20 Four principal types of neuroglia are recognised: • Oligodendrocytes are the CNS equivalent of the Schwann cells of the peripheral nervous system and are responsible for the formation of myelin sheaths in the CNS. • Astrocytes are highly branched cells that pack the interstices between the neurones, their processes and oligodendrocytes. They provide mechanical support as well as mediating the exchange of metabolites between neurones and the vascular system. They also form part of the blood-brain barrier. Astrocytes play an important role in repair of CNS tissue after damage • Microglia are the CNS representatives of the monocyte-macrophage system and have defence and immunological functions. • Ependymal cells make up a specialised epithelium which lines the ventricles and spinal canal FIG. 20.1 Grey matter FIG. 20.2 Astrocytes FIG. 20.3 White matter CNS myelin and oligodendrocytes (illustrations (e) and (f) opposite) FIG. 20.4 Microglia FIG. 20.5 Main anatomical divisions in the CNS • The grey mater over the surface of the brain is termed the cortex. • A discrete arrangement of neurones, often with a specific function, is termed a nucleus. • An arrangement of neuronal cells running along the spinal cord is termed a column. • A defined bundle of axons running in white matter is termed a tract or a fascicle. FIG. 20.6 Choroid plexus FIG. 20.7 Ependyma FIG. 20.8 Meninges
Central nervous system
H&E (HP)
Common staining methods permit neurones N to be readily distinguished from glial cells. While neurones vary greatly in different regions of the brain, they are usually recognisable by their large nuclei, prominent nucleoli and dispersed chromatin. There is usually extensive basophilic granular cytoplasm, and parts of one or more processes may be visible, often due to processing artefact.
Types of neuroglia are difficult to differentiate from each other with certainty by common staining methods. In the mature CNS, as in this specimen, oligodendrocytes O have small round condensed nuclei; their cytoplasm is unstained by routine methods, including H&E. In grey matter, oligodendrocytes are not only scattered between the nerve cell bodies along with the astrocytes but also tend to be aggregated around the neurone cell bodies. Other glial cells in the image, marked A, are probably astrocytes.
The nuclei of both neurones and neuroglia are surrounded by a dense network of branching cytoplasmic cell processes, axons and dendrites. This is seen as a fibrillar eosinophilic material on H&E and is called neuropil Np. Only some of the fibres are myelinated axons.
(a) Diagram (b) Immunohistochemical method for glial fibrillary acidic protein (HP)
(c) EM ×12 000 (d) EM ×57 500
Astrocytes are identified by immunohistochemical staining for a protein called glial fibrillary acidic protein (GFAP) in micrograph (b); these are the most numerous glial cells in grey matter. They have long, branched processes which occupy much of the interneuronal spaces in the neuropil. In grey matter, many of the astrocyte processes end in terminal expansions adjacent to the non-synaptic regions of neurones. Other processes of the same astrocytes terminate upon the basement membranes of capillaries; these perivascular feet cover most of the surface of the capillary basement membranes and form part of the blood-brain barrier as illustrated in the diagram (a). Similar foot processes invest the basement membrane between the CNS and the innermost layer of the meninges, the pia mater (see Fig. 20.9), forming a relatively impermeable barrier called the glia limitans. Astrocytes mediate metabolic exchange between neurones and blood and regulate the composition of the intercellular environment of the CNS.
All astrocytes contain bundles of intermediate filaments and microtubules. The intermediate filaments are formed of GFAP, which is characteristic of astrocytes. The astrocytes of grey matter have numerous short, highly branched cytoplasmic processes and are described as protoplasmic astrocytes. These are demonstrated in micrograph (b) by using immunohistochemical staining for GFAP. By contrast, the astrocytes of white matter have relatively few and straight cytoplasmic processes rich in intermediate filaments and are known as fibrous astrocytes.
Micrograph (c) shows an astrocyte A lying adjacent to a nerve cell body N in the cerebral cortex. The astrocyte cytoplasm contains many ribosomes, a little rough endoplasmic reticulum and a few small mitochondria and lysosomes. The origins of several cytoplasmic extensions C can be identified. The cytoplasm appears moderately electron-dense due to its content of intermediate filaments IF, which can be seen at higher magnification in micrograph (d). Typical of CNS grey matter, the adjacent neuropil Np contains numerous neuronal and glial processes in various planes of section; some myelinated axons M are included in the field.
(a) H&E (MP) (b) H&E (HP) (c) TS, solochrome cyanin (HP) (d) LS, solochrome cyanin (HP) (e) EM ×13 000 (f) Schematic diagram
White matter consists of nerve fibres (axons), often myelinated by oligodendrocytes, organised in tracts, with supporting astrocytes, microglia and vessels. Oligodendrocytes were so named because original heavy metal impregnation methods showed that they only had a small number of short, branched processes (Greek: oligos = few, dendron = tree). It is now known that oligodendrocytes are responsible for myelination of axons and the processes originally described are the short pedicles that connect the cell body to the myelin sheaths.
A single oligodendrocyte can contribute to the myelination of up to 50 axons from the same or different fibre tracts, as illustrated in the diagram (f). Conversely, any one axon will require the services of numerous different oligodendrocytes because of the limited length of the myelin segments (internodes) produced by each oligodendrocyte. The mechanism of myelin sheath formation is very similar to that of Schwann cells in peripheral nerve (see Fig. 7.6). Oligodendrocytes are the predominant type of neuroglia in white matter, as well as being abundant in grey matter.
Micrographs (a) and (b) show CNS white matter, with the oligodendrocyte nuclei ON often having an artefactual perinuclear halo and a few larger astrocyte nuclei A. Micrographs (c) is of a myelin stain, with the transverse ring-shaped profiles of blue-stained myelin each surrounding an axon, unstained and not visible in this preparation. Micrograph (d) is of longitudinal fibres using the same stain. Oligodendrocyte nuclei ON can be seen as rounded red-stained profiles.
Oligodendrocytes aggregate closely around nerve cell bodies in the grey matter, where they are thought to have a support function analogous to that of the satellite cells which surround nerve cell bodies in peripheral ganglia (see Fig. 7.20).
The electron micrograph (e) shows an oligodendrocyte O lying adjacent to a nerve cell body N, with a neuronal dendrite D at the upper right. The oligodendrocyte contains prominent rough endoplasmic reticulum, ribosomes and Golgi apparatus G. The commencement of a cytoplasmic process C is seen. The remainder of the image shows the complexity of the neuropil Np, comprising glial and neuronal processes including myelinated axons M.
Myelin sheath formation begins in the CNS of the human embryo at about 4 months gestation, with the formation of most sheaths at least commenced by about the age of 1 year. From this time, successive layers continue to be laid down, with final myelin sheath thickness being achieved by the time of physical maturity.
(a) Diagram (b) Ricinus communis agglutinin (HP) (c) Immunohistochemical method for CD68 (MP)
Microglia are small cells derived from cells of mesenchymal origin which invade the CNS at a late stage of fetal development. As shown in the diagram, microglia have elongated nuclei and relatively little cytoplasm, which forms fine highly branched processes. In consequence, they are difficult to identify in conventional preparations for light microscopy.
Immunohistochemical staining provides the best way to see microglia. Micrograph (b) shows the ramified profile of microglia cells, identified by staining using ricinus communis agglutinin which binds to sugars on the membrane of this cell type. Micrograph (c) highlights the distribution of this cell type in white matter from the cerebral hemisphere. The rounded nuclei in the background are mainly oligodendrocytes.
In response to tissue damage, microglia transform into large amoeboid phagocytic cells and are thus the CNS representatives of the macrophage-monocyte defence system (see Fig. 4.19). CD68 stains cells of macrophage lineage, including microglial cells. Other macrophages, distinct from microglia, are present in the space surrounding the CNS capillaries but are separated from the CNS compartment proper by the perivascular feet of astrocytes.
This diagram shows the brain and spinal cord viewed in a sagittal section. The main part of the brain consists of the paired cerebral hemispheres H which are invested by the cerebral cortex. Deep within the brain are large grey matter structures which form the diencephalon Di. Included in this region are the basal ganglia and thalamus. The cerebral hemispheres are connected to structures below by the midbrain B. In this region, there are important nuclei including the substantia nigra. The pons P connects to the medulla M as well as to the cerebellum C. The pons, medulla and midbrain are often collectively termed the brainstem. The spinal cord S extends from the lower end of the medulla.
Specific terms are used to describe arrangements of cells and their connections:
(a) H&E (LP) (b) H&E (HP)
The choroid plexus is a vascular structure arising from the wall of each of the four ventricles of the brain and responsible for the production of cerebrospinal fluid (CSF). CSF drains from the interconnected ventricular cavities via three channels connecting the fourth ventricle with the subarachnoid space which surrounds the CNS. CSF is produced at a constant rate and is reabsorbed from the subarachnoid space into the superior sagittal venous sinus, via finger-like projections called arachnoid villi. Thus the CNS is suspended in a constantly circulating fluid medium which acts as a support and shock absorber.
Each choroid plexus consists of a branching system of blood vessels which run in fronds composed of collagenous tissue and covered by a cuboidal or columnar epithelium. The choroid plexus is therefore a villous structure. Micrograph (a) shows the choroid plexus CP within a ventricle of the brain V.
Micrograph (b) shows detail of one of the choroid plexus processes. The capillaries and vessels of the choroid plexus are large, thin-walled and sometimes fenestrated lying in a fibrous core. The epithelial cells rest on a basal lamina. At the ultrastructural level, long bulbous microvilli project from the luminal surfaces of the choroid plexus epithelial cells and their cytoplasm contains numerous mitochondria, features which suggest that the elaboration of CSF is an active process.
The mode of CSF secretion involves active secretion of sodium ions by choroid epithelial cells into the CSF, followed by passive movement of water from the local vessels. Continuous tight junctions (zonula occludens) between epithelial cells contribute to a blood-CSF barrier, preventing ingress of almost all other molecules.
H&E (HP)
Ependymal cells form the lining of the ventricles and spinal canal. Cuboidal or low columnar in shape, the cells are tightly bound together at their luminal surfaces by the usual epithelial junctional complexes. Unlike epithelia, however, ependymal cells do not rest on a basement membrane but, rather, the bases of the cells taper and then branch into fine processes which ramify within the underlying layer of processes derived from astrocytes. At the luminal surface, there are a variable number of cilia. Microvilli are also present and probably have absorptive and secretory functions. The ependymal layer tends to become incomplete with increasing age.
(a) Diagram (b) Dura mater, H&E (MP) (c) H&E (LP)
The brain and spinal cord are invested by three layers of supporting tissue, collectively called the meninges. The surface of the nervous tissue is covered by a delicate layer called the pia mater, containing collagen fibres, fine elastin fibres and occasional fibroblasts, separated from the astrocytic processes of underlying CNS parenchyma by a basement membrane. The basement membrane is completely invested by astrocytic processes, the two layers forming the impermeable glia limitans.
Overlying the pia mater is a thicker fibrous layer, the arachnoid mater, which derives its name from the presence of cobweb-like strands which connect it to the underlying pia; since the pia and arachnoid are continuous, they may be considered as a unit, the pia-arachnoid, also called the leptomeninges. The space between the pia and arachnoid is called the subarachnoid space and, in places, forms large cisterns. This subarachnoid space is connected with the ventricular system by three foramina in the fourth ventricle (in the brainstem), and CSF circulates continuously from the ventricles into the subarachnoid space.
The subarachnoid space is lined by flattened arachnoidal cells. The outer surface of the arachnoid mater is also lined by flat cells. As shown in the diagram, arteries and veins passing to and from the CNS pass in the subarachnoid space loosely attached to the pia mater and invested by subarachnoid meningothelium. As the larger vessels extend into the nervous tissue, they are surrounded by a delicate sleeve of pia mater. Between the penetrating vessels and the pia there is a perivascular space. In humans, the pia blends with the adventitia of the vessel as it penetrates the brain, thus separating the perivascular space from the subarachnoid space. This pia component is not present around the capillaries of the CNS.
External to the arachnoid mater is a dense fibroelastic layer called the dura mater D, micrograph (b), which is lined on its internal surface by flat cells. The dura is closely applied to but not connected with the arachnoid layer and there is the potential for a space, the subdural space, to develop between the two layers. In the cranium, the dura mater merges with the periosteum of the skull but also extends into the brain space as several folds. A large fold, the falx, extends along the midline from top of the skull into the space between the cerebral hemispheres while a horizontal fold, the tentorium, is attached to the posterior skull and extends into the space between the cerebral hemispheres and cerebellum. These folds help support the brain and contain venous sinuses, forming part of the brain’s venous return system.
Around the spinal cord, dura is suspended from the periosteum of the spinal canal by denticulate ligaments, the intervening epidural space being filled with loose fibrofatty tissue and a venous plexus.
The pia and arachnoid layers of the brain meninges are illustrated in micrograph (c). Pia mater P is attached to the surface of the brain and continues into the suclus S and around the penetrating vessels. The arachnoid mater A appears to be a completely separate layer and bridges the sulcus. Meningeal vessels lie in the subarachnoid space.
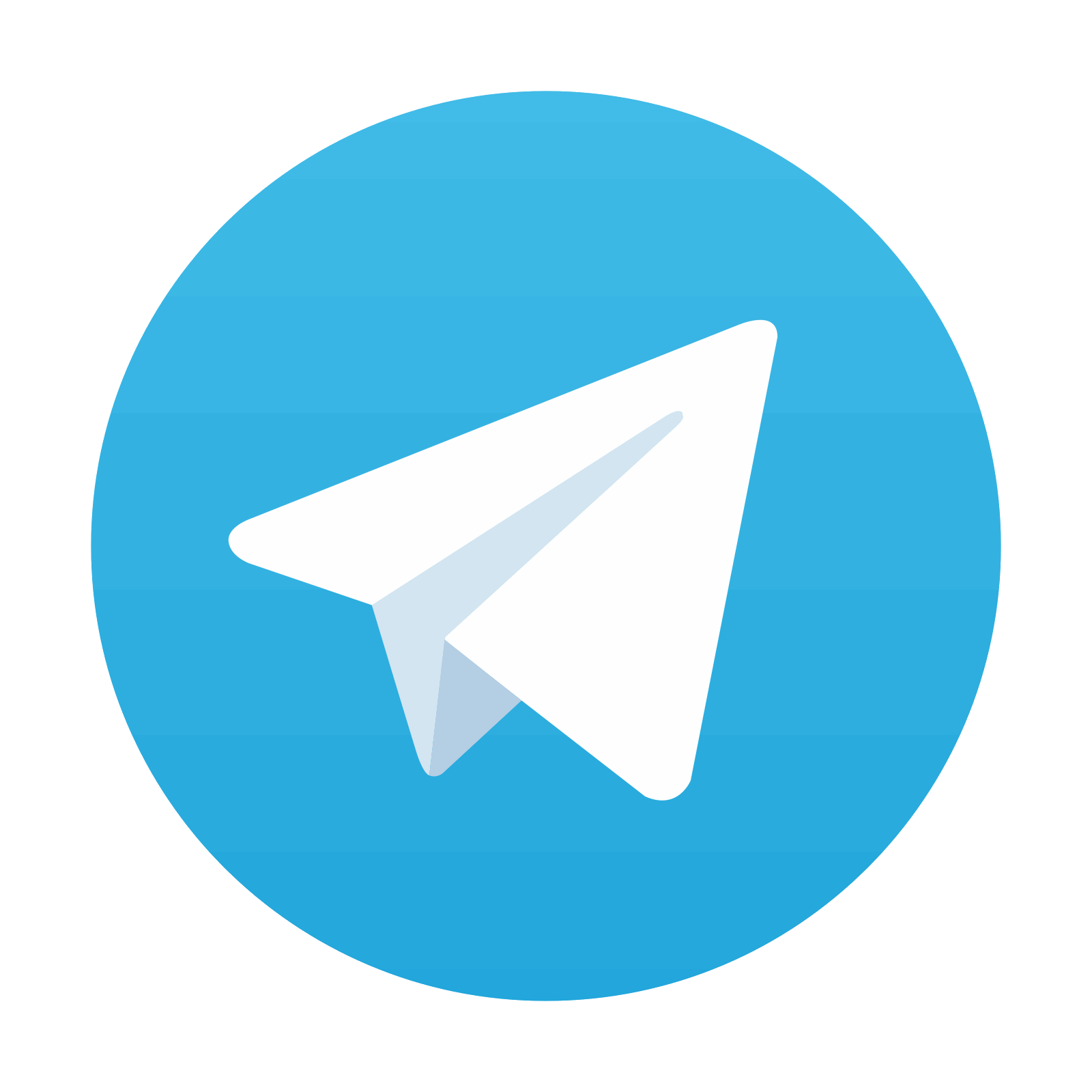
Stay updated, free articles. Join our Telegram channel

Full access? Get Clinical Tree
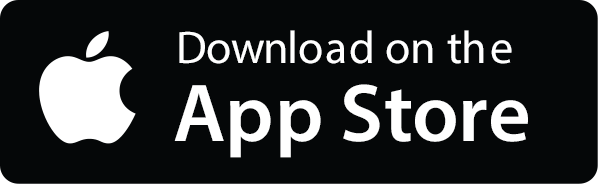
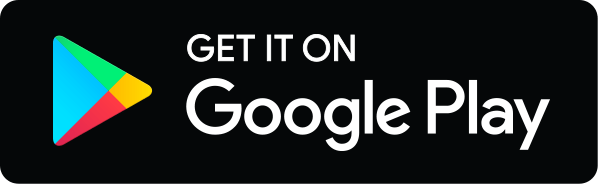