18.1.1 Limbal Epithelium
Limbus region of the eye houses limbal epithelial stem cells (LESC). This niche is thought to be located in the palisades of Vogt (Hirano et al. 2003) which is an optimal microenvironment for stem cell growth (Goldberg et al. 1982; Lagali et al. 2013; Goldberg and Bron 1982). However, there is no specific marker for the LESC, and therefore, the expression of putative stem cell markers and lack of differentiation-related marker (K3/K12), morphology (Arpitha et al. 2005), clone formation assay (Pellegrini et al. 1999), and DNA retention study (Schermer et al. 1986; Cotsarelis et al. 1989) are considered for the identification of LEST cells (Budak et al. 2005; De Paiva et al. 2005; Di Iorio et al. 2005).
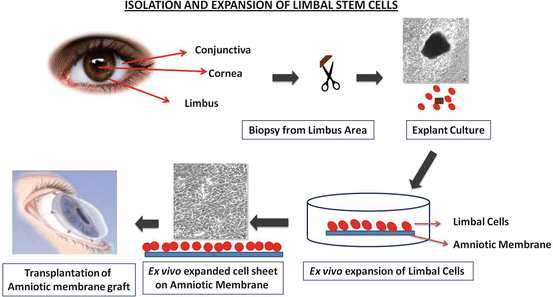
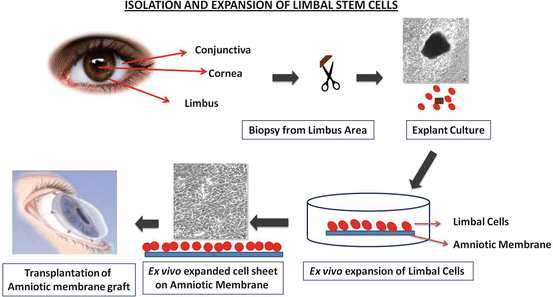
There has been a great deal of improvisation in the technique of isolation of LESC for clinical use.
1.
Conjunctival limbal autograft (CLAU): In its simplest form, conjunctival limbal autograft has been successfully used in the treatment of unilateral LSCD. However, there is a concern of inducing LSCD in the donor eye, therefore leading to its modification involving a smaller source tissue in conjunction with in vivo expansion. While these methods have been successful for patients with unilateral LSCD, similar approaches with conjunctival limbal allograft (due to bilateral LSCD) have been largely unsuccessful due to high frequency of immune rejection Since the first report in 1989 by Kenyon and Tseng, CLAU has become a widely accepted technique in the management of unilateral total LSCD (Kenyon and Tseng 1989)
2.
Corneal stem cell allograft transplantation: Allograft transplantation for the patients suffering from bilateral total LSCD or whose fellow eye is not suitable as a graft source. Generally, allograft transplantation includes cadaveric keratolimbal allograft (KLAL) and living-related conjunctival limbal allograft (Lr-CLAL) (Shanmuganathan et al. 2007; Huang et al. 2011; Lam et al. 2000; Rao et al. 1999). Due to high risk of immune rejection, both methods offer poor long-term outcomes.
3.
Simple limbal epithelial transplantation (SLET): The use of amniotic membrane for in vivo expansion of smaller graft tissue improved the success rate of limbal epithelial transplant (Meallet et al. 2003; Mittal et al. 2006). Amniotic membrane seems to inhibit inflammation and provide a supportive niche for the transplanted LEST cells. In 2012, Sangwan VS (Sangwan et al. 2012) described this surgical technique. In this procedure, a small 2 × 2 mm strip was removed from the fellow eye and chopped into pieces. Then, the tiny pieces were seeded on the amniotic membrane (AM)-covered cornea. Complete reconstruction with epithelialized, avascular, and stable corneal surface was observed after 6 weeks in all 6 recipient eye.
4.
Corneal Limbal Epithelial Transplant (CLET): The cells used for cultured limbal epithelial transplantation (CLET) are obtained from a relatively small biopsy of limbal tissue. This is grown on a denuded amniotic membrane (Fig. 18.1) which is used as a cell substrate to facilitate transfer of the cells from culture to recipient cornea. Several materials such as fibrin gels (Han et al. 2002; Rama et al. 2001; Talbot et al. 2006), collagen (Dravida et al. 2008; McIntosh Ambrose et al. 2009; Takezawa et al. 2004), keratin films (Borrelli et al. 2013; Feng et al. 2014), silk fibroin films (Bray et al. 2011), chitosan hydrogels (Grolik et al. 2012), siloxane–hydrogel contact lens (Di Girolamo et al. 2007), polystyrene, and nanofiber scaffold (Sharma et al. 2011a) (Figs. 18.2 and 18.3) have been tested as scaffolds. All of these material have been found to support the growth of LEST cells in vivo, but only human amniotic membrane and fibrin gels have been investigated in clinical studies with positive outcomes (Fig. 18.2).
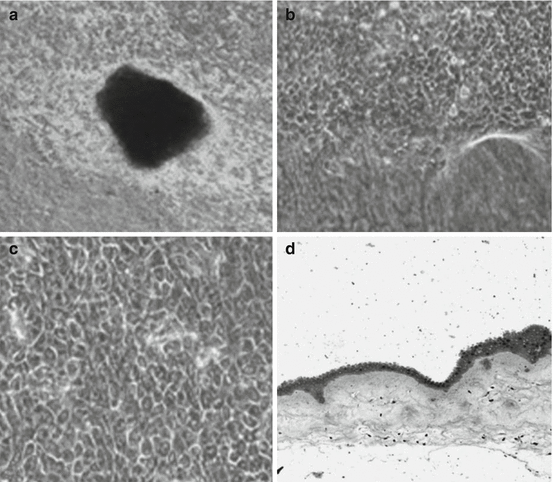
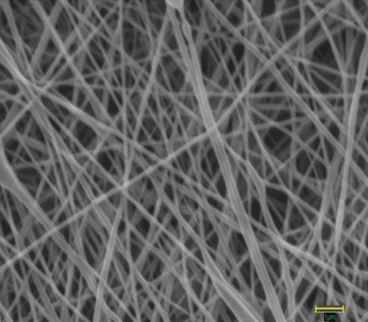
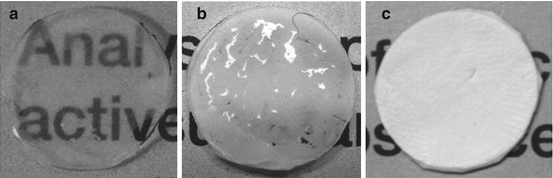
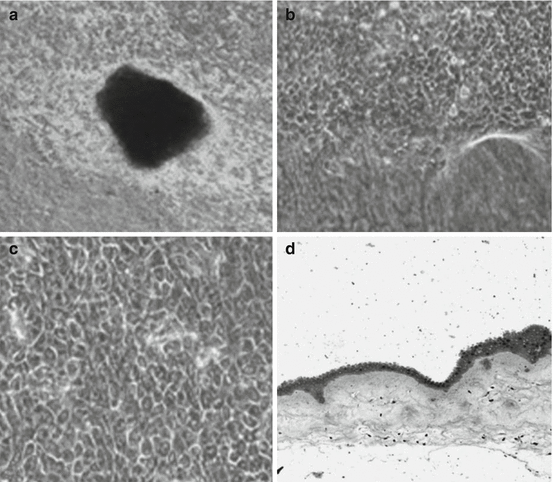
Fig. 18.1
Phase contrast light microscopic pictures of human LESCs cultivated on dHAM. (a) Picture at low magnification (32×) on third day showing expansion of LESCs from the edge of the explant. (b) Cultured limbal epithelial cells at higher magnification (310×) showing migration of limbal epithelial peripherally outward. (c) Picture at 320× magnification showing formation of monolayer with a typical honeycomb-like structure and hexagonal morphology of the cells. (d) Hematoxylin and eosin staining showing formation of multilayer of cultivated limbal epithelial cells at low magnification
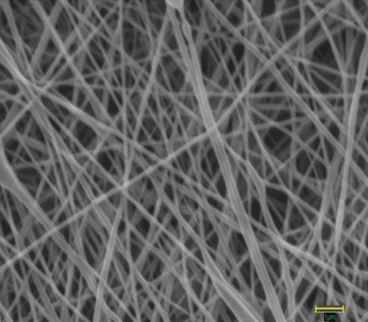
Fig. 18.2
Architecture of electrospun PCL nanofiber scaffold as seen under a scanning electron microscope at 25,000× magnification. The average fiber diameter of nanofibers was 132 ± 42 nm. Scale bar measures 1 μm
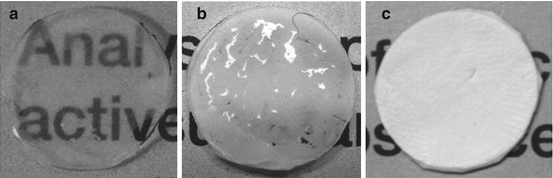
Fig. 18.3
Optical transparency of PCL nanofiber membranes and HAM. (a) Wet HAM showing transparency through which the printed text can be easily read. (b) Wet PCL membrane showing translucency through which the printed text is slightly visible. (c) Dry PCL membrane showing complete opacity and the text underneath cannot be read through it
Due to the high risk of rejection associated with allografts, ex vivo expansion of limbal epithelial stem cells is a preferable solution for bilateral LSCD.
CLET may also have a reduced risk of allograft rejection compared with direct tissue transfer because antigen-presenting macrophages do not survive the process of ex vivo culture. Limbal epithelial stem cells (LESCs) transplantation has been clinically recognized of therapeutic value in hereditary conditions such as aniridia (Gomes et al. 2005) and in acquired diseases characterized by LESC deficiency, such as SJS, chemical or thermal injury, chronic limbitis, limbal surgery, and contact lens keratopathy (Tsubota et al. 1999). Successful transplants of cultured autologous limbal epithelium in patients with unilateral limbal stem cell deficiency have been achieved (Sharma et al. 2011b) (Fig. 18.4).
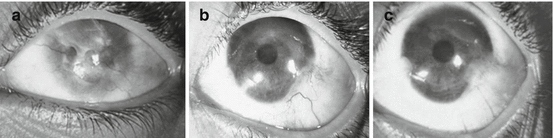
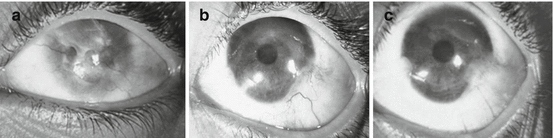
Fig. 18.4
Representative case of patient no. 8, a 10-year-old girl who suffered from lime injury. Patient underwent autologous ex vivo cultured LSCT. (a) Preoperative clinical appearance of ocular surface with 360° of conjunctivalization, corneal haze, and VA finger counting close to the face (FCCF). (b) Three-month postoperative clinical appearance with improved VA 3/60 and ocular surface. (c) 24-month postoperative appearance with stable VA 3/60 and ocular surface
18.1.2 Oral Mucosal Epithelium
Apart from limbus tissue-derived SC, oral mucosal tissue has also been tested as an alternative stem cell source. The safety and efficiency of oral mucosal epithelium base transplantation have been evaluated clinically. Several groups from Japan demonstrated that cultured oral mucosal epithelium can be used to reconstruct the corneal epithelium in animal models as well as patients with LSCD due to chemical injury and SJS (Nakamura et al. 2011; Sen et al. 2011).
1.
Cultured oral mucosa stem cell transplant (COMET): It is closely related to LSCT. It has also been used for LSCD treatment. Cultured oral mucosal epithelial transplantation (COMET) has several potential advantages. There are no risk of immune-mediated rejection, and, in the absence of autoimmune disease, immunosuppression is not required. Published outcomes for COMET show that a stable corneal surface is achieved in up to 100 % of eyes at 1 year, 100 % at 14 months, 67 % at 20 months, and 92 % at 4 years. Visual acuity was better than pretreatment levels in 90 % of eyes at 1 year, 100 % at 14 months, and 67 % at 20 months, and 53 % remained improved for 4 years following surgery (Figs. 18.5 and 18.6).
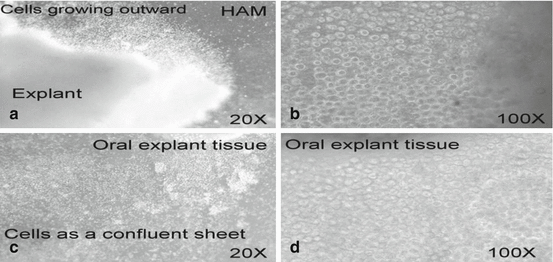
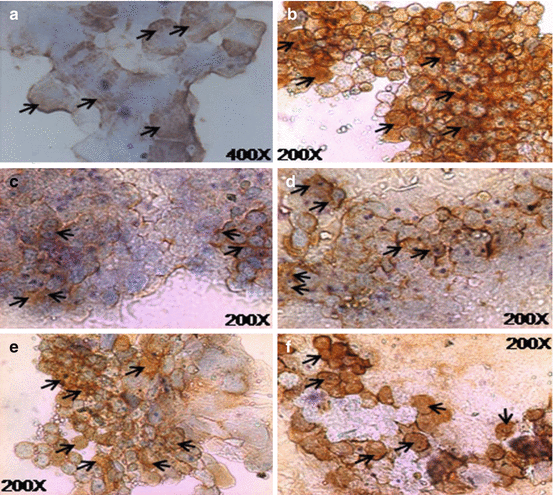
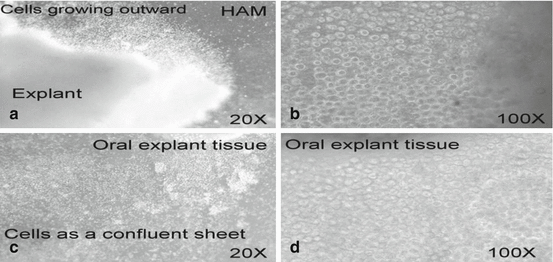
Fig. 18.5
(a, b) Morphologic findings of OMEC growing on HAM for 2–3 days. Magnification: (a) 20×; (b) 100× (c, d) OMEC as a confluent sheet on HAM (after 1–2 weeks). Magnification: (c) 20×; (d) 100×
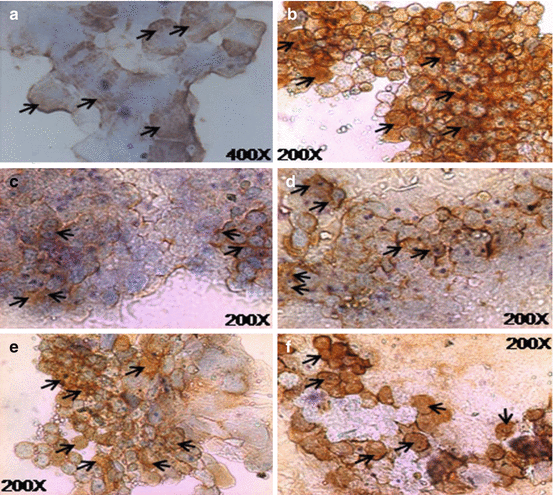
Fig. 18.6
Expression of various marker proteins as assessed by immunocytochemistry in cultivated OMEC for 1–2 weeks. (a) Expression of cytokeratin K3/K12; magnification, 400×. (b) Expression of connexin 43; magnification, 200×. (c) Expression of p63; magnification, 200×. (d) Expression of −1 integrin (CD29); magnification, 200×. (e) Expression of p75; magnification, 200×. (f) Expression of MUC1; magnification, 200×
18.1.3 Bone Marrow-Derived Stem Cells
Some studies have suggested that bone marrow-derived stem cells might be implicated in promoting corneal wound healing in vivo and in retinal disease (Ma et al. 2006; Otani et al. 2004; Ye et al. 2008; Kumar et al. 2012). Briefly, cultured human BM-MSCs on amniotic membrane were transplanted into chemically burned rat corneas achieving the same results in corneal epithelialization and vision acuity as achieved by same procedure using LESCs (Ma et al. 2006; Ye et al. 2008).
18.1.4 Hair Follicle Bulge Cells
Hair follicle bulge is an essential niche for keratinocyte stem cells (KSCs), and hair follicle stem cells have been found to successfully trans-differentiate into corneal epithelial-like cells (Ohyama 2007; Ohyama et al. 2006; Blazejewska et al. 2009). In preclinical studies, isolated autologous hair follicle bulge cells were expanded on a fibrin carrier in vitro and then transferred into the mice with LSCD. It contributes to the reconstruction of corneal epithelium by crossing the lineage boundaries and terminally differentiating into corneal epithelial-like cells.
18.1.5 Bioengineered Cornea
In vitro tissue engineering is an approach where healthy mammalian cells are used with a supporting matrix to produce a composite implant. Before the tissue can be assembled from specific cells and be ready for implantation, a series of highly orchestrated events in the correct sequential order must take place. To ensure reliable large-scale production of a durable and easily stored implant, the following criteria should be met:
Source of healthy self-renewing cells
Bioactive scaffolds with correct chemical/physical properties to promote cell
Differentiation/integration and tissue formation
In vitro conditions that mimic the in vivo environment
Non-immunogenic: biocompatible
Several materials such as human amniotic membrane (Sharma et al. 2011a), fibrin gels (Han et al. 2002; Rama et al. 2001; Talbot et al. 2006), collagen (Dravida et al. 2008; McIntosh Ambrose et al. 2009; Takezawa et al. 2004), keratin films (Borrelli et al. 2013; Feng et al. 2014), silk fibroin films (Bray et al. 2011), chitosan hydrogels (Grolik et al. 2012), siloxane–hydrogel contact lens (Di Girolamo et al. 2007), polystyrene (Takezawa et al. 2004), and nanofiber scaffold (Kuno and Fujii 2011; Sharma et al. 2011a) have been tested as scaffolds. All of these materials have been found to support the growth of LEST cells in vitro.
< div class='tao-gold-member'>
Only gold members can continue reading. Log In or Register a > to continue
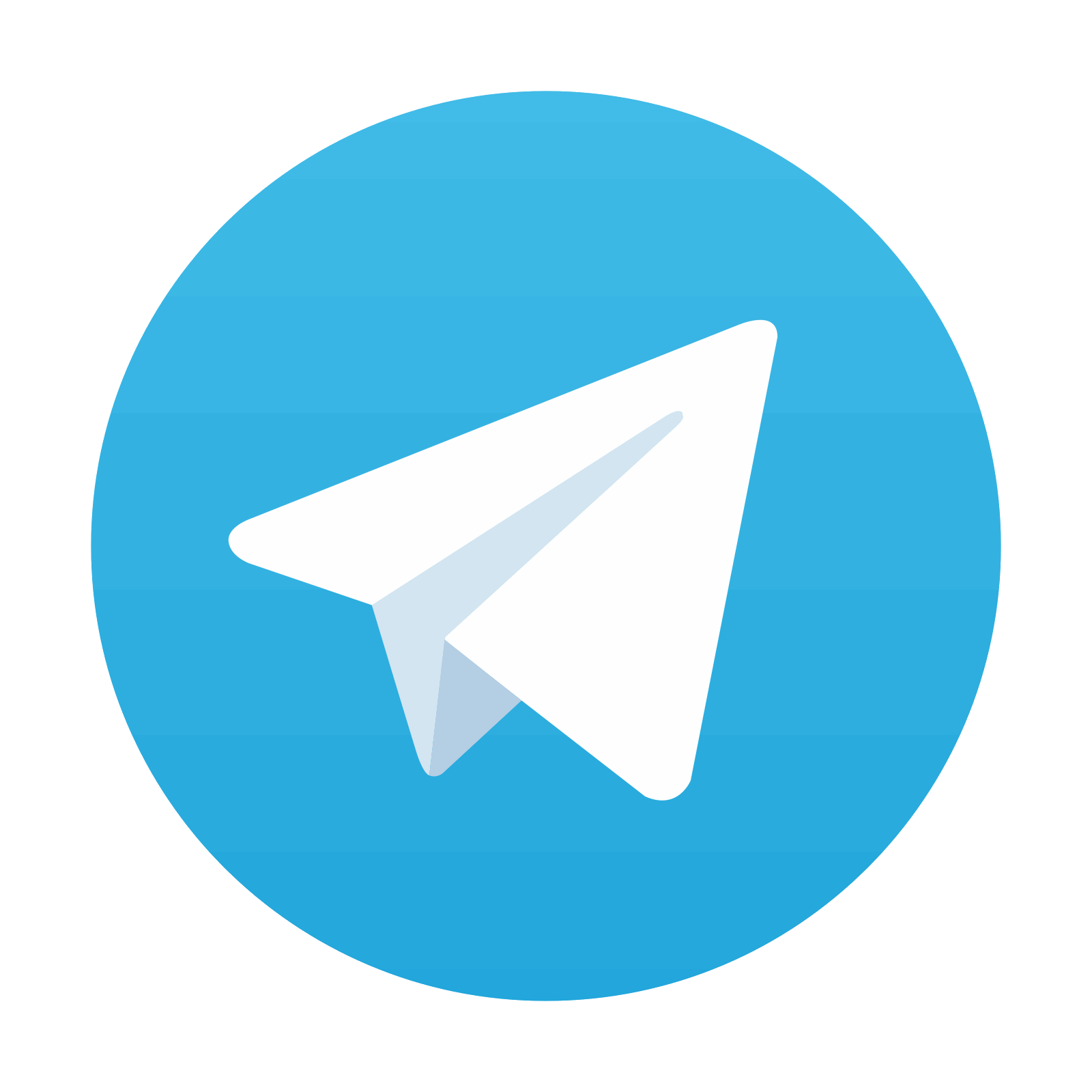
Stay updated, free articles. Join our Telegram channel

Full access? Get Clinical Tree
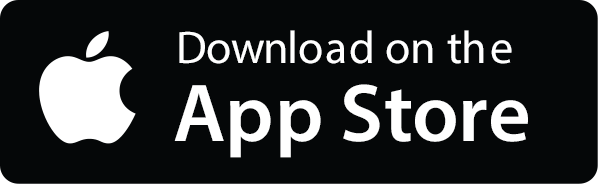
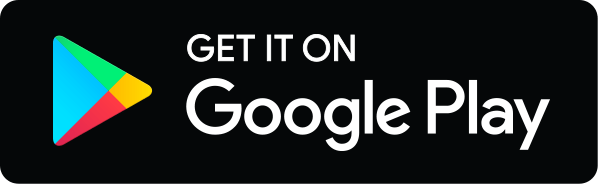