Cardiovascular Monitoring and Support
Irving L. Kron
Gorav Ailawadi
Introduction
Cardiovascular monitoring and support are essential to the care of surgical patients. The goals of cardiovascular monitoring are to ensure adequate tissue oxygenation and perfusion intraoperatively and postoperatively. Monitoring of the cardiovascular system involves an assessment of four major components: cardiac function, peripheral and pulmonary vascular tone, intravascular volume status, and oxygen metabolism. Accurate and thorough evaluation of these four components is a prerequisite to the proper application of cardiovascular support. Measurement of these parameters is accompanied with an increase in the cost, complexity, and risks associated with the use of these monitoring devices. Therefore, the decision to utilize these technologies must be determined based on the need and usefulness of the data compared to the risks and costs.
Table 1 Physical Signs of Organ-Specific and Systemic Perfusion | ||||||||||||
---|---|---|---|---|---|---|---|---|---|---|---|---|
|
Physical Findings
Cardiovascular monitoring involves a clinical assessment of organ-specific and systemic perfusion (Table 1). Physical signs are notoriously unreliable in estimating the adequacy of tissue perfusion, particularly in anesthetized or critically ill patients. Therefore, physical findings should be interpreted in the context of the patient and adjunct information obtained from more sophisticated monitoring techniques.
Electrocardiography
Continuous monitoring of the electrocardiogram (ECG) is the most sensitive, rapid, and cost-effective modality for detecting disturbances of cardiac rate, rhythm, and conduction. Such disturbances are common during general anesthesia, in critical illness, and especially in patients with a history of arrhythmias or coronary artery disease. In these settings, ECG monitoring should be routinely employed.
Another feature of continuous ECG monitoring, ST-segment monitoring, is quite reliable for the early detection of myocardial ischemia and infarction. Leads II and V5 are most commonly monitored, as these two leads together can detect >90% of intraoperative ischemic events in high-risk patients. Intraoperative ST-segment monitoring has become a standard practice in patients at high cardiac risk. Any electrical disturbances of the heart detected on continuous ECG monitoring (ST-segment changes, T-wave abnormalities, arrhythmias, etc.) should prompt a 12-lead ECG to confirm and further characterize the abnormality.
Blood Pressure Measurement
Blood pressure is the most commonly monitored cardiovascular parameter in current surgical practice. Blood pressure is prone to abrupt changes as a result of anesthesia, surgical procedures, changes in volume status and cardiac function, and underlying surgical illnesses. As arterial blood pressure is an indirect marker of systemic perfusion and a direct marker of hemodynamic status, diligent monitoring of this parameter is essential to early detection and treatment of cardiovascular instability.
Noninvasive Methods
In addition to continuous ECG, noninvasive blood pressure measurement is considered the standard intraoperative cardiovascular monitor for the majority of patients undergoing routine surgery. Noninvasive methods of arterial blood pressure determination should be considered indirect because no measurements are actually made with a device within the arterial lumen itself. Instead, such measurements are made with an inflatable cuff (sphygmomanometer), around the arm or leg and inflated to a pressure sufficient to compress the underlying artery. With the auscultatory method gradual cuff deflation permits the artery to reopen and thus produce Korotkoff sounds; systolic and diastolic blood pressure are determined by the pressure at which these sounds appear and then disappear, respectively, during cuff deflation. Alternatively, the oscillometric method relies on the principle of plethysmography, in which the pulsatile pressure changes in the underlying artery are sensed by the inflated cuff. The most popular automated oscillometric blood pressure device in clinical use is the Dinamap (GE Healthcare, UK), which is capable of measuring systolic, diastolic, and mean arterial pressures.
Although practical and noninvasive, these indirect techniques are limited by the frequency of measurements and can be inaccurate. For example, the use of an inappropriately small cuff in relation to the size of the limb (width of cuff <50% circumference) will yield a spuriously elevated blood pressure reading. Both methods can be limited in the setting of hypotension and the oscillometric devices have been little studied in the intensive care setting. Therefore, in cases of sustained or expected hemodynamic instability, noninvasive blood pressure monitoring techniques should be abandoned in favor of more accurate and reliable invasive methods.
Invasive Methods
Invasive blood pressure monitoring provides a direct and accurate assessment of arterial pressure. The advantage of this approach is the rapid detection in fluctuations in blood pressure and immediate feedback on interventions to correct hemodynamics. Furthermore, these methods provide a convenient source for frequent arterial blood sampling, both in the operating room and in mechanically ventilated patients in the intensive care unit. For these reasons, invasive blood pressure monitoring is recommended in patients that are or expected to become hemodynamically unstable, including those undergoing major abdominal, vascular, or cardiothoracic procedures. In addition, any major surgical operation in patients with a history of coronary artery disease, congestive heart failure, aortic stenosis, or poorly controlled hypertension are also an ideal setting for use of direct blood pressure measurement techniques.
Direct blood pressure measurement is performed with an indwelling intra-arterial catheter connected to fluid-filled high-pressure tubing and a transducer. The zero reference point for the transducer is at the level of the right atrium, which corresponds to the midaxillary line at the fourth intercostal space. If the transducer is positioned below the level of the right atrium, the resultant pressure will be spuriously elevated. Conversely, if the transducer is situated above the right atrium, the displayed blood pressure will be falsely low.
The contour of the arterial pressure waveform in the aorta differs from that in the peripheral arteries (Fig. 1). As the
propagating pressure wave migrates from the aortic root to the periphery, the systolic pressure gradually increases such that the peak systolic pressure in the radial artery can be 20 mm Hg higher than that in the proximal aorta. Thus, the propagation of blood into peripheral tissues is determined by the mean arterial pressure, not the systolic pressure. This increased systolic pressure in the distal arterial tree is due to less vascular elastic tissue and greater impedance, which is transmitted in a retrograde direction from vascular bifurcations and the artery–arteriolar junction. Such systolic amplification is especially prominent in more diseased, less compliant peripheral arteries, which is the physiologic basis for isolated systolic hypertension in the elderly. Amplification of peak systolic pressure in peripheral arteries is counterbalanced by a narrowing of the systolic waveform; the net result is that mean arterial pressure is unchanged. As a result, when peripherally placed intra-arterial catheters are employed, mean arterial pressure is the most accurate estimate of central arterial (i.e., aortic) pressure.
propagating pressure wave migrates from the aortic root to the periphery, the systolic pressure gradually increases such that the peak systolic pressure in the radial artery can be 20 mm Hg higher than that in the proximal aorta. Thus, the propagation of blood into peripheral tissues is determined by the mean arterial pressure, not the systolic pressure. This increased systolic pressure in the distal arterial tree is due to less vascular elastic tissue and greater impedance, which is transmitted in a retrograde direction from vascular bifurcations and the artery–arteriolar junction. Such systolic amplification is especially prominent in more diseased, less compliant peripheral arteries, which is the physiologic basis for isolated systolic hypertension in the elderly. Amplification of peak systolic pressure in peripheral arteries is counterbalanced by a narrowing of the systolic waveform; the net result is that mean arterial pressure is unchanged. As a result, when peripherally placed intra-arterial catheters are employed, mean arterial pressure is the most accurate estimate of central arterial (i.e., aortic) pressure.
Radial Artery Cannulation
The radial artery at the wrist is the most common site for insertion of an intra-arterial catheter. The advantages of this site are that the vessel is fairly superficial and easily palpable, it is of adequate diameter to accept a standard-size catheter (18- or 20-gauge), and the area is easy to keep clean. To facilitate cannulation of the radial artery, the wrist should be hyperextended to keep the thenar eminence out of the way and to bring the vessel to a more superficial location. Vessel entry is performed with a percutaneous Seldinger technique utilizing either a composite catheter-over-needle device or the standard seeker needle and guidewire. Successful vessel entry is heralded by a flash of arterial blood in the catheter hub. At this point, it is important to advance the needle another 0.5 to 1 mm to ensure that its beveled tip is entirely within the vessel lumen and not partially within its wall. The catheter or guidewire may then be safely advanced into the vessel. Historically, the Allen test has been used prior to radial arterial cannulation to evaluate ulnar artery supply to the hand; however, it is now generally believed that this test does not always predict ischemic complications from radial artery cannulation. Other potential sites for arterial cannulation include the femoral and axillary arteries, both of which have the advantages of central arterial access and a low thrombotic rate. The pedal arteries are less often utilized as a result of their small diameter and lower successful cannulation rates. The brachial artery should be avoided since this vessel has no collateral blood supply and thrombosis of it could yield severe forearm and hand ischemia.
Complications of Arterial Cannulation. The most common complication associated with all sites of arterial cannulation is thrombosis. The risk of this complication increases with the duration of cannulation; the risk of radial artery thrombosis may be as high as 29% in vessels cannulated for >4 days. However, the risk of clinically significant distal ischemia is <1%, as most thrombosed vessels tend to recanalize. Arterial catheter insertion sites should be routinely inspected for signs of ischemia, the presence of which mandates immediate catheter removal. Signs of arterial insufficiency distal to the site of catheter removal may persist for several hours as a result of vasospasm. However, ischemia persisting beyond 6 hours after catheter removal is an indication for surgical exploration with an eye toward thrombectomy and vessel repair. In addition to the duration of cannulation, other risk factors for catheter-induced arterial thrombosis include use of a larger catheter, insertion during shock, multiple needle passes and/or careless insertion technique, infusion of vasoconstricting agents, and a small recipient vessel. Other clinically important complications of arterial catheterization are infection, bleeding, and cerebral embolism. The incidence of local wound infection is 10% to 15% for radial artery catheters, but systemic infectious complications of such catheters are rare. Efforts to reduce the infection rate of radial artery catheterization include avoidance of a cutdown technique, replacement of the connector tubing every 48 hours, routine sterile dressing changes to the insertion site, and prompt removal of obviously infected catheters. However, in the absence of local or systemic infection, scheduled catheter changes are not necessary. Significant bleeding complications are most commonly associated with femoral and axillary artery lines, particularly in coagulopathic patients. For this reason, the radial artery is the preferred site of arterial catheterization in the presence of a bleeding diathesis. Although exceedingly rare, improper flushing of arterial lines has resulted in embolization of air, thrombi, and liquids to the cerebral circulation. This complication can be avoided by employing small-volume, low-pressure flushes and evacuating all air from the tubing and transducer assembly.
Pitfalls in Invasive Blood Pressure Monitoring
There are potential sources of error in the interpretation of data provided by invasive arterial pressure monitoring systems. As discussed above, erroneous blood pressure readings result from improper position of the transducer vis-à-vis the right atrium. In addition, the monitoring system is vulnerable to artifacts that distort the contour of the arterial waveform and display false blood pressure readings. An overdamped system is caused by a partial thrombus within the catheter, air bubbles within the tubing or transducer, or kinking of the catheter or tubing. The result is an attenuation of the peak systolic pressure and an overestimation of diastolic pressure, yielding a spuriously reduced pulse pressure. Conversely, underdamping or ringing occurs with extraordinarily long lengths of tubing and is characterized by augmentation of the peak systolic pressure and a blunting of the diastolic pressure, producing a falsely elevated pulse pressure. It is important to note that with either an overdamped or underdamped system, the mean arterial pressure is unaffected. Hence, if there is ever any doubt as to the fidelity of an invasive arterial pressure monitoring system, only the mean pressure readings should be considered reliable. Finally, it should be mentioned that in intensely vasoconstricted patients, such as those undergoing treatment with α1-agonists, invasive blood pressure readings via the radial artery may be significantly lower than central aortic pressure.
Central Venous Catheters
Cannulation of the central venous circulation allows rapid volume infusion through large-bore catheters, central administration of certain drugs (e.g., cardiotonic and vasoactive agents), infusion of parenteral nutrition, passage of pacing electrodes into the right heart, and monitoring of central venous pressure (CVP). Central venous catheters can be a useful adjunct for monitoring fluid status and helping to diagnose low urine output states. CVP, or right atrial pressure, is an estimate of right ventricular preload. Hence, a decreased CVP may indicate hypovolemia. An elevated CVP can occur in a variety of settings, including hypervolemia, increased intrathoracic pressure, positive pressure ventilation, pulmonary hypertension, right heart dysfunction, and left heart dysfunction. In a few conditions, the CVP can be unreliable for predicting left heart filling pressures. Normal CVP values range from 0 to 8 mm Hg and should be
measured at end-expiration in both the mechanically ventilated and spontaneously breathing patient. The normal CVP tracing has three positive waves (a, c, and v waves) and three negative deflections (x, y, and z waves, Fig. 2). These waveforms reflect tricuspid valvular function as well as right atrial and ventricular size, compliance, and contraction. Characteristic abnormalities of the central venous waveforms may be seen in a variety of conditions, including tricuspid stenosis and regurgitation, dysrhythmias, and atrial septal defect.
measured at end-expiration in both the mechanically ventilated and spontaneously breathing patient. The normal CVP tracing has three positive waves (a, c, and v waves) and three negative deflections (x, y, and z waves, Fig. 2). These waveforms reflect tricuspid valvular function as well as right atrial and ventricular size, compliance, and contraction. Characteristic abnormalities of the central venous waveforms may be seen in a variety of conditions, including tricuspid stenosis and regurgitation, dysrhythmias, and atrial septal defect.
Subclavian Vein Cannulation
All central venous cannulas should be placed with strict sterile technique including sterile preparation of the site, wide sterile draping to cover the patient and bed entirely, and requiring the operator to wear sterile gown and gloves and all personnel in the patient room with hat and masks. The infraclavicular approach to the subclavian vein is fairly consistent with regard to anatomic landmarks, the exit site is more comfortable to the patient, and indwelling catheters at this site have a lower infection rate than the internal jugular (IJ) approach. The subclavian vein originates lateral to the first rib (Fig. 3). During its course, it passes anterior to the first rib and directly posterior to the clavicle. The external landmarks for the site where the vein passes between the first rib and the clavicle are: (a) the junction between the medial and middle thirds of the clavicle and (b) the lateral edge of the sternocleidomastoid muscle where it inserts into the clavicle. The skin, subcutaneous tissues, and periosteum of this area just below the clavicle should be infiltrated with 1% lidocaine. The classic method for accessing the subclavian vein is the Seldinger technique. With the patient in the Trendelenburg position, the needle should be advanced just beneath the clavicle toward the suprasternal notch. To avoid causing a pneumothorax, it is very important to maintain a needle trajectory that is parallel to the floor. Return of free-flowing venous blood indicates entry into the lumen of the subclavian vein. At this point, the needle should be advanced a few millimeters to ensure that its beveled tip is completely within the vessel lumen. A J-tip guidewire is then carefully passed through the needle. If undue resistance is encountered upon passage of the wire, both the needle and the wire should be removed and the process repeated. If vessel puncture is unsuccessful with the technique described above, a more cephalad direction of the needle may prove successful; occasionally, the subclavian vein is displaced superiorly by the apex of the lung, particularly in emphysematous patients. After the wire has been confidently
advanced into the central venous circulation, the needle is removed, the skin exit site is enlarged with a scalpel blade, and a vascular dilator is passed over the wire. Great care should be taken during dilator passage, as the dilator is quite stiff and can easily perforate a central vein if forcefully advanced. It is best to dilate slowly and progressively, frequently ensuring that the wire moves freely within the dilator and is not being bent as it is forced against the wall of a central vein. It is critical to maintain control of the guidewire especially during maneuvers to advance the dilator and the catheter. Once the subcutaneous tract and the hole into the vessel are dilated, the catheter is threaded over the guidewire; the latter can then be removed. For most types of indwelling central venous catheters, the catheter tip should be situated at the junction of the superior vena cava and right atrium. Table 2 is a useful guide for estimating the distance to this junction from the skin exit site for each of the subclavian and IJ venous approaches.
advanced into the central venous circulation, the needle is removed, the skin exit site is enlarged with a scalpel blade, and a vascular dilator is passed over the wire. Great care should be taken during dilator passage, as the dilator is quite stiff and can easily perforate a central vein if forcefully advanced. It is best to dilate slowly and progressively, frequently ensuring that the wire moves freely within the dilator and is not being bent as it is forced against the wall of a central vein. It is critical to maintain control of the guidewire especially during maneuvers to advance the dilator and the catheter. Once the subcutaneous tract and the hole into the vessel are dilated, the catheter is threaded over the guidewire; the latter can then be removed. For most types of indwelling central venous catheters, the catheter tip should be situated at the junction of the superior vena cava and right atrium. Table 2 is a useful guide for estimating the distance to this junction from the skin exit site for each of the subclavian and IJ venous approaches.
Table 2 Approximate Distance from Skin Exit Site to Junction of Superior Vena Cava and Right Atrium (to Facilitate Correct Positioning of Central Venous Catheters) | ||||||||||||
---|---|---|---|---|---|---|---|---|---|---|---|---|
|
Internal Jugular Vein Cannulation
The IJ vein is an alternative to the subclavian vein for central venous cannulation. The advantages of this site over the subclavian approach are a lower incidence of thrombosis, a substantially decreased risk of pneumothorax, and, especially on the right side, a straighter course to the right atrium. Shortcomings of the IJ approach are that it is more uncomfortable for the patient, carries the risk of carotid artery puncture, and has a higher reported infection rate due to its proximity to aerodigestive secretions. Perhaps the most significant disadvantage of the IJ approach is that the vessel is less consistent in its anatomic relationships, thus making it somewhat more of a “blind” procedure. In approximately 70% of individuals, the IJ lies anterolateral to the carotid pulse at the base of the neck (Fig. 3). However, one should be aware that not infrequently this vein may lie directly anterior or even deep to the carotid artery, thus increasing the risk of inadvertently puncturing the latter. With the patient in the Trendelenburg position and his/her head rotated to the contralateral side, the triangle formed by the two heads of the sternocleidomastoid muscle is identified. A needle is inserted at the apex of this triangle, keeping the carotid pulse medial to the course of the needle. The needle is slowly advanced toward the ipsilateral nipple at a 45-degree angle to the skin. If venous blood is not obtained after one pass of the needle to 5 cm, the needle should be withdrawn and oriented in a slightly more medially. Once the operator is certain of needle entry into the lumen of the IJ (e.g., return of deoxygenated blood without arterial pulsations), Seldinger technique is used to insert the catheter.
Femoral Vein Cannulation
The femoral vein can be cannulated rather easily and expediently, and in select circumstances is the preferred route for central venous access. Examples include victims of burns to both sides of the neck and upper chest, trauma patients encumbered with cervical spine immobilization devices, and certain patients with acquired obstruction or congenital anatomic anomalies of the superior vena cava. The disadvantages of the femoral approach are a 10% risk of venous thrombosis, the need for the patient to remain supine and immobile, and a higher risk of catheter infection. The femoral vein is the most medial structure in the femoral sheath, lying just medial to the femoral artery. Puncture of the femoral vein is performed by first palpating the arterial pulse 2 cm below the inguinal ligament. At a point 1 to 2 cm medial to the femoral pulse, the needle is advanced at a 45-degree angle through the skin until venous blood is encountered. If the femoral pulse is nonpalpable, the femoral vein can be located at a point 1 to 2 cm medial to the junction of the medial and middle thirds of the inguinal ligament.
Complications of Central Venous Cannulation
In general, the complications of central venous catheter insertion are either mechanical or infectious. Complications ascribed to both the subclavian and IJ approaches include venous thrombosis, transient cardiac arrhythmias from ventricular irritation by the catheter or guidewire, cardiac or central vein perforation, thoracic duct injury, and embolization of catheter or guidewire fragments. With regard to the latter, these objects can usually be retrieved through a second catheter, but rarely require a sternotomy. A potentially fatal complication of central venous catheterization is venous air embolism. The risk of this dreaded complication is increased in patients with a low CVP, in whom the pressure inside the vein may become lower than atmospheric pressure during spontaneous inspiration. Air may enter the central venous system through defects in the catheter, the uncapped port(s) of the catheter, and the uncovered end of the needle during vessel puncture. The clinical scenario may be one of acute dyspnea and hypoxia, rapidly progressing to hypotension and cardiac arrest. If venous air embolism is suspected, the patient should be immediately placed in the left lateral decubitus and Trendelenburg positions, and an attempt made to withdraw air through the central venous catheter. Although pneumothorax can occur after either subclavian or IJ catheterization, the risk is higher via the subclavian approach. Inadvertent subclavian artery puncture is also a risk of the subclavian approach; however, unless the patient is coagulopathic, immediate withdrawal of the needle and 5 to 10 minutes of digital pressure is sufficient treatment. The most common complication of IJ catheterization is carotid artery puncture. If the carotid artery is entered with the probe needle, it should be promptly removed and direct pressure maintained on the area for 10 minutes. If it is apparent that the carotid artery has been cannulated with the dilator or catheter, these devices should not be removed blindly, particularly in an anticoagulated patient. To do so could produce serious airway-threatening hemorrhage. It may be safer to remove the dilator or catheter via direct surgical exposure, followed by suture repair of the artery. Methods to remove carotid artery catheters using arterial closure devices or covered stent grafts have been described. Other complications unique to IJ catheterization include Horner syndrome, brachial plexus injury, and phrenic nerve palsy.
The topic of indwelling central venous catheter infections deserves special mention, since this is an all-too-common and clinically important issue. Catheter-related infections are second to pneumonia as causes of nosocomial septicemia in critically ill patients, and such infections contribute significantly to morbidity, mortality, and length of hospital stay. Risk factors for catheter infections include immunosuppression, multilumen catheters, elderly
patients, insertion under emergent conditions or cutdown technique, and long duration of catheter in the same site. It is believed that the skin insertion site is the dominant source of the microorganisms causing catheter-related infections. Organisms migrate from the skin insertion site along the fibrin peel that forms around the catheter, eventually colonizing the intravascular catheter tip. A catheter-related infection is diagnosed by semiquantitative culture when 15 or more colonies of the same pathogen grow from the catheter tip. There may or may not be accompanying signs of local infection (e.g., erythema, purulence, tenderness, or warmth at skin insertion site) or systemic inflammation (e.g., fever or leukocytosis). Catheter-related infection is a prelude to catheter-related septicemia, which is defined as growth of the same organism from a peripheral blood culture and the catheter tip.
patients, insertion under emergent conditions or cutdown technique, and long duration of catheter in the same site. It is believed that the skin insertion site is the dominant source of the microorganisms causing catheter-related infections. Organisms migrate from the skin insertion site along the fibrin peel that forms around the catheter, eventually colonizing the intravascular catheter tip. A catheter-related infection is diagnosed by semiquantitative culture when 15 or more colonies of the same pathogen grow from the catheter tip. There may or may not be accompanying signs of local infection (e.g., erythema, purulence, tenderness, or warmth at skin insertion site) or systemic inflammation (e.g., fever or leukocytosis). Catheter-related infection is a prelude to catheter-related septicemia, which is defined as growth of the same organism from a peripheral blood culture and the catheter tip.
Replacing central venous catheters at regularly scheduled intervals has not been shown to decrease the risk of catheter-related infections. However, the following guidelines are recommended: (a) If there are clear signs of infection at the skin exit site, the catheter should be promptly removed and, if clinically indicated, a new catheter should be inserted at a fresh site; (b) when a catheter-related infection is suspected (with or without signs of local exit site infection), the catheter should be exchanged over a guidewire; (c) if the tip of any catheter replaced via guidewire exchange grows >15 colonies of a pathogen in semiquantitative culture, the existing catheter should be removed and replaced at a new site; and (d) any catheter placed emergently and without strict sterile technique should undergo guidewire exchange.
Pulmonary Artery Catheters
In 1970, Swan and colleagues introduced the concept of bedside right heart catheterization via manipulation of a flexible balloon-tipped catheter into the pulmonary artery (PA). Despite being used for nearly three decades as a monitor for the cardiovascular assessment and management of critically ill patients, there has been a great deal of recent controversy over whether the inherent risks and expense of using a PA catheter are justified by the clinical benefits of the information it provides. Unfortunately, many previous studies that questioned its use suffer from methodological flaws and/or failure to include surgical patients. Notwithstanding such controversy, this device remains a valuable tool in the care of critically ill surgical patients. General indications for use of the PA catheter include characterization and management of shock states and perioperative monitoring of patients at high risk for hemodynamic instability. With specific regard to the latter, examples of such patients include those with significant cardiovascular disease, advanced age, and significant burns or trauma, as well as those undergoing a surgical procedure associated with increased risk of hemodynamic disturbances. Clearly, in such patients clinical evaluation alone is inaccurate. Furthermore, as cardiac function can be impaired for a variety of reasons in critically ill surgical patients, a central venous catheter is an unreliable surrogate for estimating left-sided filling pressures in such individuals.
Pulmonary Artery Catheter Features
The PA catheter is 100 to 110 cm in length and usually has three lumens: a distal lumen, which opens at the catheter tip into the PA for measurement of PA pressures, PA wedge pressure (PAWP), and cardiac output; a proximal lumen 30 cm from the tip, which communicates with the right atrium and is used for measurement of CVP; and a third lumen approximately 15 cm from the tip, which can be used for infusion. In addition, the basic catheter is equipped with a temperature-sensing thermistor a few centimeters proximal to the catheter tip, as well as a 1.5-mL balloon surrounding the tip. Newer PA catheters are able to provide continuous monitoring of cardiac output, measurement of right ventricular ejection fraction (RVEF), and continuous assessment of mixed venous oxygen saturation (Svo2).
Pulmonary Artery Catheter Insertion
Prior to insertion, the balloon should be tested for defects by inflating it with 1.5 mL of air, all lumens should be flushed with sterile saline, and the distal port connected to a pressure transducer and an oscilloscope monitor. As described for invasive blood pressure monitoring, the pressure transducer must be zeroed at the level of the right atrium. The PA catheter is first placed through a sterile sheath to facilitate future manipulations, and is then inserted through a large-bore (7 or 8 French) introducer in the subclavian or IJ vein. If necessary, a femoral vein approach may also be used. During insertion, pressure waveforms are monitored continuously via the distal port to identify the intravascular location of the catheter tip (Fig. 4). With the balloon fully deflated, the catheter should be inserted to a distance of 15 cm, where the CVP tracing of the superior vena cava or right atrium appears. At this point, the balloon is slowly inflated with 1.5 mL of air and the catheter is advanced into the right ventricle. The characteristic right ventricular waveform is normally one of a pulsatile systolic pressure of 15 to 30 mm Hg and a diastolic pressure equal to CVP. With further advancement, the catheter should reach the PA, as identified by an abrupt increase in diastolic pressure while systolic pressure remains unchanged. As the catheter is manipulated farther into the pulmonary arterial system, the systolic waveform disappears and gives way to the characteristic pulmonary artery wedge (PAW) tracing. Under normal circumstances, the PAWP is similar to the PA diastolic pressure (6 to 12 mm Hg). With the appearance of the PAW tracing, the balloon is deflated,
whereupon the pulsatile PA waveform should reappear. If not, the catheter may be advanced too far into a PA branch (i.e., “overwedged”) and should be withdrawn slightly with the balloon deflated. When the tip of the catheter is in the proper position, the inflated balloon obstructs antegrade flow through the PA branch in which it is lodged. This creates a continuous stationary blood column from the catheter tip to the left atrium, such that the pressure measured through the distal port should be equivalent to left atrial pressure. Since under normal circumstances left atrial pressure is equivalent to left ventricular end-diastolic pressure (LVEDP), PAWP is used as an estimate of LVEDP (i.e., left ventricular preload).
whereupon the pulsatile PA waveform should reappear. If not, the catheter may be advanced too far into a PA branch (i.e., “overwedged”) and should be withdrawn slightly with the balloon deflated. When the tip of the catheter is in the proper position, the inflated balloon obstructs antegrade flow through the PA branch in which it is lodged. This creates a continuous stationary blood column from the catheter tip to the left atrium, such that the pressure measured through the distal port should be equivalent to left atrial pressure. Since under normal circumstances left atrial pressure is equivalent to left ventricular end-diastolic pressure (LVEDP), PAWP is used as an estimate of LVEDP (i.e., left ventricular preload).
Pitfalls in Pulmonary Artery Catheter Insertion
A potential difficulty encountered during PA catheter insertion is failure of the catheter to advance into the PA. This is caused by coiling of the catheter in the right ventricle, and is most often a result of the catheter being advanced too rapidly and forcefully. A simple and often successful solution to this problem is to advance the catheter more slowly and continuously, avoiding thrusting. This technique takes full advantage of the balloon-flotation characteristics of the PA catheter, allowing it to float gently with the stream of blood across the pulmonary valve.
Another common problem of PA catheterization is failure to obtain a PAW tracing. Although, in most cases, the reason for this is uncertain, it may be due to a faulty balloon or eccentric inflation. After several unsuccessful attempts to obtain a PAW waveform, the catheter should be removed and the balloon retested. If the balloon is not the source of the problem, there are two options. First, if the patient does not have known pulmonary hypertension, the PA diastolic pressure can be monitored as an estimate of PAWP. However, in cases in which the presence of pulmonary hypertension confounds the use of PA diastolic pressure as a surrogate of PAWP and it is essential to patient management that PAWP be determined, the authors have had success utilizing portable fluoroscopic guidance to manipulate the catheter into the wedge position.
Pitfalls in Interpretation of Pulmonary Artery Wedge Pressure
There are several sources of potential error in interpreting PAWP (and CVP). As mentioned in the section on invasive arterial pressure monitoring, the transducer must remain at the level of the right atrium for pressure measurements to be valid. This is especially important in measuring right heart pressures, as the magnitude of the resultant error in the pressure reading due to transducer malposition is greatest in low-pressure systems.
A source of error in correlating PAWP with left ventricular (LV) preload involves changes in intrathoracic pressure. Pressures measured by the PA catheter are intravascular pressures, whereas the most accurate estimate of actual LV filling pressure is transmural pressure. Transmural pressure is equal to the difference between intravascular pressure and intrathoracic pressure. At end-expiration intrathoracic pressure is normally equivalent to atmospheric pressure and thus considered negligible. For this reason, PAWP should only be determined at end expiration for both spontaneously breathing and mechanically ventilated patients (Fig. 5). However, in certain pathologic states (e.g., adult respiratory distress syndrome, high positive-pressure ventilatory settings, and tension pneumothorax), intrathoracic pressure may be significantly increased and produce a spurious increase in intravascular pressure (i.e., PAWP). In this setting, the increased PAWP does not reflect a true increase in LV preload, since the physiologically more accurate transmural pressure remains unchanged.
Another situation in which PAWP does not accurately reflect LV preload occurs when the tip of the PA catheter is improperly positioned within the lung. Three physiologic lung zones have been described based on pulmonary arterial, alveolar, and venous pressures. Alveolar pressure exceeds both arterial and venous pressures in zone 1, and exceeds venous pressure in zone 2. As a result, in zones 1 and 2 PAWP is actually more a reflection of airway pressure than left atrial pressure. Only in zone 3 PAWP is accurately reflective of left atrial pressure and not subject to the confounding effects of alveolar pressure. In the supine patient, zone 3 represents the region of the lung posterior to the left atrium, which is the most dependent area of the lung. Since zone 3 has the greatest blood flow, the air-filled balloon tip of the PA catheter will most often float into this zone. However, clues that the PA catheter tip is not positioned within zone 3 are: (a) the presence of marked respiratory variation on the PAW tracing; (b) if the positive end-expiratory pressure (PEEP) is increased and the PAWP increases by 50% or more of the increased PEEP; and (c) if the PAWP is greater than the pulmonary artery diastolic pressure (PADP). The presence of any of these criteria should prompt repositioning of the catheter. It is important to note that any condition that decreases pulmonary vascular pressure (e.g., hypovolemia) or increases alveolar pressure (e.g., high PEEP) can reduce the total area of physiologic zone 3 in the lung, even if the catheter tip is positioned posterior to the plane of the left atrium.
Mitral stenosis creates another pitfall in correlating PAWP with LV preload. In this condition, there is a pressure gradient between the left atrium and ventricle, such that left atrial pressure is higher than LV end-diastolic pressure. Therefore, although PAWP does reflect left atrial pressure in mitral stenosis, it cannot be used as an accurate predictor of LV preload in this condition.
In the presence of normal LV compliance, PAWP provides an accurate estimation of LV end-diastolic volume and thus preload. However, in conditions associated with decreased LV compliance (e.g., ventricular hypertrophy and myocardial ischemia), PAWP may be high even in the face of a normal or even decreased preload. Hence, PAWP is not a reliable index of LV preload in the setting of a poorly compliant ventricle.
The physician who uses a PA catheter to assist in the management of critical illness should bear in mind the above-mentioned shortcomings of PAWP in estimating LV preload. In situations in which PAWP is an unreliable index of left heart filling pressures, there are other modalities currently available to estimate LV preload. These
alternative modalities, such as transesophageal echocardiography (TEE) or RVEF obtained from the newer fast-response PA catheters, are discussed in detail below. With these concerns of causing an injury to the PA during wedging of the balloon tip and due to uncertain accuracy in certain cases, many intensivists do not interrogate wedge pressures routinely and simply follow the PADP in critically ill patients.
alternative modalities, such as transesophageal echocardiography (TEE) or RVEF obtained from the newer fast-response PA catheters, are discussed in detail below. With these concerns of causing an injury to the PA during wedging of the balloon tip and due to uncertain accuracy in certain cases, many intensivists do not interrogate wedge pressures routinely and simply follow the PADP in critically ill patients.
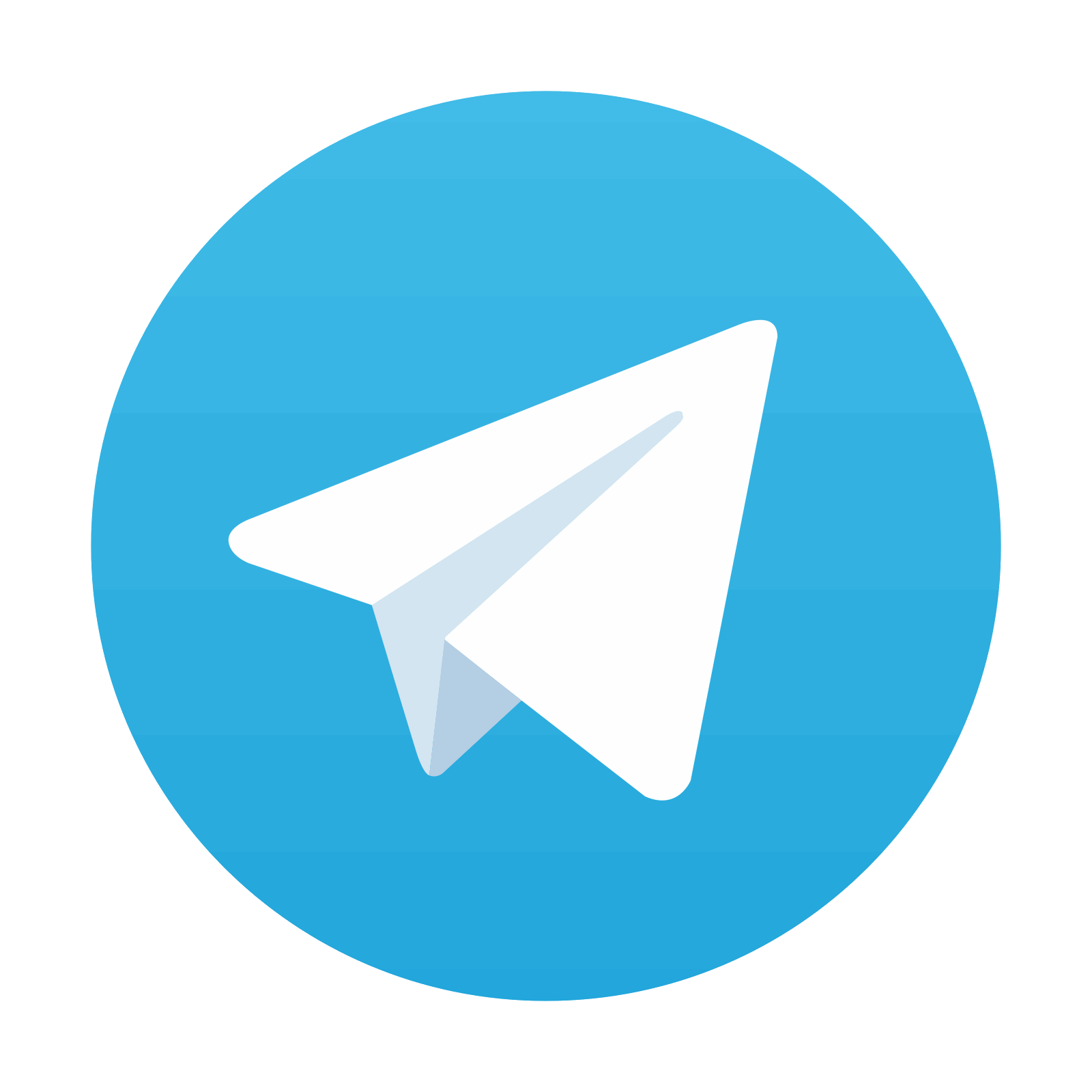
Stay updated, free articles. Join our Telegram channel

Full access? Get Clinical Tree
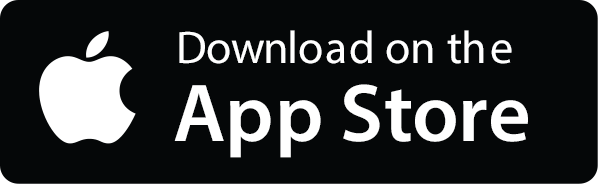
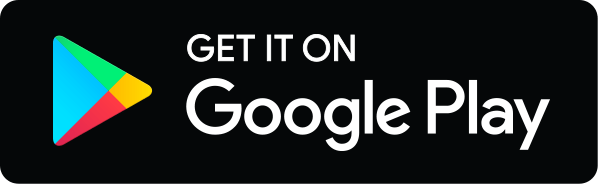
