). Furthermore, the method of presenting information in a didactic lecture format and with text readings that do not usually include clinical reasoning exercises encourages passivity and rote learning, which inhibits the development of understanding (Patel et al 2004). This has been increasingly recognized by medical educators, and medical schools have taken steps to make basic science teaching more clinically relevant (Benbassat et al 2005).
In the past 20 years, information technology has had a profound effect on the practice of medicine (Shortliffe & Blois 2006). However, the ways in which these changes should affect clinical training is the subject of ongoing debate in medical informatics (Patel & Kaufman 2006). Information technology can provide access to a wealth of information and has the potential to improve patient care substantially. Serious concerns have been raised about whether future health science practitioners will continue to require the kinds of scientific training that their predecessors received. According to Prokop (1992), there are clear historical trends that are likely to continue. New discoveries in science will continue to provide physicians with increasingly powerful investigative tools with which to see the workings of the human body and through which to prevent disease. When we consider the historical precedents, it seems likely that the best clinical judgement will require a broader understanding of both biology and medicine than ever before (Prokop 1992). A relatively recent report by the AAMC (2001, p. 5) proposed:
It is likely that advances in genomics, proteomics (defined as ‘the study of the set of proteins produced (expressed) by an organism, tissue or cell, and the changes in protein expression patterns in different environments and conditions’; University of Indiana 2007) and bioinformatics will influence clinical medicine in the near future and it will therefore need to be incorporated into medical curricula. In addition, an increased risk for infectious diseases such as SARS and bird flu, as well as the potential dangers of agents of bio-terrorism are new risks that physicians must be prepared to grapple with (Debas 2000, Fauci 2005). Given that treatment guidelines are unlikely to cover the spectrum of emerging illnesses, it may be necessary for clinicians to have a deeper understanding of dangerous pathogens and how they may affect human disease.
CURRICULAR AND EPISTEMOLOGICAL ISSUES
Clinical knowledge includes knowledge of disease entities and associated findings, and basic science knowledge incorporates subject matter such as biochemistry, anatomy and physiology. Basic science or biomedical knowledge provides a scientific foundation for clinical reasoning. It had been widely accepted that biomedical and clinical knowledge can be seamlessly integrated into a coherent knowledge structure that supports all cognitive aspects of medical practice, such as diagnostic and therapeutic reasoning (Feinstein 1973). From this perspective, clinical and biomedical knowledge become intricately intertwined, providing medical practice with a sound scientific basis. Since the Flexner report (1910), medical schools have made a strong commitment to this epistemological framework. The report recommended the partitioning of the medical curriculum into a basic science component and an applied component. Medical educators and researchers have argued over how to best promote clinical skill as well as foster robust conceptual change (Boshuizen & Schmidt 1992, Clough et al 2004, Patel & Groen 1986).
Traditionally, the curricula of most medical schools during the first and second years involve preclinical courses which predominantly teach the basic sciences. The remaining two years of medical school and further postgraduate training consist of clinical courses and practica. This has begun to change in recent years, in part as a result of the growing popularity of problem-based learning (PBL). In PBL programmes, instruction involving clinically meaningful problems is introduced at the beginning of the curriculum. This practice is guided by the assumption that scientific knowledge taught abstractly does not help students to integrate it with clinical practice (Norman & Schmidt 2000). Recently, conventional or traditional clinical schools have embraced the idea of emphasizing a more clinically relevant basic science curriculum. Following PBL, they have also incorporated small group teaching and have focused more on fostering clinical skills. The renewed emphasis on skills and competency has been partly in response to reports indicating that patient care is sub-optimal. In particular, reports by the Institute of Medicine (e.g. 2001) characterized a state of affairs in which medical errors have caused an alarming number of deaths in the USA and the quality of care has been found to be deficient in significant respects. Studies have also indicated that physicians are not very effective in communicating with patients (Debas 2000) or in conducting physical examinations (Benbassat et al 2005), deficiencies which are likely to contribute to the problems associated with quality of care.
Medical problem solving can be characterized as ill-structured, in the sense that the initial states, the definite goal state and the necessary constraints are unknown at the beginning of the problem-solving process. In a diagnostic situation, the problem space of potential findings and associated diagnoses is enormous. The problem space becomes defined through the imposition of a set of plausible constraints that facilitate the application of specific decision strategies (Pople 1982). For example, when faced with a multi-system problem such as hypokalemic periodic paralysis associated with hyperthyroidism, a physician may need to confirm the more common disorder of hyperthyroidism before solving the more vexing problem of hypokalemia. Once this is confirmed, there is a set of constraints in place such that there are classes of disorders that co-occur with hyperthyroidism and there is a set of symptoms that have not yet been accounted for by this disorder and are consistent with hypokalemic periodic paralysis. As expertise develops, the disease knowledge of a clinician becomes more dependent on clinical experience, and clinical problem solving is increasingly guided by the use of exemplars, becoming less dependent on a functional understanding of the system in question. Biomedical knowledge, by comparison, is of a qualitatively different nature, embodying elements of causal mechanisms and characterizing patterns of perturbation in function and structure.
The focus of the instructional approach for the biomedical curriculum is necessarily on the extensive coverage of a broad corpus of knowledge as opposed to in-depth conceptual understanding. The volume of information in any one of the basic science disciplines is now so large that it cannot be completely mastered even by a full-time graduate student pursuing doctoral studies for 5 years (Prokop 1992). It is unreasonable to expect that medical students can master five or more fields in the first 24 months of medical school. In our view it is not tenable, given a finite time frame and finite psychological resources, to coordinate these multiple sources of knowledge and harmonize all biomedical knowledge with a clinical body of knowledge of disease entities and associated findings.
Feltovich and colleagues (1993) proposed that medicine can be construed as a domain of advanced knowledge acquisition. These domains necessitate learning that takes place beyond the initial or introductory stages. For example, medical students are expected to have a substantial background in the biological sciences. Much of the basic science subject matter in medical schools is predicated on the fact that students have a basic mastery of the introductory materials, so that instructors can focus on more advanced topics. The goal of introductory learning is to provide exposure to large areas of content with the goal of providing a basic literacy or familiarity with the domain. There is not much emphasis on conceptual mastery of knowledge. Advanced knowledge acquisition carries the expectation of students attaining a deeper understanding of the content material and the ability to use it flexibly and productively in diverse contexts. Although we view many of the curricular changes as a substantive improvement, there are lingering questions as to the effect on mastery of basic science knowledge.
RESEARCH IN CLINICAL REASONING
CLINICAL REASONING STRATEGIES AND EXPERTISE
Lesgold et al (1988) investigated the abilities of radiologists at different levels of training and expertise to interpret chest X-ray pictures and provide a diagnosis. Experts were able to initially detect a general pattern of disease with a gross anatomical localization, serving to constrain the possible interpretations. Novices had greater difficulty focusing on the important structures, being more likely to maintain inappropriate interpretations despite discrepant findings in the patient history. The authors concluded that the knowledge that underlies expertise in radiology includes the mental representation of anatomy, a theory of anatomical perturbation, and the constructive capacity to transform the visual image into a three-dimensional representation. The less expert subjects had greater difficulty in building and maintaining a rich anatomical representation of the patient.
Norman et al (1989) compared dermatologists’ performance at various levels of expertise in tasks that required them to diagnose and sort dermatological slides according to the type of skin lesion evident. Expert dermatologists were more accurate in their diagnoses and took significantly less time to respond than novices. The sorting task revealed that each group sorted the slides according to different category types. Experts grouped the slides into superordinate categories such as viral infections, which reflected the underlying pathophysiological structure. Novices tended to classify lesions according to surface features such as scaly lesions. The implication is that expert knowledge is organized around domain principles which facilitate the rapid recognition of significant problem features. It supports the idea that experts employ a qualitatively different kind of knowledge to solve problems based on a deeper understanding of domain principles.
The picture that emerges from research on expertise across domains is that experts use a quite different pattern of reasoning from that used by novices or intermediates, and organize their knowledge differently. Three important aspects are that experts: (a) have a greater ability to organize information into semantically meaningful, interrelated chunks; (b) do not process irrelevant information; and (c) in routine situations, tend to use highly specific knowledge-based problem-solving strategies (Ericsson & Smith 1991). The use of knowledge-based strategies has given rise to an important distinction between a data-driven strategy (forward reasoning) in which hypotheses are generated from data, and a hypothesis-driven strategy (backward reasoning) in which one reasons backward from a hypothesis and attempts to find data that elucidate it. Forward reasoning is based on domain knowledge and is thus highly error-prone in the absence of adequate domain knowledge. Backward reasoning is slower and may make heavy demands on working memory (because one has to keep track of goals and hypotheses), and is most likely to be used when domain knowledge is inadequate. Backward reasoning is characteristic of non-experts and experts solving non-routine problems (Patel et al 2005).
In experiments with expert physicians in cardiology, endocrinology and respiratory medicine, clinicians showed little tendency to use basic science in explaining cases, whereas medical researchers showed preference for detailed, basic scientific explanations, without developing clinical descriptions (Patel et al 1989). In medicine, the pathophysiological explanation task has been used to examine clinical reasoning (Feltovich & Barrows 1984). This task requires subjects to explain the causal pattern underlying a set of clinical symptoms. Protocols from this task can be used to investigate the ability of clinicians to apply basic science concepts in diagnosing a clinical problem. In one study (Patel & Groen 1986), expert practitioners (cardiologists) were asked to solve problems within their domain of expertise. Their explanations of the underlying pathophysiology of the cases, whether correctly or incorrectly diagnosed, made virtually no use of basic science knowledge. In a similar study (Patel et al 1990), cardiologists and endocrinologists solved problems both within and outside their domains of expertise. The clinicians did not appeal to principles from basic biomedical science, even when they were working outside their own domain of expertise; rather, they relied on clinical associations and classifications to formulate solutions. The results suggest that basic science does not contribute directly to reasoning in clinical problem solving for experienced clinicians. However, biomedical information was used by practitioners when the task was difficult or when they were uncertain about their diagnosis. In these cases, biomedical information was used in a backward-directed manner, providing coherence to the explanation of clinical cues that could not be easily accounted for by the primary diagnostic hypothesis that was being considered.
There have been many other studies highlighting the difficulty of integrating basic and clinical knowledge (e.g. Boshuizen & Schmidt 1992, Patel et al 1993, Woods et al 2005). Pathophysiological information is used by physicians and senior medical students either when the problem-solving process breaks down (i.e. no obvious solution) or to explain loose ends (i.e. leftover findings) that cannot be accounted for by the diagnostic hypothesis(es). In general, there is evidence to suggest that unprompted use of biomedical concepts in clinical reasoning decreases as a function of expertise. In addition, students have difficulty in applying basic science concepts in contexts that differ from the initial conditions of learning (Patel et al 1993). The first three studies described in this section focus on expertise in visual diagnosis and suggest a more transparent role for basic science knowledge than does the work on expertise in the domains of cardiology and endocrinology. Although pattern recognition is an important aspect of all diagnostic expertise, certain domains necessitate a greater use of core biomedical concepts in understanding even basic problems.
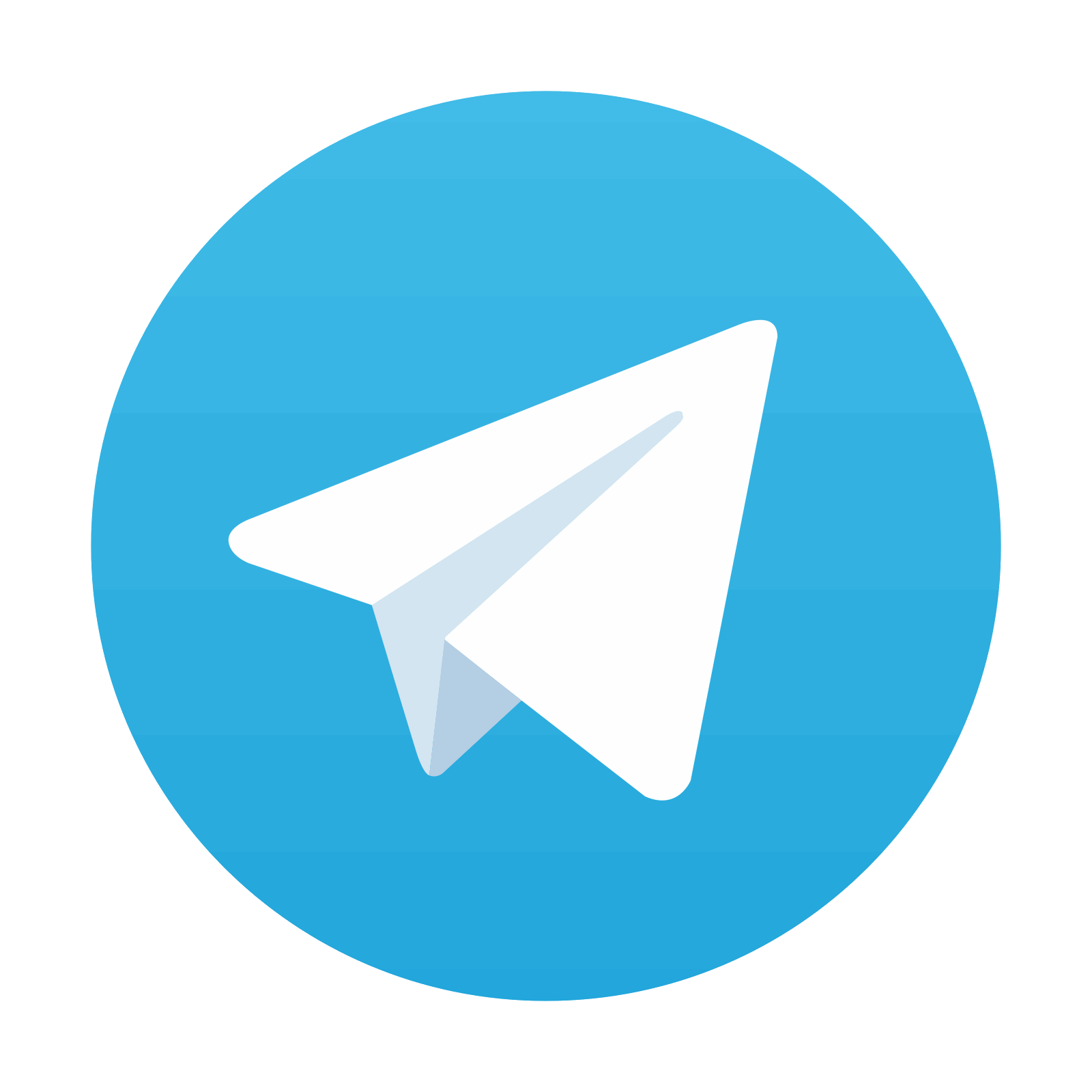
Stay updated, free articles. Join our Telegram channel

Full access? Get Clinical Tree
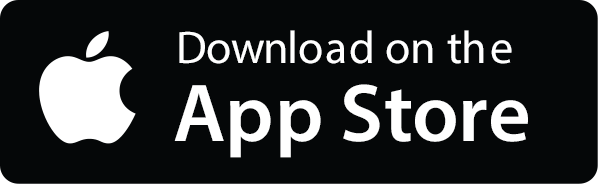
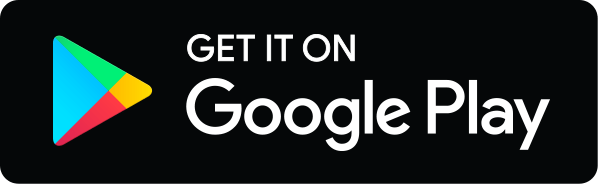