- Adhesive interactions between cells and their extracellular environment occur through extracellular matrix (ECM) receptors such as integrins, and intercellular adhesion receptors such as cadherins. The sites of physical interaction are called adhesion complexes. Integrins bind matrix proteins, while cadherins join cells together. Both organize intracellular cytostructure and transmit signals to the cell.
- Major aspects of cell physiology, including cell cycle progression, suppression of apoptosis, cellular differentiation, and migration, depend upon signals being received through adhesion complexes. The regulation of these cell fate choices is perturbed in cancer, and altered cell adhesion is central to cancer progression.
- The normal spatial organization of cells within tissues is determined by adhesive interactions. The transition from a primary tumor to a malignancy requires loss of both the proliferative and positional controls that would otherwise maintain correct cell number and architecture. The homeostasis of a whole affected organ is disrupted in cancer.
- Malignant cells move from their site of origin and disseminate to distant organs, where they form metastases. This progression requires the acquisition of a large number of characteristics, which involve changes in cell adhesion. These include migration, an ability to invade the stroma and endothelial linings, dissemination through the circulation, survival in inappropriate tissue contexts, and environmental decoding to allow a tumor to grow in defined secondary target sites. However, the formation of metastases is inefficient and rare.
- Reduced adhesiveness at adherens junctions is a key stage in malignant progression. Loss of E-cadherin occurs through mutations as well as phosphorylation, degradation, and altered transcription. It is also lost during the epithelial–mesenchymal transition.
- Invasive cells acquire the ability to migrate by altering their repertoire of integrins, activating the intracellular signaling machinery that controls the cytoskeleton and motile processes, and expressing ECM-remodeling enzymes. Cancer cells frequently migrate in collective groups rather than as single cells.
- Although most of the genetic changes in carcinoma occur within epithelial cells, the supporting tissue, or stroma, has a critical role in malignant progression. The stroma contributes growth factors and other signaling molecules to carcinoma cells, and altered ECM composition can change the biomechanical forces exerted on cancer cells, both of which contribute to disease progression.
- Anoikis is a specific form of apoptosis that is induced when cells no longer receive appropriate signals from the ECM. To survive in the stromal bed of the primary tissue that malignant cells migrate through and secondary metastatic sites, they undergo activating mutations in the ECM survival pathway.
Introduction
Cellular adhesion to the extracellular matrix and to neighboring cells orchestrates tissues into functional units, and is required for cell migration (Fig. 12.1). Adhesion also regulates cell shape, via the cytoskeleton and intracellular tension, and has a key role in determining the responses of signaling ligands to control cellular fate and differentiation, as well as survival and proliferation. Adhesion is mediated through specific classes of cell surface receptors, which join cells to each other or to the ECM. In cancer, altered adhesion influences each of the hallmarks of tumorigenesis, and is the defining characteristic of malignancy. Changes in cell–cell adhesion and cellular interactions with the ECM have severe consequences on the ability of extracellular factors to signal properly, so that cells lose their positional identity. Altered adhesion signaling results in the unscheduled migration of tumor cells, their dissemination through the circulation, and their embedment, survival, and proliferation at distant sites, thereby forming life-threatening metastases.
Figure 12.1 Tissue architecture. The key components of epithelial tissues shown as (a) a 3D representation of a pseudostratified epithelium, and (b) a schematic cross-section of a simple epithelium. They contain epithelial cells, separated from the stroma by a basement membrane; stromal cells such as fibroblasts and adipocytes; capillaries; neurons; and cells of the immune system. Although the main cell type to become altered genetically, and therefore neoplastic, is the epithelial cell itself, cancer progression depends on influences from other cell types. Thus, cancer is a disease where homeostasis of a whole organ is disrupted. It not only involves the cancer cells themselves, but also is characterized by disturbed stromal, endothelial, and immune cells, as well as altered ECM.
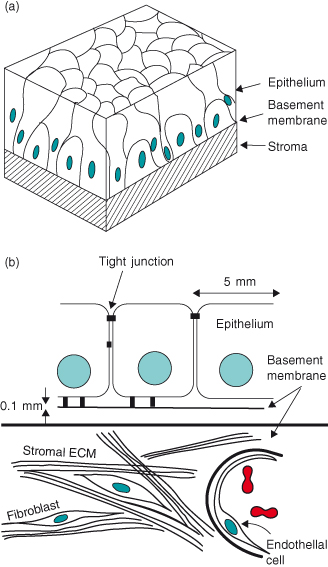
As discussed in other chapters, cancer is largely caused by genomic instability that arises within stem cells. It involves the escape of protective apoptosis and immune surveillance mechanisms, deregulated proliferative and aging controls, as well as inappropriate sampling of the environment so that tumor cells ignore normal positional cues and thereby migrate to and survive at distant metastatic sites (Box 12.1). This chapter is about how cells respond to their microenvironment, and how alterations in the way that cells perceive their neighbors and the ECM contribute to deregulated homeostasis, leading to the formation of benign tumors and subsequently malignant cancers that metastasize to distant organs.
- Benign tumor: a tumor that slowly grows at its site of origin and is well circumscribed. The cells within benign tumors frequently have normal morphology and are differentiated. These tumors do not normally cause the death of an organism, but sometimes can provide the seed cells for malignant tumors. Benign tumors can be large, but they are often operable.
- Malignant tumor: a rapidly spreading tumor that progresses through a number of stages. Initially they have diffusely infiltrative margins and can show differentiated characteristics. They evolve to poorly differentiated structures that sequentially invade basement membrane, stroma, and then vasculature. It is only once a tumor has spread that a person has contracted “cancer.”
- Metastasis: a tumor growing within another organ of the body. Although individual metastases are usually clonal in origin, they are often present in large numbers within any given organ, making them inoperable. The excessive tumor burden resulting from multiple metastases leads to the failure of essential body functions and death.
The most common cancers arise in epithelial tissues, such as the epidermis, lungs, intestine, prostate, or breast, and are called carcinomas. This chapter considers the types of adhesive interactions that epithelial cells normally encounter, how such interactions break down in cancer, and the mechanisms that allow cells to migrate to distant sites, and then survive and grow there. Many of the references are to contemporary review articles, which cover each topic in much more detail.
Adhesive Interactions with the Extracellular Matrix
There are two main types of adhesive interactions; those with the ECM and those between cells (Fig. 12.2). Normally, parenchymal cells (this includes epithelial cells) reside on a sheet-like ECM called the basement membrane (sometimes referred to as the basal lamina). This matrix is a continuous and flexible part of the basal surface of all epithelia, which is 40–120 nm thick and forms the interaction zone with stromal ECM. Basement membranes are also associated with endothelial cells lining capillaries and with muscle and Schwann cells. The stroma provides support for epithelia and is composed of the connective tissue matrix (synonymous with stromal ECM). Stromal cells, such as fibroblasts, myofibroblasts, adipocytes, endothelial cells and macrophages, are embedded within it, as are nerves. Blood and lymph vessels penetrate the stroma to provide nutrients and immune protection.
Figure 12.2 Key adhesive interactions of epithelial cells. (a) Several types of cell–cell adhesion systems bind adjacent cells together. Tight junctions form a ring around the apical surface of epithelia, and thereby ionically separate the extracellular spaces at the apical and basal surfaces. Adherens junctions connect cells via cadherins and organize the microfilaments, particularly toward the apical side of polarized cells. They also sequester the transcription factor β-catenin. Gap junctions provide ionic communication between adjacent cells. Desmosomes are large plaques that form strong intercellular bonds, again via cadherins. They are of key importance in maintaining tissue integrity under conditions of sheer stress. The basal surface of epithelial cells interacts with glycoproteins within the specialized ECM, the basement membrane, via both integrin receptors and transmembrane proteoglycan receptors (the latter are not shown). Integrins can be located either within multiprotein assemblies known as adhesion complexes, where they link to the actin cytoskeleton, or in very large adhesion plaques called hemidesmosomes, which link to the intermediate filament network, itself forming a bridge to the nuclear envelope. (b) In addition to binding cells to the ECM, integrin receptors regulate cell shape and polarity via the cytoskeleton, and they link to intracellular signaling pathways via structural adaptor proteins and signaling enzymes. Adherens junctions have a similar function in cytostructural regulation and signaling. Polarity: Most adhesive cells have an intracellular “direction,” so that different components become spatially segregated to different sides of the cell. Epithelial cells have a basal surface, which contacts the basement membrane, and an apical surface at the opposite side of the cell. Plasma membrane lipids are different on apical and basal surfaces. Similarly, the cytoplasmic contents are different toward apical and basal poles, and nuclei are usually located within the basal half.
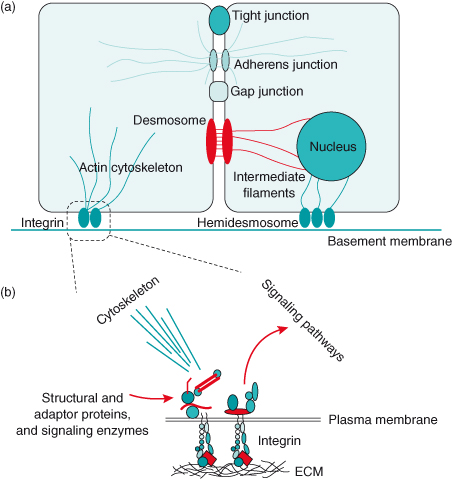
In cancer, epithelial cells come into contact with different types of ECM during their progression to malignancy. The basement membrane forms a boundary between epithelia and stroma in both normal tissue and premalignant lesions such as carcinoma in situ. In malignancy, the cells become invasive, breaking through the basement membrane and coming into contact with stromal or other ECM. Changes in epithelial cell-to-ECM interactions are required in order for the cells to do this.
Basement Membrane
The basement membrane provides essential signals for the morphogenesis of epithelial structures during development as well as the survival, proliferation, differentiation, and cytostructural architecture of cells within them. ECM molecules, together with the growth factors (GFs) they harbor, deliver these signals. A key class of basement membrane glycoproteins is the laminins, which are cross-shaped molecules of an α–β–γ composition. 5 α, 3 β, and 2 γ laminin genes exist, and their protein products assemble into at least 15 different heterotrimers. Some of the main laminin isoforms in epithelial basement membrane are laminin-1, laminin-5, and laminin-10. Laminin heterotrimers interact via their N-terminal head and arm domains to form a lattice-like network of interacting proteins. They bind to transmembrane cell surface integrin and proteoglycan receptors, resulting in organization of cell shape via the cytoskeleton, and triggering of intracellular signaling pathways.
An additional basement membrane protein is collagen type IV (collage-IV), which forms a self-interacting network that looks a bit like chicken wire. Although collagen-IV does bind surface receptors, its major function is to provide rigidity to the basement membrane. The laminin and collagen networks are linked together by nidogen, stabilizing the basement membrane. The fourth major component of basement membrane is perlecan, a large proteoglycan which binds a variety of growth and morphogenesis factors, and is involved with both sequestration of factors away from cells until they are released by proteases, as well as (in some cases) their presentation to appropriate surface receptors for signal transduction. Other minor components of the basement membrane include agrin, fibulin, and collagen types VII, XV, and XVIII.
Basement membranes are made through collaboration between epithelial and stromal cells, and are deposited to form a lamina at the interface between the two tissue compartments. Epithelia require direct signals from the basement membrane to function properly, indicating one way that the stroma influences how epithelia behave.
Basement Membrane Drives Polarity
The interaction of epithelial cells with the ECM at their basal surface leads to adhesion-regulated organization of the cytoskeleton and, together with a contribution from cell–cell contacts, to the establishment of cellular polarity (Fig. 12.2). ECM receptors control the orientation of epithelial polarity by causing the internalization of apical components and directing their trafficking to the opposite side of the cell.
The ability of ECM to regulate the cytostructure of individual cells also extends to the organization of multicellular structures. For example, the functional units of mammary glands are epithelial acini, which consist of a layer of polarized cells organized around a central lumen and with their apical surfaces facing inward (see, later in this chapter, Fig. 12.15b–c). Since epithelia in this tissue secrete milk, the cells have to be topologically orientated in this way for milk to be delivered into a discrete extracellular compartment. Modeling cancer accurately in experimental tissue culture depends upon appreciating the three-dimensional (3D) nature of tissues (Fig. 12.3).
Figure 12.3 Three-dimensional (3D) culture models for cancer. Although many aspects of cell behavior, including survival and proliferation, are frequently studied in monolayer culture, these often do not reflect the responses of cells in 3D arrays in vivo, and extreme caution has to be taken to interpret experimental data. Fortunately, culture models are now available which mimic the 3D organization of tissues, and better models are therefore being developed to study carcinoma progression. ErbB2 is a member of the EGF receptor family and is frequently upregulated in breast cancer. However, an activated ErbB2 does not transform normal breast epithelial cells, as assessed by conventional methods such as growth in soft agar. In this example, (a) breast cells plated in 3D basement membrane gels grow to form hollow acini surrounded by a collagen IV–containing basement membrane, which resemble mammary alveoli in vivo. Under the same culture conditions, (b) the activated ErbB2 now causes the cells to proliferate so that they fill the luminal spaces and it alters their properties so that they form multi-acinar structures. These resemble some forms of early breast cancer, such as comedo ductal carcinoma in situ (Figure 12.15d) (Debnath and Brugge, 2005; Weigelt and Bissell, 2008).
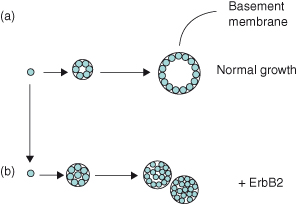
The ECM control on multicellularity and topology has important consequences for the response of cells to their local environment. For example, in a polarized epithelium, extracellular regulatory factors or cytokines that are supplied to one surface of an epithelium work only if their receptors are present on the same cell surface. The expression of basement membrane proteins is often reduced in malignant cancer, contributing to a loss of cell polarity and altered proliferation (Fig. 12.4).
Figure 12.4 Polarity segregates signaling ligands from their receptors and thereby controls proliferation. GF receptor signaling in simple epithelia can be regulated by polarized segregation of the ligand to a different plasma membrane compartment than the receptor. Airway epithelia express the receptor ErbB1-4 as well as one of its ligands, heregulin-α. (a) The ligand is expressed on the apical cell surface and is secreted into apical medium, while the receptor is present only basally and cannot be activated unless heregulin-α is added ectopically to the basal cell surface or if tight junctions in intact monolayers are broken. Wounding the epithelium causes rapid heregulin-α-activated ErbB2 signaling. In this way, segregation of ligand and receptor provides an elegant mechanism for rapid GF receptor activation and tissue repair after epithelial injury. (b) Unfortunately, this has dire consequences if cellular polarity is disrupted in disease processes, because it can lead to unscheduled proliferation. For example, increased epithelial permeability in smoking-associated bronchitis can disrupt growth homeostasis and therefore contribute to tumor formation (Vermeer et al., 2003).
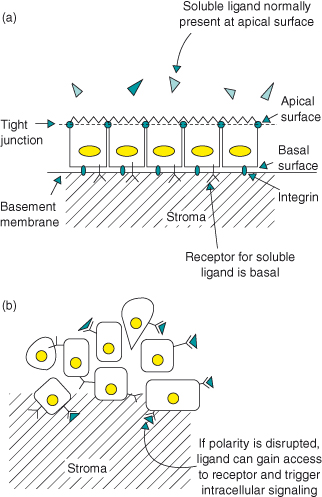
Basement Membrane Orchestrates Growth and Survival Signals
Integrin-mediated adhesion is essential for most cell types to respond to growth factors. Thus, in addition to organizing the cytoskeleton and cell shape, cell–matrix interactions in epithelial cells are crucial for orchestrating accurate responses to growth and survival factors. Receptors for basement membrane proteins can activate signaling pathways and are required in trans for many GF receptors and their downstream signaling enzymes to work properly, and thereby for normal cells to proliferate (discussed further in this chapter and Chapter 5). Integrins are also required in most normal cell types to protect them from apoptosis; death following altered or lost adhesion is a process called anoikis (see the “Anoikis” section of this chapter). This effectively means that basement membrane proteins provide essential contextual information for instructing the way that epithelial cells behave. Appropriate crosstalk between cell–ECM interactions and oncogenes is essential for tumor formation.
Stromal–Epithelial Interactions
The stroma has an essential supporting function for epithelia, contributing signals for epithelial morphogenesis and function, and providing a vital substratum at times of tissue repair. Stromal ECM also has certain key properties that are important for cancer progression. First, it is composed of different proteins to the basement membrane and it harbors soluble factors and cytokines. This means that the stromal ECM can contribute directly to cancer progression by providing growth factors.
Second, the stromal ECM stimulates epithelial cells coming in contact with it to behave quite differently from those contacting basement membrane, for example by changing their shape, and altering gene expression as well as proliferative and survival responses. Tumor stroma is often more crosslinked and denser than normal stromal ECM, so its physical characteristics change during cancer progression. This means that during the course of malignancy, when epithelial cancer cells invade stromal ECM, the inappropriate environment may affect cells in ways that contribute to tumor development.
Third, stromal ECM networks serve to impede the passage of cells that are not specially designed to migrate through it. An important characteristic of malignant tumor cells is that they acquire mechanisms to help them migrate through the stroma, including both phenotypic changes that activate the motile machinery and inappropriate expression of proteinases that degrade collagens.
Fibrillar Collagen
Stromal ECM is largely composed of fibrous proteins such as collagens and proteoglycans, which are proteins with large glycosaminoglycan (GAG) chains covalently bound to them. Collagen fibrils are made of helical bundles of collagen type I or III (in epithelial tissue stroma), to which are attached small collagens (e.g. collagen type IX) and proteoglycans (e.g. decorin). The triple-helical collagens assemble as bundles of fibrils that are organized into lattices, and through their high degree of mechanical strength they provide the tensile rigidity to maintain tissue form.
GAGs
GAGs are chains of repeating disaccharides, which can be up to several hundred sugar residues long, and are negatively charged due to sulphate groups. This allows GAGs to form gels, because of the mutual repulsion of negative charges and the entrapment of large numbers of water molecules, and thereby permits the stromal ECM to withstand compressive forces. Some GAG molecules are not covalently bound to a protein core and can occupy huge spaces; a single hyaluronan molecule, for example, may consist of up to 25 000 disaccharides and can form a 300 nm cube. Because of this property, hyaluronan can separate tissue components and thereby provide a space within dense stroma for cells to migrate. Hyaluronan also activates its cell surface receptor, CD44, which is a signal-transducing receptor for promoting migration. Although important for regulated tissue morphogenesis during development, altered hyaluronan expression and its interaction with CD44 occur in tumor cells and contribute to malignant progression.
Other Stromal Proteins
Other secreted glycoproteins, such as elastin and fibrillin, are also part of the stromal ECM, and contribute in their own way to the biology of the stroma; for example, the elastin fibers (made up of crosslinked networks of elastin molecules) provide resilience to tissues. Tissue fibronectin is also present throughout the stroma. This protein, which is a dimer, can provide adhesive links between collagen and cells, although cells also bind to some stromal ECM components directly. Fibronectin is one protein that cells rely on to migrate their way through stroma.
Tenascin is a further ECM protein that has a role in cancer progression. However, rather than being adhesive, its main function is anti-adhesive, because it interferes with integrin-dependent cell spreading. By antagonizing stable links between cells and the ECM, tenascin promotes cell migration. It is expressed during embryonic development when considerable cell movement occurs, and in the adult it is re-expressed in areas of tissue remodeling such as at wound repair sites. Tenascin is frequently upregulated in cancer.
Altered Stroma Contributes to Malignancy
Although carcinomas are epithelial in origin, they usually show a strong stromal reaction, reflected in disorganized composition and cellular content of the neighboring ECM. The gene expression profile of stromal cells adjacent to primary carcinomas is widely different from that of normal stromal cells, and many of these changes contribute directly to tumor progression. Importantly, genomic alterations are confined to carcinoma cells and absent from stromal cells. This means that the epithelial tumor cells have a dominant influence over the stroma, driving it to “assist” the cancer cell’s mission for dissemination. However, in some situations, the stroma can contribute directly toward the advancement of epithelial malignancy (Fig. 12.5).
Figure 12.5 The stroma contributes directly to epithelial malignancy. In this experiment, (a) the epithelium is removed from the mammary gland of a 3-week-old mouse, leaving the mammary fat pads which consist of only the stromal components. (b) Eight weeks later, the mice are irradiated on one side only, and mammary epithelial cells are transplanted into both irradiated and control mammary fat pads. After a further 8 weeks, the cells in the non-irradiated glands proliferate and undergo developmental morphogenesis to form a normal-appearing ductal outgrowth. The cells in the irradiated side also form ductal outgrowths, but a large number of tumors also develop. The mammary cells injected are normal apart from harboring mutations in both p53 alleles, indicating that they are genomically unstable. Thus, ionizing radiation, which is a known carcinogen, causes changes in the stroma, which then facilitates the expression of neoplasia within the epithelial cell compartment. This type of communication between stroma and epithelium is often overlooked, but is of key importance in cancer progression (Barcellos-Hoff and Ravani, 2000).
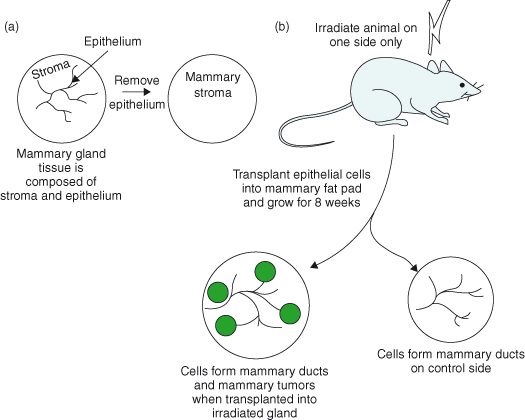
Stromal ECM Harbors Growth Factors
An additional feature of the stroma is that it harbors most of the locally acting paracrine factors that control epithelial cell function: transforming growth factor-β (TGF-β) binds to latency-associated peptide (LAP), fibroblast growth factors and Wnts are sequestered by proteoglycans, and insulin-like growth factors (IGFs) bind to IGF-binding proteins, which themselves are matrix bound (Chapter 5 covers growth factor signaling). GFs are released from the stroma by a variety of enzymes including ECM-degrading proteinases, which are inappropriately expressed in cancer cells. Chemokines such as CXCL12 are secreted by stromal cancer fibroblasts, and they also have a profound role in promoting tumor growth and the formation of secondary tumors.
Most of these proteins, and the ECM proteins themselves, are synthesized by stromal cells. Thus they have a strong controlling influence over the fate and function of epithelia. This has a key role in guiding the progression of epithelial malignancy, since cells within the stroma can be influenced by tumor cells to secrete factors that contribute to the invasive behavior of tumor cells themselves (Fig. 12.6).
Figure 12.6 Stromal–epithelial interactions in invasive behavior of tumor cells. Tumor cells can subvert the normal function of stromal cells so that they contribute toward malignant progression, even though they don’t become altered genetically. Here, tumor cells secrete TGF-β, which recruits myofibroblasts to differentiate from fibroblasts. These cells are also sometimes called cancer-associated fibroblasts (CAFs). CAFs have several effects on carcinoma cells that encourage them to migrate through the stroma. Firstly, they secrete HGF, which causes the carcinoma cells to undergo a phenotypic transition so that they take on features of migratory mesenchymal cells. Secondly, CAFs secrete tenascin into the stromal ECM: this is enhanced by TGF-β. As a consequence, the small GTPase Rac is activated within the tumor cells, while Rho is inhibited. These enzymes regulate the cytoskeleton so that the cells become more motile. Together, this epithelial–stromal–epithelial activation loop causes stationary carcinoma cells to become migratory (De Wever et al., 2004, 2008). CAFs also secrete SDF-1 (CXCL12), which acts on both endothelial cells and cancer cells through the CXCR4 receptor to promote tumor growth (Orimo et al., 2005).
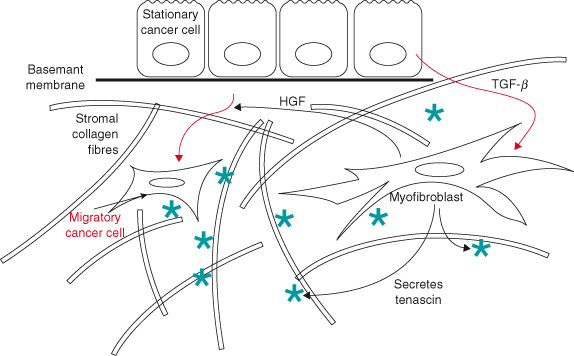
Integrins and Adhesomes
ECM proteins bind to cells through a variety of transmembrane cell surface receptors. The most prominent are integrins, which are α–β heterodimers (Fig. 12.7). Adhesion to ECM occurs at the distal ends of the heterodimeric receptors, where the α and β subunits interact. On the cytoplasmic side, integrins bind adaptor proteins that link them both to the actin-based cytoskeleton and to enzymes that trigger signal transduction cascades. Integrins therefore integrate the extracellular anchoring elements of the ECM with intracellular proteins of the cytoskeleton. Because the interaction with ECM proteins occurs with low affinity, numerous ligand–receptor bonds are required to form stable adhesion sites for cells. The result is that macromolecular assemblies, visible in the light microscope, build up at the plasma membrane. This is rather like molecular “Velcro,” where greater numbers of the same-sized bonds between two surfaces greatly increase the requirement for tensive forces to separate them. Large number of adaptor proteins, cytoskeletal elements, and enzymes are recruited to these sites, which are called adhesion complexes, or adhesomes (Fig. 12.8). Adhesomes are membrane-associated multifunctional machines, which are simultaneously sites of interaction between the cell and ECM, anchor points for the cytoskeleton, platforms for intracellular signaling, and the engines of cell migration.
Figure 12.7 Integrin heterodimers. There are at least 25 distinct integrin heterodimer pairs in total, made up from 18 α and 8 β subunits. Each is specific for a unique set of ligands. This diagram shows only a subset of the various ECM proteins bound by integrins. Some integrin heterodimers are promiscuous and bind several ligands, while several ECM proteins interact with cells through different integrins, depending on the cell type and differentiation state. Coll: collagen I, III, or IV; LM: laminins; FN: fibronectin; VN: vitronectin.
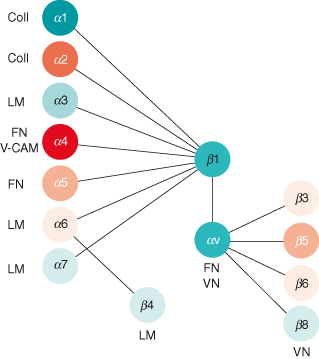
Integrin subunit composition frequently changes during the progression to malignancy, providing carcinoma cells with the machinery to survive and migrate in the interstitial ECM, which they do not normally come into contact with. It is well known that signaling enzymes are upregulated in many cancers, but this actually extends to adhesion-activated enzymes such as FAK and ILK.
Figure 12.8 Adhesion complexes are multiprotein assemblies at the cell–ECM interface. (a) The schematic diagram of the adhesion complex includes transmembrane integrins, which link cells to the matrix on the outside and organize the cytoskeleton and cell shape on the inside. Integrin ligation activates proximal cytoskeletal adaptor proteins, such as talin and vinculin; signaling enzymes, for example FAK, Src, and integrin-linked kinase (ILK); and signaling adaptors such as Pinch and parvin, paxillin, and 130Cas that couple to further downstream pathways. These include the Ras–Erk and PI3K–PKB axes, PKC and PLCγ, and small GTPases including Rho, Rac, and Cdc42. A large number of other proteins (not shown) are also within adhesion complexes. See Humphries et al. (2009) for proteomic analysis of adhesion complex components. Note that adhesion-regulated signaling enzymes have key roles in controlling many aspects of cell behavior, including migration, polarity, survival, proliferation, and differentiation. The fluorescence image is of cells cultured on a tissue culture dish. It shows the integrin adhesion complexes where the cell interacts with ECM (red), the actin cytoskeleton (green), and nuclei (blue). In this case, the cell has been stained with an antibody specific for the phosphorylated, active form of focal adhesion kinase (otherwise known as pp125FAK or FAK). Note that the skeletal components of the cell begin at the adhesion complexes (i.e. their “feet”). (b) Images of cells in 3D culture (Debnath and Brugge, 2005), also stained for p-FAK. Note the staining is located to the periphery of the “acinar” structures that form in 3D culture. In the fluorescence section, taken at the “top” of the ball of cells, are visible adhesion complexes similar to those that form in 2D culture.
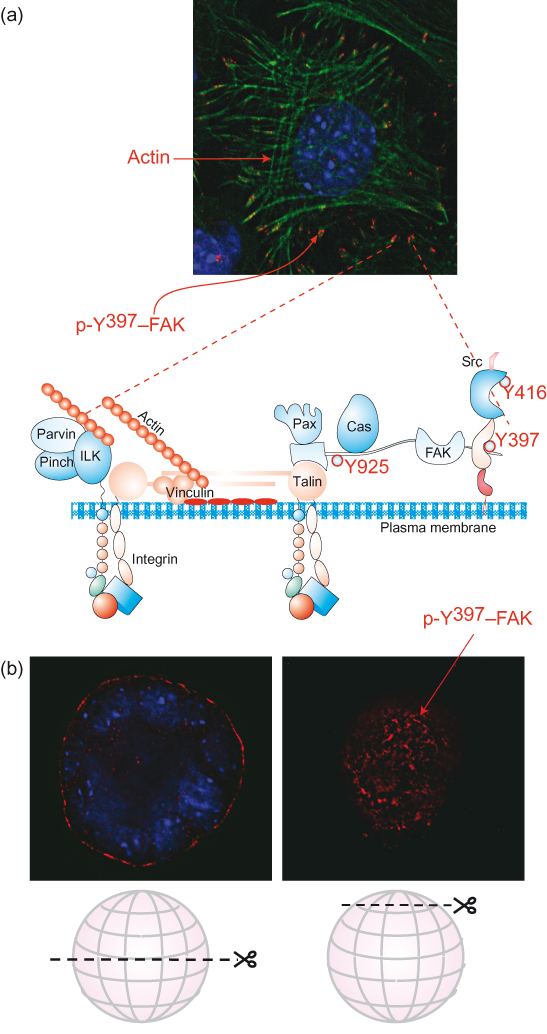
Integrin-containing adhesions exist in all cells, but some epithelia also contain a specialist type of assembly known as the hemi-desmosome. These are large structures that form strong bonds between epithelial cells and the underlying interstitial ECM through a chain of molecular interactions. Outside the cell, hemi-desmosomes link to the basement membrane protein laminin-5, via the signaling integrin, α6β4, together with a transmembrane collagen called BP180, or collagen type XVII. Inside the cell, rather than linking to the actin-based cytoskeleton, hemi-desmosomes contain adaptor proteins that bind to intermediate filaments. At their other ends, intermediate filaments bind to Nesprins, which directly link to Sun proteins and the structural laminins of nuclei. So hemi-desmosomes provide the ECM contact points that physically link the ECM with internal architectural proteins and nuclei. Hemi-desmosomal integrins are also altered in cancer, and for example are associated with advanced breast cancer.
Integrins Integrate Signaling Networks
One important function of integrins is to control the activity of intracellular signaling pathways, including members of the Ras family discussed in Chapters 5 and 6, and other small GTPases such as Rho and Rac. These pathways in turn regulate proliferation, motility, polarity, differentiation, and survival. Integrins can trigger those pathways to some degree independently of GFs, while in the absence of adhesion GFs do not activate them efficiently (sometimes they fail to activate signaling at all). This means that in normal, nontumor cells, integrins are context-dependent checkpoints for signaling: cells monitor their local extracellular environment and only permit GF and cytokine responses if they are in the right place.
Perturbations in the GF arm of this signaling network lead to oncogenesis. Mutations or epigenetic changes in the level or activity of integrin-regulated proteins also upset the homeostatic signaling balance, and altered adhesion thereby has a profound effect on cell growth. Integrins are essential for GF responses, but their signaling pathways can be hijacked deleteriously in cancer (Fig. 12.9). Integrin mutations are actually even more damaging because they additionally affect migration, and consequently metastasis.
Figure 12.9 Integrins are essential for tumor formation. (a) The normal mammary ductal tree consists of epithelial cells that form tubular arrays. In nonpregnant animals, these ductal trees remain stable. (b) If the polyoma middle-T antigen (MT) is activated in vivo, multifocal tumors form within a few weeks. This is because the middle-T antigen activates numerous signal transduction pathways that are normally associated with GF receptor signaling, such as those involving c-Src, phosphatidylinositol 3′-kinase (PI3K), and the Ras–Erk pathway. (c) If β1 integrin is deleted through conditional ablation of the gene, tumors do not form. This is most likely because the middle-T-driven signaling pathways need active integrins in order to function properly. Interestingly, the role of integrins varies among different cancer models. Thus, β1 integrin is dispensable for the growth of primary tumors in an ErbB2 animal model for breast cancer, but is required in order for those primary transformed cells to develop malignancies (Huck et al., 2010; White et al., 2004).
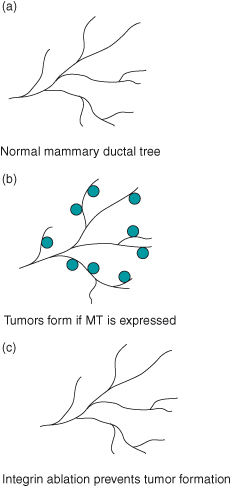
Transmembrane Proteoglycan Receptors for ECM
Some ECM proteins bind to other classes of transmembrane receptors that generate intracellular signals and are completely distinct from integrins. The important cell–regulatory ECM protein, laminin, binds to both a proteoglycan known as dystroglycan, as well as a lectin-like receptor β1,4-galactosyltransferase. Both of these receptors link laminin to the actin-based cytoskeleton as well as specific signaling enzymes. Dystroglycan has classically been studied in muscle, but has subsequently been found in epithelia. Both dystroglycan and galactosyltransferase have critical roles in regulating epithelial morphogenesis during development, and they are also involved with cellular polarity and differentiation. Dystroglycan is frequently lost in carcinoma progression, indicating a possible tumor suppressor role. Syndecan and glypican are additional proteoglycan adhesion receptors that have essential roles in epithelial cell function and neoplasia.
The Elasticity of the Cell Niche
Different types of tissues have differing degrees of elasticity, which profoundly affects the behavior of cells within them. There are soft tissues such as brain and adipose, and stiff tissues such as cartilage. Most epithelial organs are in between. The degree of softness, or compliance, is largely determined by the ECM composition, and is measured in Pascal units. ECM compliance is a key contributor to developmental processes because it determines lineage selection of stem cells and therefore tissue identity. For example, identical mesenchymal stem cells embedded into soft ECMs are specified to become adipocytes, while those in stiff ECMs take on chondrocyte cell fates.
The mechanisms for detecting ECM elasticity lie within adhesion systems (i.e. cell–cell and cell–ECM junctions) that sense forces within the cellular microenvironment, and convert them into intracellular chemical signaling pathways and reorganized cytoskeleton and actomyosin contractile machinery. For example, integrin adhesion complexes respond to sheer forces by triggering signaling enzymes (e.g. FAK), which in turn activate Rho GTPases and a downstream signaling pathway involving Rho kinase, myosin phosphatase, eventually leading to myosin contraction and the formation of f-actin bundles that create high levels of intracellular tension. One mechanism of force detection at the molecular level is through conformation changes of adhesion proteins. For example, the ECM protein fibronectin, integrins, and the intracellular adhesion complex proteins talin, vinculin, and p130Cas all expose cryptic binding sites for other proteins when they are stretched. It is not well understood how such tension determines cell fate. One possibility is that signaling through transcription factors, such as YAP, might be involved. Another is that the stretched cytoskeleton mechanically alters the shape of the nucleus, which might directly affect gene expression through epigenetic changes in chromatin architecture.
Extracellular forces applied to cancer cells have a significant effect on their behavior, and contribute to the progression of the disease. Breast cancer cells within stiff ECMs have more integrin clustering, activated adhesion complex proteins, and Rho-mediated cytoskeletal contraction whichis reflected in greater potential for migration. This is now recognized to be an important factor in cancer progression. A high mammographic breast density, which equates to more fibrillar collagen, is one of the greatest risk factors for developing cancer. In animal models, collagen-dense stroma promotes the initial formation of mammary tumors, as well as their progression to form metastases. Collagen crosslinking increases the stiffness of the stroma and enhances tumor formation. Moreover, higher actomyosin contractility causes tissues to become stiffer and promotes tumors (Fig. 12.10).
Figure 12.10 Tissue stiffness promotes cancer. (a) Two widely used animal models for breast cancer are the polyoma MT and the Neu–ErbB2 transgenic mice. In both cases, carcinomas arise sporadically from ductal epithelium. The ducts are surrounded by basement membrane and subtended by stromal ECM composed of collagen I and other ECM proteins, cells, and so on (see Fig. 12.1). Atomic force microscopy reveals that the stromal ECM is stiffer in the close vicinity of tumors than farther away, and there is increased collagen deposition and crosslinking, and higher levels of lysyl oxidase (Lox), a collagen crosslinking enzyme. Reducing crosslinking by using Lox inhibitors lowers the complexity of collagen crosslinking and tumor incidence. Conversely, increasing collagen crosslinking, either by using transgenic mice expressing collagenase-resistant collagen I, or by injecting glands with fibroblasts expressing excess Lox, promotes breast cancers. (b) Altering intracellular contractility also promotes cancer, and this occurs via changes in the stromal ECM. In a skin cancer model, genetically activating Rock alters the cellular microenvironment by increasing collagen crosslinking. The resulting extracellular forces impact back on the keratinocytes to increase proliferation in normal skin, and to enhance tumor formation in squamous skin cancer. The ECM forces most likely cause increased cell cycle by inducing integrin clustering and FAK activation, which promotes β-catenin nuclear localization and transcription of its targets. It is notable that the key players in both these models, Lox, collagen crosslinking, high Rock levels, and FAK and β-catenin activation, are all features of human carcinomas (Levental et al., 2009; Provenzano et al., 2008; Samuel et al., 2011).
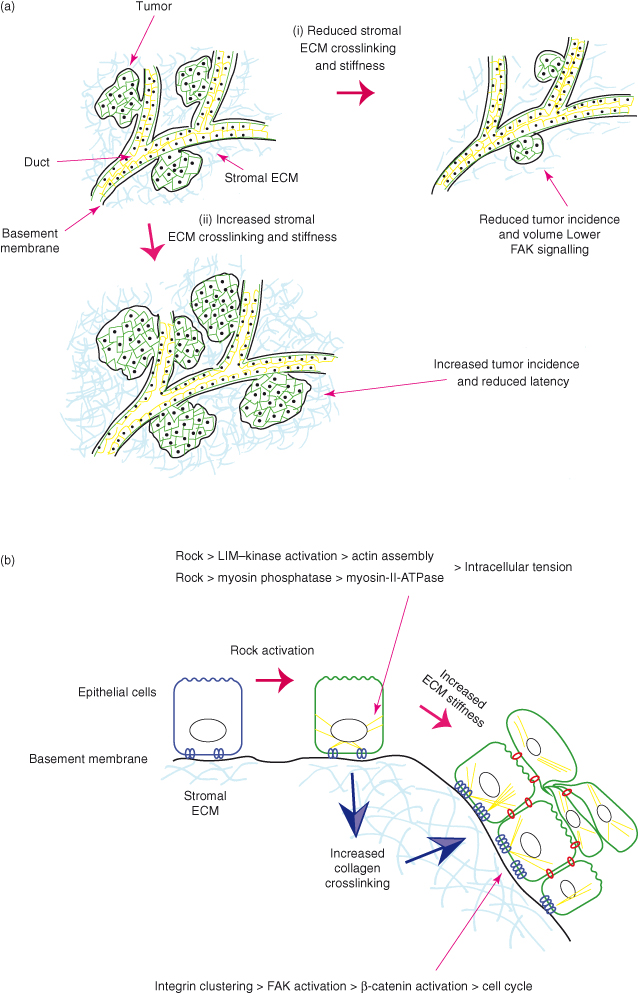
Together, this discussion so far highlights the central role of the cell microenvironment in cancer formation and progression. Carcinomas are characterized by mutations within the epithelial component of the tissue; however, they are much more than just diseases of mutated “tumor” cells. In order to form tumors, changes in the cell niche occur which are required for cancers to progress. Thus, it is not appropriate to view cancer as a disease simply of “cancer cells”; rather, it is a dysfunction of a whole organ in which stromal cells and the ECM play profound and essential parts.
Cell–Cell Interactions
Cell–cell interactions are critical for many of the cell processes elaborated in this book, including cell cycle and apoptosis. Cells interact and communicate directly with each other through a wide variety of mechanisms. Two different classes of cell–cell adhesive systems are involved. Multiprotein assemblies form the physical junctions between cells and provide contact points with the cytoskeleton. They are also sites of considerable signaling activity. These include adherens and tight junctions and desmosomes. Simple receptor–ligand pairs also have essential controlling roles at adhesive sites, and are involved with activating a variety of intracellular pathways with signaling nodes that include B-catenin, Erks, Smads, Nicd, Smoothened, and others.
Cadherins
Cell–cell adhesive junctions contribute to physically joining cells (Fig. 12.11). Two of these, adherens junctions (zonula adherens) and desmosomes, contain the most prominent cell–cell adhesion molecules, cadherins. Cadherins are transmembrane proteins that interact with each other both in trans and in cis. They bind to each other at their distal amino-terminal ends across the intercellular space, thereby connecting adjacent cells. They also form lateral interactions between neighboring molecules on the same cell. In this way, they form large multiprotein complexes that have sufficient numbers of adhesive interactions to hold cells together. These interactions are dependent on calcium cations. Although there are many cadherin species, their binding is usually homotypic.
Figure 12.11 Adhesion complexes at the cell–cell interface. (a–b) These fluorescence images of epithelial cell sheets show the localization of adhesion molecules distributed to cell–cell contact sites. (a) Cells stained for E-cadherin (green), which is visible in a continuous ring at the lateral junction between cells. (b) Cells stained for plakoglobin (γ-catenin), which localizes only to desmosomes and therefore has a punctate appearance. (c–d) Schematic diagrams of (c) an adherens junction and (d) a desmosome. Adherens junctions connect to, and organize, the actin-based cytoskeleton via the adaptor proteins α- and β-catenin. p120 is an important regulatory molecule, while β-catenin has a dual function as it is also a transcription factor. Desmosomes are strong spot welds between cells, holding them together. They link to the intermediate filament network and thereby couple the scaffolding between hemi-desmosomes and adjacent cells.
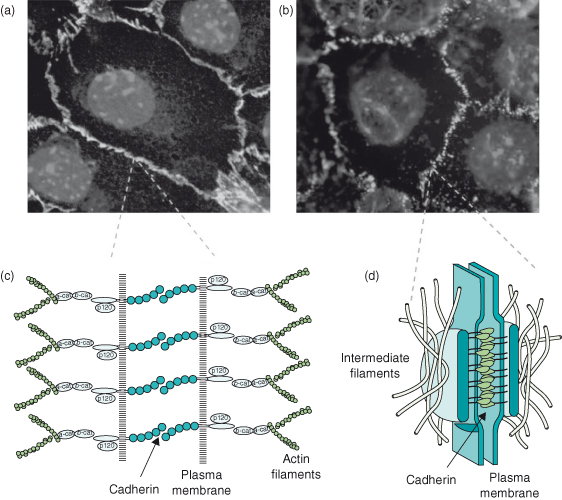
The prototype, epithelial (E)-cadherin, is the founder member of a small collection of “classical” cadherins, present in most epithelia in junctions that are visible in the electron microscope. The family is a very large one, and includes desmosomal cadherins and protocadherins that mediate, respectively, strong connections between epithelial cells and interneuron junctions in the central nervous system (CNS).
Cadherins play a key role in both developmental processes and tissue homeostasis, and their altered expression in malignant cancer plays an important part in the dissemination of cells and the resulting metastasis.
The β-Catenin–WNT Connection
The cytoplasmic face of an adherens junction contains adaptor proteins that connect to actin filaments, and also to signaling molecules. One of these is β-catenin, a transcription factor that can be sequestered by E-cadherin. β-catenin is not only of importance at adherens junctions, but also a transcription factor that is regulated by a developmental signaling cascade, the Wnt–β-catenin pathway.
β-catenin is not normally present as a free molecule within the cytosol because any that is not bound by E-cadherin at adherens junctions is targeted for degradation by a complex of three proteins which include a serine–threonine kinase called glycogen synthase-3β (GSK-3β) and the tumor suppressors axin and adenomatous polyposis coli (APC, originally identified in a type of hereditary colon cancer called “familial adenomatous polyposis,” in which its activity is lost – see Chapters 3 and 5). Wnt proteins are secreted signaling ligands whose distribution is spatially controlled by binding to proteoglycans in the ECM (Chapter 5). They stabilize β-catenin by signaling through the cell surface receptors comprising Frizzled and LRP5/6. The resulting intracellular pathway inhibits the activity of GSK3β, and so prevents β-catenin degradation. This leads to its nuclear translocation and interaction with a family of transcriptional repressors called Tcf–Lef. This interaction transiently switches Tcf–Lef from a transcriptional repressor to an activator, and thereby induces the transcription of numerous target genes including some involved in cell cycle regulation, apoptosis, and migration, such as c-Myc (Chapter 6).
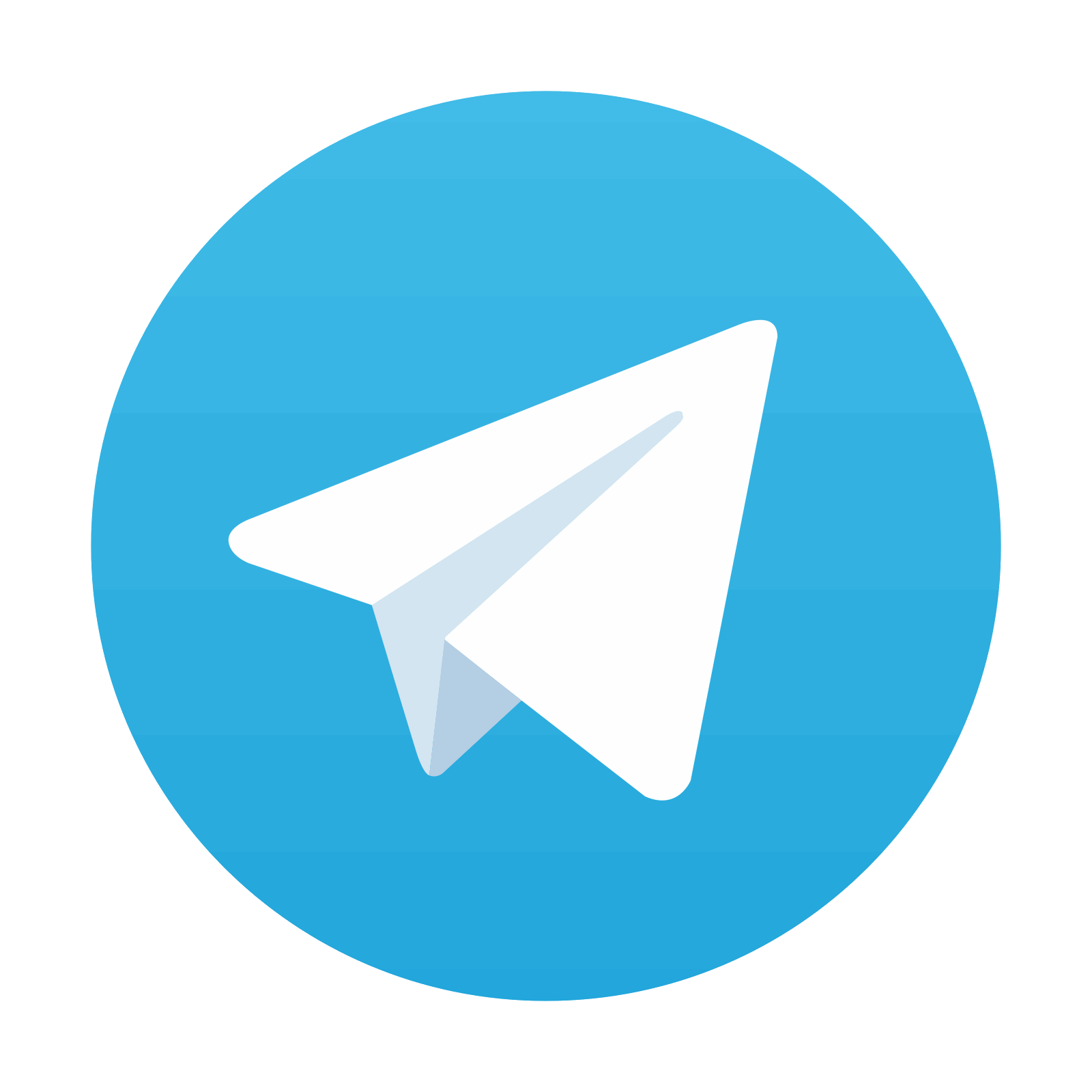
Stay updated, free articles. Join our Telegram channel

Full access? Get Clinical Tree
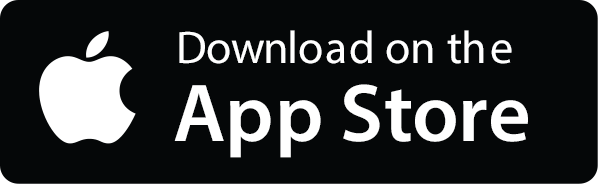
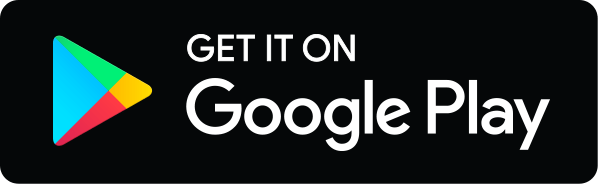