DEVELOPMENTAL CHANGES FROM NEONATE TO ADULT
Pediatric laboratory medicine provides many unique opportunities to study how the homeostatic and physiologic mechanisms that control normal human development evolve. With these opportunities to study development comes a completely new set of challenges, many based on failure of some component of the normal development process and resulting disease. With this scenario in mind, it becomes clear that the environment for the specialist pediatric laboratorian is different from the environment encountered in adult practice, in which physiologic development is not a major issue. The diseases encountered in pediatric practice, therefore, differ considerably from those in adult situations. Moreover, the nature of body size and, hence, available blood volume create additional problems for the analyst with regard to choice of instrumentation and testing menu.
The greatest pediatric challenge relates to the birth of an infant. There is a requirement at this time for rapid adaptation from intrauterine life, in which homeostasis is maintained by maternal and placental means, to the self-maintenance needed to adapt to extrauterine life. Issues related to this adaptation are further complicated by prematurity or intrauterine growth restriction (IUGR), when many organ systems have not reached sufficient maturity to enable the newborn to adapt to the necessary changes at the time of delivery.1
Respiration and Circulation
At birth, the normal infant rapidly adapts by initiating active respiration. The stimuli for this process include clamping of the umbilicus, cutting off maternal delivery of oxygen, and the baby’s first breath. Initiation of breathing requires the normal expression of surfactant in the lungs. Surfactant is necessary for the normal expansion and contraction of alveoli and allows gaseous exchange to take place.
Initiation of respiration and expansion of lung volume causes increased pulmonary blood flow and reduced blood pressure. This, in turn, results in closure of the ductus arteriosus and a shift in blood flow through the heart that allows newly oxygenated blood from the lungs to be directed through the left side of the heart to the body. Blood flow now goes from the right side of the heart to the lungs for oxygenation. Closure of the ductus arteriosus is essential for this process to take place.
Growth
A normal baby delivered at term weighs about 3.2 kg. A baby whose birth weight is below the 10th percentile for gestational age is considered small for gestational age (SGA), a condition potentially caused by IUGR. Babies are regarded as premature when born at less than 37 weeks of gestational age. In the first days of life, weight loss is a result of insensible water loss through the skin. This is generally offset by weight gain of 6 g/kg/d as feeding is initiated. An infant’s body weight will double in 4 to 6 months. Premature babies tend to grow at a slower rate and often still weigh less than does a term baby at the equivalent of term.
Organ Development
Most organs are not fully developed at birth. Glomerular filtration rate (GFR) of the kidney and renal tubular function mature during the first year of life at which point laboratory markers of renal function approximate adult values. Liver function can take 2 to 3 months to fully mature. Motor function and visual acuity develop during the first year of life. This development is accompanied by changes in the electroencephalogram until the normal “adult” picture is seen. There are dramatic changes in hematopoiesis as the switch from fetal hemoglobin to adult hemoglobin takes place. This coincides with significant hyperbilirubinemia as fetal hemoglobin is broken down, coincident with immature hepatic pathways of bilirubin metabolism. Bone growth in the rapid growth phases in the first few years of life and at puberty results in cyclical changes in bone growth markers. Sexual maturation results in significant endocrine changes, particularly of the hypothalamic–pituitary–gonadal hormone pathway, which eventually lead to the constitutive development of adult secondary sexual characteristics and eventually to the adult.
Problems of Prematurity and Immaturity
Intrauterine development is programmed for a normal 38 to 40 weeks of gestation.1 Many organs are not fully ready to deal with extrauterine life before this time. This organ immaturity results in many of the clinical problems that we see associated with prematurity, which include respiratory distress (lung immaturity), electrolyte and water imbalance (kidney immaturity), and excessive jaundice (liver immaturity). Infants born before their due date constitute a major burden on the laboratory. They not only have abnormal biochemical parameters that require frequent blood drawing, they also have small blood volumes from which to draw on.
PHLEBOTOMY AND CHOICE OF INSTRUMENTATION FOR PEDIATRIC SAMPLES
Phlebotomy
Blood collection from infants and young children is complicated by the patient’s size and frequently by the ability of the patient to communicate with the phlebotomist. The small blood volume of small patients dictates both the number of tests that can be performed safely on the patient and the number of times that blood can be drawn safely for repeat analysis.2 Table 35.1 shows the percentage of total body blood volume that is drawn from an individual with a 10-mL blood draw. This volume is standard in adult laboratory medicine, but the table clearly shows that this amount of blood represents about 5% of total blood volume in a premature neonate. Clearly, frequent blood draws of this nature quickly will lead to anemia and the need for blood transfusion. Table 35.2 shows the guidelines for blood volume collection developed at Children’s Hospital of Philadelphia. Infants and children have smaller veins than adults; to ensure that small veins do not collapse, narrow-gauge needles are generally used for venipuncture. Smaller needles increase the risk of hemolysis and hyperkalemia.
TABLE 35.1 Implications of a 10-mL Blood Draw in an Infant Population
TABLE 35.2 Recommended Blood Draw Volumes for Pediatric Patients
Courtesy of Dr. David Friedman, Children’s Hospital of Philadelphia, Philadelphia, Pennsylvania.
Frequently, good access to veins is impossible in a pediatric patient with intravenous and central lines in place. Capillary samples often are collected when suitable veins are not available. However, capillary blood obtained by skin puncture has the potential to be contaminated, at least to some extent, by interstitial fluid and tissue debris. The concentration of protein (and protein-bound constituents) is approximately three times lower in interstitial fluid than in plasma. Table 35.3 highlights the major differences in analyte composition between venous serum and capillary serum. The lower concentrations of protein, bilirubin, and calcium in capillary specimens likely reflect mixing (and dilution) with interstitial fluid. Capillary samples, by either heel or finger stick, should be collected by phlebotomists with pediatric expertise. The heel should be warmed and well perfused to “arterialize” the capillaries, and the lancet puncture should be in an area of the heel away from bone. Excessive squeezing or milking of the lancet site can result in both hemolysis and factitious hyperkalemia from tissue fluid leakage.
TABLE 35.3 Differences in Composition of Capillary and Venous Serum
Source: Burtis CA, Ashwood ER, eds. Tietz Fundamentals of Clinical Chemistry. 5th ed. St. Louis, MO: WB Saunders; 2012.
Preanalytic Concerns
There is a growing trend toward complete front-end automation in clinical chemistry laboratories. The clear advantage with automation of sample handling is that traditional bottlenecks at sites of data entry and centrifugation are removed and turnaround times are reduced. Several issues have prevented the introduction of full-scale automation in pediatrics. A typical pediatric chemistry laboratory receives samples in tubes of many different sizes, varying from standard adult tubes to small pediatric tubes or Microtainers. While several of the large chemistry analyzers can directly sample from small pediatric tubes, as of this time, no manufacturer of laboratory equipment has developed a fully automated system that can handle this range of tubes. Although there have been reports in the literature from individual laboratories that have modified existing robotic instrumentation to allow complete automation of the testing process using pediatric tubes,3,4 this is not routine practice.
A second important issue relates to evaporation of sample from open tubes. Most automated sample handling systems require open-topped tubes for processing. With large sample volumes, the effect of evaporation is minimal. With small volumes that have relatively large surface areas to total volume, evaporation can be significant and may affect results by as much as 10%.
Choice of Analyzer
Careful inspection and choice of analytic systems remain crucial for handling pediatric samples. Until recently, only a few analyzers were capable of performing multiple analytic procedures on small sample volumes (5 to 50 μL). Today, most analyzers can perform this function. Choice of analyzer becomes dependent on issues such as the following:
1. How much dead volume is there in the system? The smaller the dead volume, the greater is the number of tests that can be run.
2. Does clot or bubble detection allow for salvage of sample? All analyzers can detect a clot, but not all will allow retrieval of sample.
3. Is the system truly random access? This allows selectivity of menu for a given sample.
Typically, sample throughput time is less of an issue, as pediatric facilities tend to run fewer samples than busy adult services.
POINT-OF-CARE ANALYSIS IN PEDIATRICS
Point-of-care testing (POCT), or near-patient testing, plays an important and expanding role in pediatric practice.5 Testing devices that are portable and easy to use, require small specimen volume, do not require sample preparation, and provide rapid results at the bedside are providing the momentum for increased POCT. To provide cost-effective and appropriate quality assurance for POCT, several factors need to be addressed.5
1. Does the analyte really require immediate turnaround for optimal patient management? Analyzers are becoming available that measure increasing numbers of different analytes at the patient’s bedside. Typically, the cost of POCT measurement is higher than the traditional laboratory measurement. The idea of instant results is so seductive that nonlaboratorian users often discount economic factors. In the author’s institution, the clinical laboratory has played a leading role in determining which POCT assays will be available and in which clinical settings they have real value (Table 35.4).
2. Who chooses the POCT device? As the field of POCT expands, the number of devices on the market is also increasing. The clinical laboratory should be the setting in which POCT devices for institutional use should be first evaluated. The laboratory should make choices for instrumentation. Important features of a good POCT device should include the following:
- The ability to lock out untrained users. Only individuals accredited to use the device should be allowed access via a personal code.
- The device should not be allowed to proceed to patient sample analysis without running and validating appropriate quality assurance procedures.
- The data should be downloadable to the hospital laboratory information system (LIS) for evaluation by the hospital quality assurance officer. Downloading the data also allows for billing and data entry into patient charts, features readily lost when analyzers are used that cannot be linked to the LIS.
TABLE 35.4 Important Pediatric Point-of-Care Tests and Testing Sites
The data generated by POCT devices have limitations. Typically, analytic performance is not as good as that with the main laboratory analyzer. POCT data are less precise and not suited to monitoring therapy in instances where small changes are important. The linear range for most POCT devices is not as broad as that of the main chemistry analyzer, and users need to be aware of these limitations. A prime example in the authors’ institution is the use of POCT glucose analyzers. The instrument used for acute diabetic management in the outpatient setting loses linearity above 400 mg/dL, while the main chemistry analyzer measures up to 600 mg/dL (and the range can be extended even higher with sample dilution). Hypoglycemia is also particularly common in pediatrics and characteristically difficult to accurately quantitate using POCT devices. Low glucose levels should be confirmed using a more sensitive main laboratory analyzer.
REGULATION OF BLOOD GASES AND PH IN NEONATES AND INFANTS
Primary maintenance of blood gas and pH homeostasis following birth requires that the lungs and kidneys are sufficiently mature to regulate acid and base metabolism. At about 24 weeks of gestation, the lung expresses two distinct types of cells: type 1 and type 2 pneumocytes. Type 2 pneumocytes are responsible for the secretion of surfactant, which contains the phospholipids lecithin and sphingomyelin. Surfactant is required for the lungs to expand and the transfer of blood gases following delivery. Oxygen crosses into the circulation, and carbon dioxide is removed and expired. Immaturity of the surfactant system as a result of prematurity or IUGR results in respiratory distress syndrome (RDS). In RDS, there is failure to excrete carbon dioxide and, as a result, carbon dioxide levels rise, causing respiratory acidosis; oxygen levels are low and result in additional oxygen requirements for the baby.
The relative amounts of lecithin and sphingomyelin are critical for normal surfactant function. The measurement of amniotic fluid lecithin/sphingomyelin (L/S ratio) has been used for many years to predict fetal lung maturity. A ratio of less than 1.5 is considered indicative of surfactant deficiency.
The fetal fibronectin (fFN) test is a newer test used to determine the likelihood of premature delivery and risk for fetal maturity in women at high risk for preterm delivery.6,7 fFN is a protein secreted uniquely by the fetus; toward term, or when preterm labor is potentially imminent, it is found in maternal cervical fluid. A POCT device is available that has been designed for use in the obstetrician’s office. A negative test has a high predictive value that preterm delivery of the baby (within 7 to 14 days following testing) is unlikely.6 A positive test is moderately predictive of preterm delivery, warrants close follow-up, and can be used to alert the pediatrician to the potential for RDS.
The trauma and relative anoxia during delivery can also induce acidosis in the newborn. This is typically a metabolic acidosis associated with increased lactic acid production. Serum bicarbonate levels are reduced in this situation compared with the respiratory acidosis in RDS. Persistent metabolic acidosis in the newborn that is difficult to correct with bicarbonate replacement is an indication for further intensive evaluation for possible inborn error of metabolism or other etiologies that require differentiation (Table 35.5).
TABLE 35.5 Causes of Acidosis and Alkalosis in Neonates and Infants
Alkalosis is an unusual finding in pediatric medicine. One important cause of alkalosis is hyperammonemia, which may be secondary to a number of etiologies, including liver disease and inborn errors of metabolism (Table 35.5).
Blood Gas and Acid–Base Measurement
Oxygen status can readily be measured using noninvasive transcutaneous monitoring. Good correlation has been demonstrated between the arterial pressure of oxygen and transcutaneous measurement. Transcutaneous carbon dioxide monitors are also in widespread use. The measurement of acid–base status requires blood sampling. Most blood gas/acid–base analyzers can be adapted to take the small capillary samples routinely collected in pediatric settings. It is important for the person drawing the capillary blood to do so anaerobically, which requires thorough warming of the capillary site and collection of a freely flowing blood sample from a lancet stick. The sample needs to be sealed to ensure minimal gas exchange. Analysis should be performed immediately to not compromise the sample integrity. The author’s laboratory maintains a goal of a 10-minute turnaround time from the sample receipt.
Most blood gas analyzers measure pO2, pCO2, and pH by ion-specific electrodes and calculate bicarbonate concentration by the Henderson-Hasselbalch equation. The Henderson-Hasselbalch equation is less valid when pH is far outside the normal physiologic range (extreme acidosis or alkalosis). On these occasions, it may become important to measure the bicarbonate concentration using a direct measurement.
Many blood gas analyzers have been upgraded in recent years to measure additional analytes, including blood sodium, potassium, and chloride, using ion-specific electrodes and lactate and urea and several instruments measure bilirubin and creatinine. The major advantage of this type of analyzer in pediatrics is that whole blood can be used. The volumes are typically smaller than those required for the main chemistry analyzer, and the lack of need for centrifugation shortens the turnaround time. One disadvantage of using whole blood is that the analyst cannot detect whether a sample is hemolyzed.
REGULATION OF ELECTROLYTES AND WATER: RENAL FUNCTION
From the 35th week of gestation, the fetal kidneys develop rapidly in preparation for extrauterine life.1 The kidneys, critical organs for the maintenance of electrolyte and water homeostasis, control the rate of salt and water loss and retention. At term, neither the glomeruli nor the renal tubules function at the normal rate. The GFR is about 25% of the rate seen in older children and does not reach full potential until age 2 years (Table 35.6). Tubular function also develops at a similar rate. The maximal concentrating power of the kidney is only about 78% of that of the adult kidney at this time, although the tubular response to antidiuretic hormone appears to be normal. This gradual process of renal development in the newborn results in diminished filtration and impaired reabsorption of salt and water; therefore, in the newborn period and early infancy, large shifts in serum electrolyte levels can be observed. These problems are exacerbated in the preterm infant with renal function that is even less mature.
TABLE 35.6 Development of Glomerular Filtration in the Newborn
aAdult values.
The kidneys also primarily maintain water loss and retention. However, in the newborn period, insensible water loss through the skin is also an important cause of water and electrolyte imbalance. Water loss and consequent hemoconcentration frequently result from the use of radiant heaters that are used to maintain body temperature. Increased water loss also occurs via respiration in children with RDS. Up to one third of insensible water loss may occur through this route. The total body water content of a newborn is about 80%; 55% is intracellular fluid, and 45% is extracellular fluid. The extracellular water is 20% plasma water and 80% interstitial. During the first month of extrauterine life, the total body water content decreases to about 60%, mostly a result of loss of the interstitial component.1
Disorders Affecting Electrolytes and Water Balance
The causes of hypernatremia (sodium, >145 mEq/L) and hyponatremia (sodium, <130 mEq/L) are listed in Table 35.7. Both disturbances can have dire outcomes, with a high risk of seizures. This is a result of the shift of water out of or into brain cells, with concurrent shrinkage or expansion of these cells. Hypernatremia results from hypotonic fluid loss, and hyponatremia results from hypertonic fluid loss. Hyponatremia may also be a result of excessive body water content and needs to be distinguished from hypertonic loss. Clinical evaluation and measurement of other components, including hematocrit, serum albumin, creatinine, and blood urea nitrogen, can be used to differentiate these etiologies. All of these compounds will be elevated with hemoconcentration. Clinically, it is usually possible to distinguish dehydration from excessive hydration.
TABLE 35.7 Causes of Hypernatremia and Hyponatremia
Treatment of electrolyte and water loss is directed at replacing the loss to regain normal physiologic levels. Care must be taken to avoid too rapid a replacement, particularly with hypertonic dehydration. If water replacement is done too quickly, a rapid expansion of neuronal cell volume can occur, which results in seizures.
The causes of hyperkalemia and hypokalemia are listed in Table 35.8. The symptoms of hyperkalemia (serum potassium, >6.5 mEq/L) include muscle weakness and cardiac conduction defects that may lead to heart failure. In pediatrics, it is particularly important to recognize factitious hyperkalemia as a result of hemolysis and bad capillary blood collection, without hemolysis but with high potassium tissue leakage.
TABLE 35.8 Causes of Hyperkalemia and Hypokalemia
Because the situation regarding electrolyte and water homeostasis can change rapidly in small infants, it is important to monitor therapeutic intervention on a frequent basis.1,2 The availability of POCT devices that use small volumes of whole blood helps with management of these imbalances, with the only caution being that it is impossible to detect hemolysis and factitious hyperkalemia on a whole blood sample.
DEVELOPMENT OF LIVER FUNCTION
Physiologic Jaundice
The liver is an essential organ for many metabolic processes. The processing of many normal metabolic pathways and the metabolism of exogenous compounds, in particular, pharmacologic agents, proceed slower in neonates. The most striking effect of an immature liver, even in a normal-term baby, is the failure to adequately metabolize bilirubin. Bilirubin is an intermediate of the breakdown of the heme molecules, which accumulate as fetal hemoglobin is rapidly destroyed and replaced by adult hemoglobin. Normally, the liver conjugates bilirubin to glucuronic acid using the enzyme bilirubin UDP-glucuronosyltransferase. Conjugated bilirubin can be readily excreted in the bile or through the kidneys. At birth, this enzyme is too immature to complete the process, and increased levels of unconjugated bilirubin and “physiologic” jaundice result. At this time, a normal baby may have a serum bilirubin level of up to 15 mg/dL, most of which is unconjugated. This level, which would be alarming in adult practice, should fall back to baseline by about 10 days of age. Because excessive jaundice can lead to kernicterus and result in severe brain damage, the measurement of blood conjugated and unconjugated bilirubin has an important role in pediatrics. An alternative means of reducing high unconjugated circulating bilirubin levels is phototherapy with ultraviolet light, which causes bilirubin to be converted to a potentially less toxic and more readily excreted metabolite. Severe cases may require an exchange transfusion. Complete absence of the bilirubin-conjugating enzyme results in severe persistent jaundice and Crigler-Najjar disease, a rare genetic disease. It is important to differentiate Crigler-Najjar from physiologic immaturity because treatment options vary considerably.
CASE STUDY 35.1
A newborn infant was born with a bilirubin level of 7 mg/dL (mostly unconjugated) that rose to 10 mg/dL by the 3rd day of life. The infant was breast-fed normally, and the bilirubin levels returned to normal values within 2 weeks without treatment.
questions
1. What is the most likely diagnosis? Are there likely to be any long-term adverse health effects?
2. What is the most likely biochemical cause of the elevated bilirubin level?
3. What other disorders can cause unconjugated hyperbilirubinemia in neonates?
Energy Metabolism
The liver plays an essential role in energy metabolism for the whole body (Table 35.9). Carbohydrates derived from the diet as disaccharides or polysaccharides form the bulk of our energy sources. They are broken down into simpler monosaccharides, which reach the liver via the portal blood system. The primary sugars in newborns and infants come from the breakdown of disaccharide lactose in milk. Lactose is broken down to glucose and galactose. When it reaches the hepatocytes, galactose is converted to glucose by a series of enzymic reactions that have unique pediatric significance. Genetic deficiency of any of the reactions results in failure to convert galactose to glucose and essentially reduce the energy content of milk by 50%. The most common cause of failure to convert galactose to glucose results in galactosemia or deficiency of galactose-1-phosphate uridyltransferase, a serious genetic disease of the newborn. In this disease, galactose-1-phosphate accumulates inside liver cells and causes hepatocellular damage and rapid liver failure. Other organs are also involved with this disease, including the renal tubules and the eyes. Galactose-1-phosphate accumulation causes acute renal tubular failure and tubular loss of glucose, phosphate, and amino acids. The loss of glucose in cooperation with the liver damage results in severe hypoglycemia. Accumulation of galactose in the eye results in cataract formation. A simple test that directs us to the diagnosis of galactosemia is the urine-reducing substance test. This detects the presence of non–glucose-reducing sugars (galactose) in urine when a child is symptomatic. The clinical significance of establishing a diagnosis is clear; galactosemia is often fatal if undiscovered but completely treatable by dietary lactose restriction when diagnosis is made.
TABLE 35.9 Important Biochemical Pathways in the Liver
Another critical pathway of carbohydrate energy metabolism in the newborn involves the pathway of gluconeogenesis. At birth, a term baby has sufficient liver glycogen stores to provide glucose as an energy source and maintain euglycemia. If the delivery is particularly stressful, these reserves of energy may become depleted prematurely. At this time, the normal physiologic role of gluconeogenesis, which essentially converts the amino acid alanine into glucose, becomes critical in maintaining glucose homeostasis. This pathway is not always mature at birth, and suboptimal operation results in what is termed physiologic hypoglycemia. Newborns can survive blood glucose levels below 30 mg/dL, although adults would fall into rapid hypoglycemic coma and risk sudden death at these levels. Physiologic hypoglycemia usually corrects quickly as the enzyme systems mature or by simple intravenous glucose infusion. Persistent and severe hypoglycemia should alert the physician to a possible inborn error of metabolism, such as galactosemia, gluconeogenesis, or disorders of fatty acid oxidative metabolism.
Diabetes
Blood glucose homeostasis and hepatic metabolism of glucose are maintained by the concerted actions of several hormones.8 Following a meal, the level of glucose in the circulation rises, which triggers increased synthesis and release of insulin by the pancreatic β cells of the islets of Langerhans. Increased levels of insulin in the circulation cause glucose to be taken up by certain cells, such as hepatocytes and muscle cells, and to be converted into glycogen as a future source of energy. As a result of the insulin action, blood glucose levels begin to fall to the preprandial level. Glucagon, a hormone secreted by the α cells of the islets of Langerhans, has an opposing effect to that of insulin. It is generally believed that the insulin–glucagon ratio, rather than absolute amounts of either, is the primary endocrine modulator of circulating glucose levels. Other hormones, including cortisol, epinephrine, and insulin-like growth factor (IGF), can also affect glucose levels. These hormones are secreted in response to stress and can affect glucose measurement when samples are collected under stressful situations.
Diabetes mellitus, a condition in which the endocrine control of glucose metabolism is abnormal, is usually related to failure of the insulin regulatory pathway. Type 1 diabetes (insulin dependent) is the most common in pediatrics. This may be caused by failure of the pancreas to secrete insulin or by the presence of circulating insulin antibodies that reduce the ability for endocrine action. This type of diabetes can be further classified as immune mediated or idiopathic. In the immune-mediated form (the most common form), the insulin-secreting beta cells are destroyed by a T-cell–mediated autoimmune response. This form of diabetes was previously termed “juvenile diabetes” because it is the predominant form in children. A patient typically presents with diabetic ketoacidosis, with profound hyperglycemia and metabolic acidosis that result from the liver increasing fatty acid metabolism and producing excess ketone bodies.
Type 2 diabetes (non–insulin dependent) is normally associated with increased resistance to normally secreted insulin in obese individuals. Obesity, particularly of the central (abdominal) region, is the most important risk factor for developing type 2 diabetes as it is strongly associated with insulin resistance, which, when coupled with relative insulin deficiency, leads to the overt development of type 2 diabetes. During the past 30 years, the number of children diagnosed as being overweight has increased by >100%, and this epidemic of childhood obesity is causing children to suffer from chronic complications that were previously only seen in adults.9 Type 2 diabetes now accounts for a considerable proportion of newly diagnosed cases of diabetes in the pediatric population.
To be diagnosed with diabetes mellitus, an individual must demonstrate one of the following diagnostic criteria: fasting plasma glucose level ≥126 mg/dL (7.0 mmol/L), plasma glucose ≥ 200 mg/dL (11.1 mmol/L) 2 hours after a 75-g oral glucose load, symptoms of hyperglycemia and random plasma glucose ≥200 mg/dL (11.1 mmol/L), or glycated hemoglobin (Hb A1C) ≥ 6.5%.10 A positive result on any of the above should be repeated on a different day prior to the diagnosis of diabetes.
Urbanization, unhealthy diets, and increasingly sedentary lifestyles have contributed to increase the prevalence of childhood obesity, particularly in developed countries.11 The metabolic syndrome is a combination of medical disorders that when occurring together, greatly increase the chance of cardiovascular disease and diabetes. Several definitions of the metabolic syndrome exist but generally include diabetes or impaired glucose resistance, hypertension, dyslipidemia (increased triglycerides and decreased HDL), and central obesity. The link between obesity, metabolic syndrome, and type 2 diabetes has been well characterized in adult populations but has been increasingly observed in pediatric populations. In children and adolescents, a number of studies have demonstrated a link between childhood metabolic syndrome and elevated cardiovascular risk in later life.11
It is important to recognize diabetes as a cause of hyperglycemia in children and to distinguish it from other medical causes of high blood sugar, including acute pancreatic disease or hypersecretion of counterregulatory hormones such as growth hormone (GH), cortisol, or catecholamines. Chronic hyperglycemia can be readily distinguished from acute causes by simply measuring the blood concentration of glycated hemoglobin or hemoglobin A1C, a well-established marker for long-term hyperglycemia. In addition to being included as a diagnostic criteria of diabetes, this assay also plays a major role in monitoring compliance in diabetic patients on treatment.10
Nitrogen Metabolism
The liver plays a central role in nitrogen metabolism. It is involved with the metabolic interconversions of amino acids and the synthesis of nonessential amino acids. The liver synthesizes many body proteins, including most proteins found in the circulation, such as albumin, transferrin, and the complement clotting factors. The liver does not synthesize immunoglobulins. The liver is also responsible for complete metabolism of the breakdown products of nitrogen turnover, such as ammonia and urea through the urea cycle and creatinine and uric acid from energy stores and nucleic acids, respectively. Blood ammonia levels are higher in the newborn period than in later life, presumably due to immaturity of urea cycle enzymes and the portal circulation. A blood ammonia level of 100 μmol/L in a newborn would be regarded as less significant than the same level in a 1-year-old. Persistently elevated ammonia levels should alert the investigator to possible liver damage and secondary failure of the urea cycle. High ammonia levels suggest a possible primary defect in the urea cycle, and patients should be evaluated for such a defect.
Nitrogenous End Products as Markers of Renal Function
In contrast to the high neonatal ammonia levels, creatinine and uric acid levels are lower in newborns. Both metabolites rise eventually to normal adult ranges. Creatinine concentrations in blood increase with muscle mass and may vary with diet (i.e., meat ingestion). It is filtered at the glomerulus and not extensively reabsorbed by the renal tubules. Its measurement as a clearance ratio in blood and in a 24-hour urine sample has been used as a marker for the GFR for many years. Creatinine clearance is calculated from the creatinine concentration in the collected urine sample, the urine flow rate, and the plasma creatinine concentration. Creatine clearance calculations are not commonly performed anymore due to the difficulties in collecting a complete 24-hour urine specimen and the fact that newer, more convenient methods to estimate the glomerular filtration rate (eGFR) using a single plasma creatinine value are commonly used.
While several different equations exist to estimate GFR from plasma creatinine values in adults, the most widely used is the Modification of Diet in Renal Disease (MDRD) formula.12 The equation estimates GFR from the patient’s serum creatinine (mg/dL), age, sex, and ethnicity as follows:
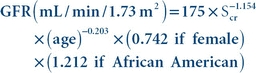
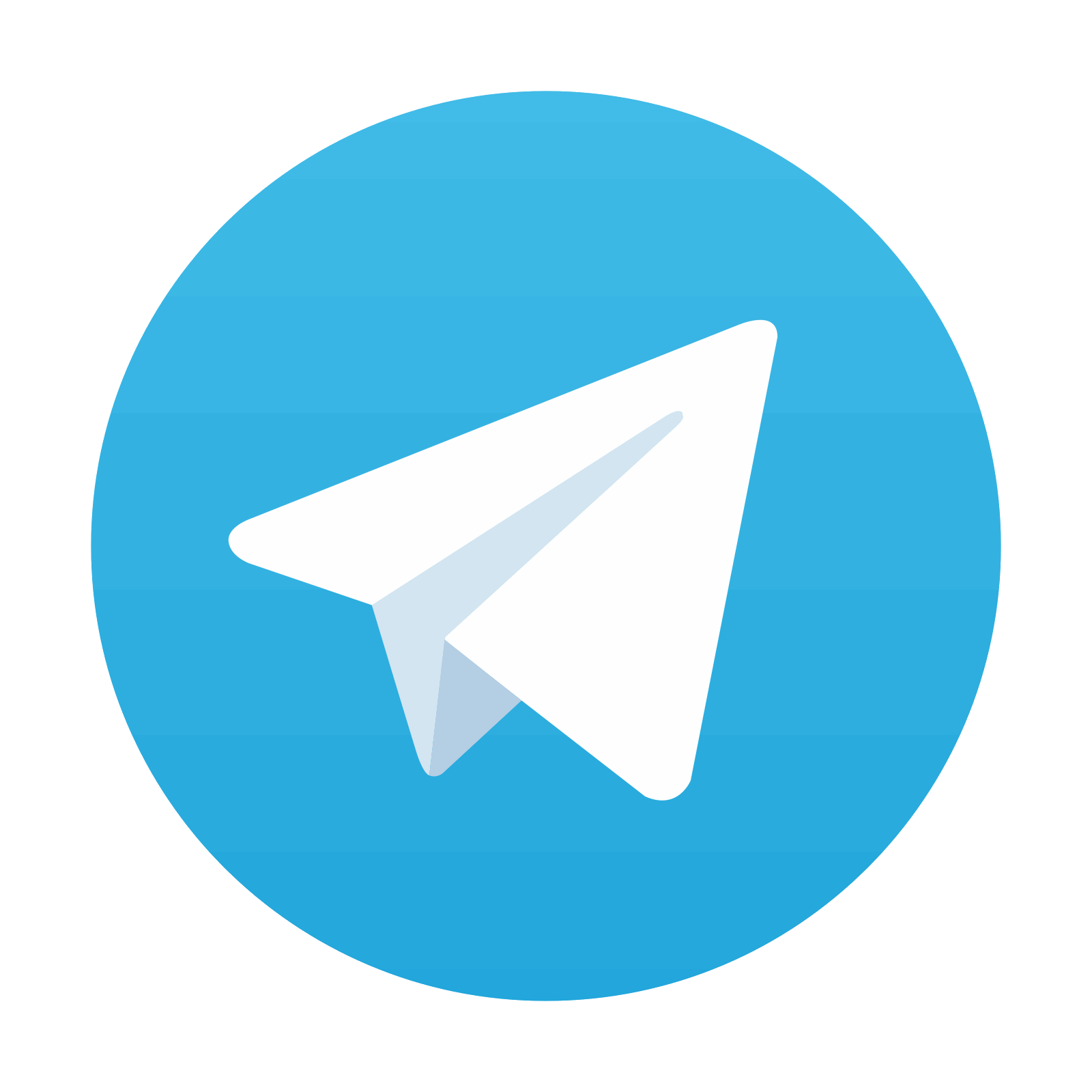
Stay updated, free articles. Join our Telegram channel

Full access? Get Clinical Tree
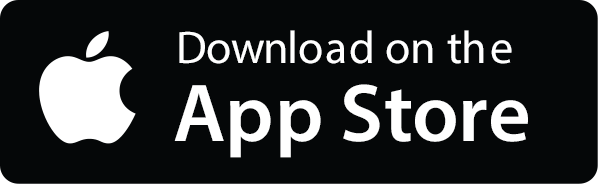
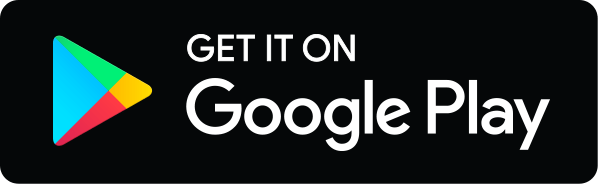