Capsule and lipopolysaccharide
Lisa M. Willis and Chris Whitfield, University of Guelph, Guelph, ON, Canada
Introduction
Pathogenic E. coli require many different virulence factors which allow them to invade the host, evade host immune defenses and colonize specific niches in the host where they can cause disease. The first interactions between E. coli and its host occur at the outer membrane and are mediated by proteins and carbohydrate-containing macromolecules (glycoconjugates) on the bacterial and host cell surfaces (Figure 17.1). Bacterial glycoconjugates provide crucial defenses against different elements of the innate and acquired immune system, as well as generating tremendous diversity in surface antigenicity. In most Gram-negative bacteria, the outer membrane is composed predominantly of the glycolipid known as lipopolysaccharide (LPS). In E. coli, this complex molecule is composed of three structurally distinct regions; the hydrophobic anchor called lipid A, a core oligosaccharide, and the long-chain polysaccharide called O antigen (or O-polysaccharide; O-PS). The differences in E. coli O-PS structures give rise to more than 180 distinct O antigens and these have been exploited in serotyping classification methods (Orskov et al., 1977). Many E. coli isolates also produce another long-chain polysaccharide, known as capsular polysaccharide (CPS). CPS provides another major surface antigen, called the K-antigen, named after the German term ‘kapsel’. There are more than 80 different K antigens in E. coli. The means of attachment of CPS to the cell surface is not known in all cases but these polymers create a coherent structural entity (the capsule) that is visible by light microscopy and extends 50–100 nm from the cell surface. As a result, the capsule often masks underlying O antigens in serotyping studies that exploit specific antisera and whole-cell agglutination methods. A single E. coli isolate can produce one O- and one K-antigen.
Together, LPS and CPS represent major virulence factors, which have been the target of numerous vaccines and therapies, and they provide the focus of this chapter. However, they are not the only glycoconjugates produced by E. coli. All isolates produce a polysaccharide called enterobacterial common antigen that provides resistance to organic acids in E. coli (Barua et al., 2002) and to bile salts and detergents in Salmonella (Ramos-Morales et al., 2003). Under certain stress conditions (e.g. perturbations of cell envelope integrity), some E. coli isolates also produce an exopolysaccharide called colanic acid which has a biosynthetic pathway that is part of the Rcs-regulon (Majdalani and Gottesman, 2005). Unlike CPS, colanic acid is poorly retained at the cell surface. Like several other bacterial species, the biofilm mode of growth in E. coli is supported by formation of bacterial cellulose and a by-polymer of N-acetylglucosamine (PNAG) (Cerca and Jefferson, 2008; Saldana et al., 2009). However, some commensal E. coli isolates also produce a polysaccharide of unknown structure that impairs biofilm formation by other bacteria including Staphylococcus aureus, potentially affecting community dynamics (Rendueles et al., 2011). Similar anti-adhesion properties have been reported for certain E. coli CPSs (Valle et al., 2006). In summary, the cell surfaces of E. coli isolates are rich in complex carbohydrates and these molecules play diverse, niche-dependent roles in the physiology of E. coli. In this chapter, we will focus only on LPS and CPS.
Structure and biosynthesis of E. coli LPS
The LPS molecule contains three regions, the lipid A, core, and O-PS. Lipid A is the most highly conserved portion of the molecule. The typical structure contains two phosphorylated glucosamine residues with six acyl chains (Figure 17.2A), though this can be modified in several places to alter the biological properties of the molecule (reviewed in Raetz et al., 2007) (see below). The core oligosaccharide can be divided into two regions. The inner core consists of two residues of 3-deoxy-D–manno-oct-2-ulosonic acid (Kdo) and three residues of L-glycero-D–manno-heptose (Hep) but it may also be modified with a number of other groups such as phosphate, pyrophosphorylethanolamine, or additional sugars (Figure 17.2B). This region is highly conserved in E. coli and Salmonella and is important for membrane stability (reviewed in Heinrichs et al., 1998). For example, loss of the phosphate moieties on Hep residues compromises outer membrane integrity and, in Salmonella, attenuates virulence (Yethon et al., 2000). The outer core serves as the point of attachment for O-PS and contains sugars such as glucose (Glc), galactose (Gal), glucosamine (GlcN), and N-acetylglucosamine (GlcNAc). Despite the potential for great diversity, there are only five known core types in E. coli, K-12, R1, R2, R3, and R4 (Heinrichs et al., 1998; Kaniuk et al., 2004). The core type R1 is most commonly found in E. coli causing extraintestinal infection while the verotoxin-producing E. coli isolates are predominantly R3 (Figure 17.2B).
Lipid A-core is synthesized as one unit in a step-wise manner by the Lpx and Waa enzymes, starting with the acylation of UDP-GlcNAc on the cytoplasmic face of the inner membrane (Figure 17.3) (reviewed in Raetz and Whitfield, 2002; Raetz et al., 2007). Most of the steps in this process were established by the efforts of Christian Raetz and his research group, leading to what is often known as the ‘Raetz pathway’. In this conserved pathway, formation of tetraacyl lipid A (called lipid IVA) is followed by addition of the two inner core Kdo residues before the secondary acyl chains can be added to complete the hexaacyl lipid A. Once the lipid A-core is complete, it is flipped to the periplasmic side of the inner membrane by MsbA, an essential protein belonging to the ATP-binding cassette (ABC) transporter superfamily. MsbA plays a crucial role in quality control of lipid A structure since it has a preference for hexaacyl lipid A over earlier intermediates (such as lipid IVA) as a substrate (Doerrler and Raetz, 2002). As a result, the minimal LPS structure for export and cell viability is normally considered to be Kdo2-lipid A. However, suppressor mutants lacking Kdo (and therefore also devoid of secondary acyl chains) are viable, albeit with a compromised outer membrane barrier, leading to LPS molecules comprising lipid IVA (Meredith et al., 2006). Alternatively, the absence of Kdo can also be overcome by overexpression of the secondary acyltransferases to generate pentaacyl and hexaacyl free lipid A (Reynolds and Raetz, 2009). After export of lipid A-core to the periplasmic face of the inner membrane, the ligase enzyme (WaaL) covalently links it to the O antigen, which is synthesized separately.
The O antigen is highly variable and typically consists of repeat units of 2–5 sugars in a polymer that can be more than 100 sugars long. The range of repeat unit numbers is specific for a particular strain and is controlled by different strategies, depending on which of the two biosynthetic pathways is used for O-antigen biosynthesis: the Wzy-dependent pathway or the ABC transporter-dependent pathway (Figure 17.3) (Raetz and Whitfield, 2002). In all cases, biosynthesis of O antigens begins at the cytoplasmic face of the inner membrane using the lipid carrier undecaprenyl phosphate. In E. coli, the enzyme WecA initiates O-PS biosynthesis by transferring GlcNAc-1-phosphate from UDP-GlcNAc to undecaprenyl phosphate to form undecaprenyl pyrophosphoryl-GlcNAc. In some cases, this is epimerized to undecaprenyl pyrophosphoryl-GalNAc (Rush et al., 2010). The GlcNAc/GalNAc residues occur in each repeat unit in O-PSs formed by the Wzy-dependent pathway, or just once at the reducing terminus in the ABC transporter-dependent process.
In the Wzy-dependent pathway, individual repeat units are synthesized on undecaprenyl pyrophosphoryl-GlcNAc/GalNAc using nucleoside phosphate sugar donors by enzymes encoded in the locus. These repeat units are then flipped to the periplasmic side of the inner membrane by the flippase Wzx and polymerized into the complete O antigen by the polymerase Wzy. Wzy transfers the growing glycan from one undecaprenyl pyrophosphate carrier to the incoming lipid-linked repeat unit. Insight into this pathway has benefitted from model systems, including the O7 and O86 antigens (Table 17.1), studied in the laboratories of Miguel Valvano and George Peng Wang, respectively. In the ABC transporter-dependent pathway, the O antigen is synthesized entirely at the cytoplasmic face of the inner membrane through the sequential action of glycosyltransferases that add sugars to undecaprenyl pyrophosphoryl-GlcNAc, before the completed chain is exported via an ABC transporter. This process is less common than the Wzy-dependent pathway and the resulting repeat-unit structures tend to be less elaborate. The serotype O8/O9/O9a antigens provide influential prototypes for this type of assembly process and many of the steps have been resolved by the research group of Klaus Jann and the Whitfield lab (Table 17.1). The E. coli WaaL ligase can operate effectively with nascent undecaprenyl-linked O-PSs from either pathway.
The LPS species extracted from a bacterial cell shows heterogeneity best illustrated by profiles separated by SDS-PAGE (Hitchcock and Brown, 1983). The most obvious variations occur in the chain-lengths of O-PS, evident as a ladder of high-molecular-weight molecules. The length of the O-PS has important functional consequences (see below) and is established by different mechanisms, depending on the assembly pathway. In the Wzy-dependent pathway, the length of the O antigen is controlled by the protein Wzz, a transmembrane protein with a large periplasmic domain that belongs to the polysaccharide co-polymerase (PCP) family (reviewed in Cuthbertson et al., 2009; Morona et al., 2009). In the ABC transporter-dependent pathway, chain length of some O-PS molecules is controlled by the addition of novel residues (ones not found in the repeat-unit domain) to the non-reducing end of the glycan (reviewed in Cuthbertson et al., 2010). These residues may be alternative sugars or non-glycose moieties and they not only prevent further elongation of the glycan, but are also required to engage the ABC transporter for export across the inner membrane. However, other O antigens in this pathway engage the ABC transporter in the absence of any identifiable terminating group and coordination of the activities of the O-PS elongating enzymes and the ABC transporter determine O-PS chain length (Cuthbertson et al., 2010).
The Lpt proteins, which span the inner and outer membranes as well as the periplasm, are responsible for transport of the mature LPS. Given the heterogeneity in LPS species, this system can effectively handle molecules with diverse structures and it is well conserved across bacterial species though it has been identified only recently. In the current working model, LptBFG form an ABC protein complex to extract nascent LPS out of the inner membrane. The molecule is passed via LptC to a filament of LptA molecules spanning the periplasm and then to LptDE located in the outer membrane, which completes translocation to the cell surface (reviewed in Ruiz et al., 2009; Sperandeo et al., 2009; Chng et al., 2010).
Most LPS modifications occur at the periplasmic face of either the inner or outer membrane and, as such, can serve as markers indicating the stage of transport. These include modification of the phosphate groups with 4-amino-4-deoxy-L-arabinose (L-Ara4N) or ethanolamine by enzymes found in the inner membrane, which increases bacterial resistance to innate immune defenses (see below). The outer membrane protein PagP adds a secondary palmitate in an acyloxyacyl linkage to the hydroxy-myristate located at the 2-position. The resulting heptaacyl species is a much less potent activator of cytokine induction (Raetz et al., 2007). PagP is normally latent in E. coli but is activated by membrane perturbations and by defects in acylation of the 3´ position of the diglucosamine backbone with a myristol residue. However, this modification can alter host–pathogen interactions in other ways, because PagP activity in E. coli O157 has an indirect effect on the completion of the LPS core oligosaccharide, leading to loss of O antigen and serum sensitivity (Smith et al., 2008).
Structure and biosynthesis of E. coli CPSs
The CPSs of E. coli have been subdivided into four groups based on structural and genetic criteria (reviewed in Whitfield, 2006). Group 1 and 4 capsules share the same mode of synthesis, a Wzy-dependent pathway, and mainly differ in the chromosomal locations of key genes. Similarly, groups 2 and 3 capsules are both produced by ABC transporter-dependent processes but the genes are organized differently within the locus.
Group 1 (and 4) CPS are found in E. coli isolates causing intestinal infections. They are heteropolymers of repeating sugar units, as in many O antigens. Biosynthesis uses a process identical to the Wzy-dependent O antigens but the pathways diverge once the polymerized glycan is formed at the periplasmic face of the inner membrane (Figure 17.4). O antigens enter the LPS assembly pathway by ligation to lipid A-core and are transferred to the surface via Lpt proteins. Capsular K antigens have their own surface assembly process that involves four key components: (i) Wzc, an inner membrane PCP protein belonging to the PCP-2a subfamily; (ii) Wzb, a cytoplasmic protein tyrosine phosphatase; (iii) Wza, an outer membrane lipoprotein belonging to the OPX (outer membrane polysaccharide export) protein family (Cuthbertson et al., 2009); and (iv) an accessory outer membrane protein called Wzi. Understanding the group 1 CPS translocation processes has come from studies with the serotype K30 prototype in the Whitfield laboratory. In O-antigen biosynthesis, the corresponding PCP protein belongs to sub-family 1 and its action appears to be confined to regulating the polymerization activity, although the exact mechanism is still unknown. PCP-2a proteins are more complex. Wzc certainly affects polymerization but this activity (and capsule formation) is dependent on the activity of a C-terminal tyrosine autokinase domain, as well as the dephosphorylation of these residues by the Wzb phosphatase (Wugeditsch et al., 2001). The autokinase domain is absent in PCP-1 family members. The additional role played by Wzc is mediated by its periplasmic domain, which is larger in PCP-2a proteins, and interacts with the extensive periplasmic region of Wza (Collins et al., 2007). The crystal structure of Wza reveals an octomer which forms a large periplasmic barrel connected to an outer membrane channel (Dong et al., 2006). The outer-membrane pore is formed by one α-helix contributed by each protamer and was the first example of an outer-membrane channel which is not a β-barrel (Dong et al., 2006). It is not known how the Wzc/Wza complex transports the capsule to the outer membrane or what the nature of the reducing end of the exported polymer is; i.e. is the CPS attached to an anchoring protein or lipid. In O-antigen biosynthesis, the ligase (WaaL) releases the nascent glycan from the undecaprenyl pyrophosphate carrier and links it to lipid A-core. There is no corresponding activity in the biosynthesis of group 1 and 4 K antigens. The CPS could retain its linkage to undecaprenyl pyrophosphate, or it could potentially be released by a leaky polymerization step, where the transfer of the growing chain to the incoming repeat unit may be incomplete. Wzi is important for surface retention of group 1 CPS although its precise function is not yet known and it is not present in group 4 systems (Rahn et al., 2003). The Wza-Wzb-Wzc proteins are highly conserved in all isolates possessing a group 1 or 4 capsule, indicating that they do not recognize any particular glycan repeat-unit structure. Group 1 K antigens are typically co-expressed with an O antigen synthesized by the ABC transporter pathway (e.g. O8/O9/O9a) and the corresponding gene clusters are both located near his. The group 1 gene cluster is allelic with genes for colanic acid biosynthesis, so expression of a capsule and colanic acid are mutually exclusive. However, group 4 K antigens really emphasize the parallels between O- and K-antigen biosynthesis. In these cases, the structures of the repeat units are identical and are produced by the same chromosomal locus. Some undecaprenyl pyrophosphate-linked molecules are diverted by WaaL into LPS, while others enter a CPS translocation pathway by Wza-Wzb-Wzc proteins that are encoded by a separated locus elsewhere on the chromosome (Peleg et al., 2005). Serotype O111 provided the first example, and the use of the same repeat structure led to the early description of ‘O-antigen capsules’ (now group 4) (Goldman et al., 1982). It is now known that serotypes O127 and O157 also have group 4 capsules (Table 17.1) (Peleg et al., 2005; Shifrin et al., 2008). Isolates that can produce group 4 CPSs (unlike their group 1 counterparts) retain the genes for colanic acid production.
K antigens from extraintestinal pathogenic E. coli mostly fall into groups 2 and 3 (Whitfield, 2006). These include capsules from isolates causing urinary tract infections and meningitis. The production of many of these K antigens is temperature-regulated, unlike group 1 and 4 K antigens, with expression being ‘on’ at 37°C but ‘off’ at temperatures below 20°C. Some of the CPS structures resemble eukaryotic glycans and this is thought to aid virulence by preventing an effective immune response. For example, the K4 glycan is fructosylated chondroitin and the K5 glycan is heparosan, both similar to human glycosaminoglycans (Table 17.1). As well, the meningitis-causing K1 isolates possess a CPS containing α2,8-linked polysialic acid, which is identical in structure to the glycan found on neural cell adhesion molecule (NCAM) in the human brain. The K1 and K5 systems have been influential models for understanding the mechanisms of biosynthesis through studies in the laboratories of Eric Vimr, Willie Vann, and Ian Roberts. ABC transporter-dependent capsules are synthesized entirely on the cytoplasmic face of the inner membrane, similar to ABC transporter-dependent O antigens (Figure 17.4) (Whitfield, 2006). However, one critical difference is that undecaprenyl phosphate is apparently not involved (Finke et al., 1991). It has been shown that the mature capsular polysaccharide is attached at the reducing end to a phospholipid although the precise chemical structure has not been resolved (Gotschlich et al., 1981). In the essentially identical process in N. meningitidis, lipidation occurs before transport of polysialic acid CPS to the cell surface, but it is not known whether the glycan is synthesized directly on this phospholipid or if it is synthesized on another molecule before transfer to the phospholipid (Tzeng et al., 2005). Once the capsule is synthesized, it is transported through the inner membrane by the ABC transporter (KpsMT) (reviewed in Vimr and Steenbergen, 2009). The final steps of glycan translocation are mediated by KpsE, a representative of the PCP-3 subfamily, and KpsD, an OPX protein (Cuthbertson et al., 2009). A multiprotein export complex comprising KpsMTED is thought to span the cell envelope (Rigg et al., 1998; McNulty et al., 2006). Like the group 1 and 4 translocation export proteins, they are conserved in all isolates possessing a group 2 or 3 capsule, indicating that they also do not recognize any particular glycan repeat-unit structure. The genes encoding group 2 and 3 K antigens are located on the chromosome near serA and these glycans can be found together with a wide range of O serotypes, many formed by a Wzy-dependent system.
Evasion of host cell defenses
Mammals have evolved with the pressure of bacteria, viruses, and fungi and so have developed ways of dealing with microbial infections; the most extensive defense machinery is the immune system. The human immune system consists of two branches, innate and adaptive immunity, between which there is extensive crosstalk. Innate immunity has developed as a first line of defense against challenges not necessarily seen before to protect the body from pathogens. Toll-like receptors (TLR) are the major effectors of innate immunity which recognize conserved structures in pathogens called pathogen-associated molecular patterns (PAMPs), somewhat of a misnomer, as they are found in non-pathogenic as well as pathogenic microbes; in Gram-negative bacteria, these include LPS, CPS, flagella, and nucleic acids. Binding of TLRs to their respective ligands activates a signaling cascade, resulting in recruitment and activation of immune cells, which can then eliminate the threat. In addition to the cellular receptors, there are also soluble complement proteins found in serum, which can bind directly to bacterial cell surfaces leading to opsonization, phagocytosis, and formation of the membrane attack complex leading to lysis. LPS and CPS are important parts of the arsenal of virulence factors used by bacteria to circumvent these processes.
Lipid A structure influences susceptibility to polycationic peptides
Cationic antimicrobial peptides (CAMPs) are short peptides secreted by immune and epithelial cells in response to bacterial products, like LPS, and other inflammatory signals (reviewed in Brown and Hancock, 2006). It is well established that the structure of lipid A has a profound effect on the susceptibility of bacteria to CAMPs and polycationic drugs such as polymyxin B. These compounds typically exploit negatively charged phosphate residues on the diglucosamine backbone to bind to the cell surface and then insert into the membrane, causing disruption of the permeability barrier and lysis of the cell (Brown and Hancock, 2006). Many bacteria can overcome susceptibility to these compounds by modifying the 1 and 4’ phosphates with L-Ara4N or phosphorylethanolamine (PEtN) by the activity of ArnT and EptA respectively, following transfer of the nascent lipid A-core to the periplasm (Raetz et al., 2007). The enzymes involved in these processes in E. coli are activated under specific growth conditions (e.g. growth at low pH or in the presence of cationic peptides) and are under the regulation of the PmrAB and PhoPQ two-component systems (Guo et al., 1997; Gunn, 2008).
An additional modification involves phosphorylation of the 1-phosphate on the lipid A backbone to make pyrophosphate and is mediated by LpxT using undecaprenyl pyrophosphate as a donor (Touze et al., 2008). This activity helps recycle the essential lipid carrier for peptidoglycan, O antigen, group 1 K antigens, etc., but other cellular proteins can also fulfill this need (El Ghachi et al., 2005). The activity of LpxT is inhibited in PmrA-activated cells (Herrera et al., 2010).
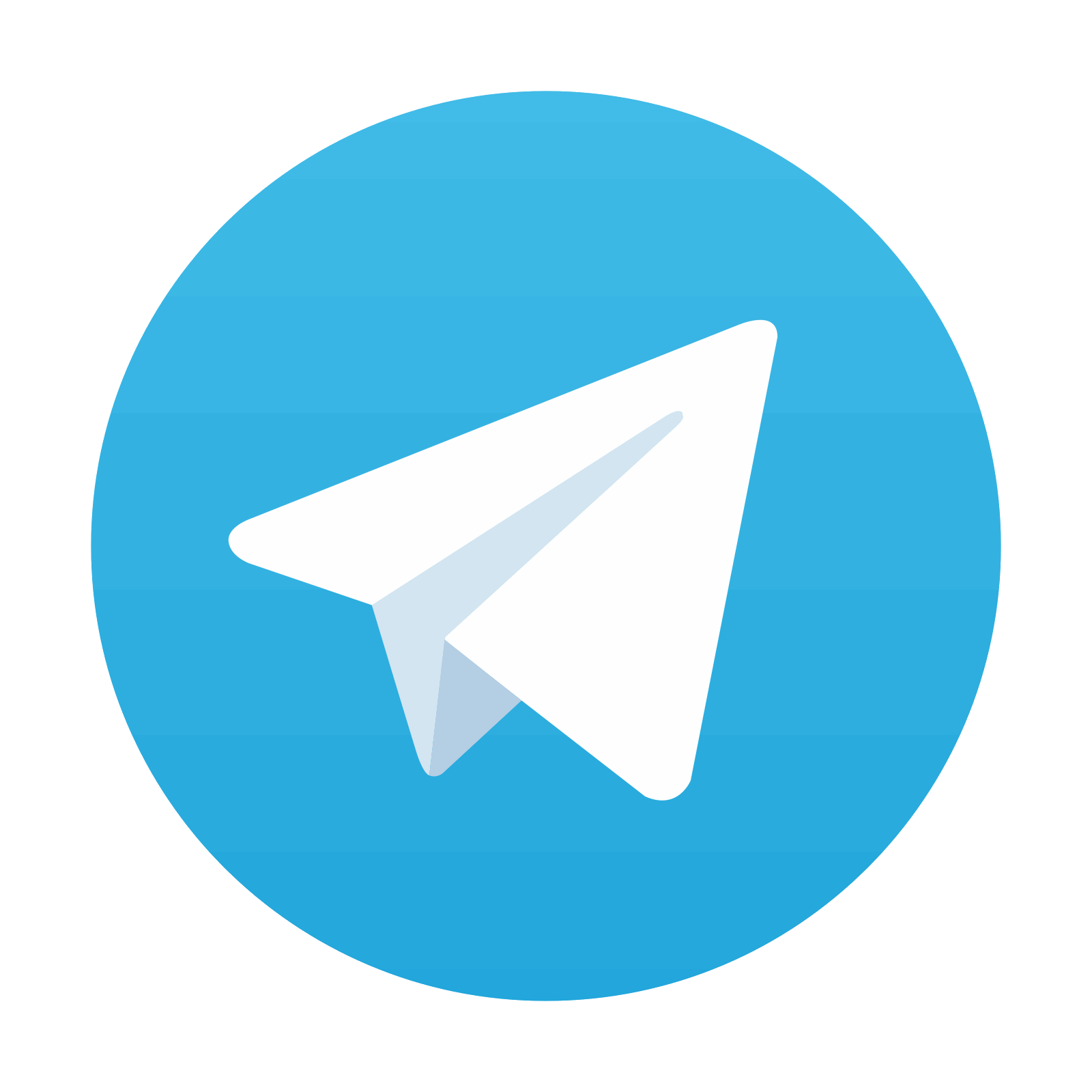
Stay updated, free articles. Join our Telegram channel

Full access? Get Clinical Tree
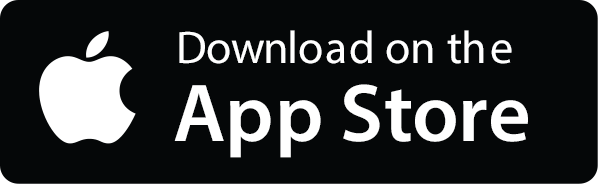
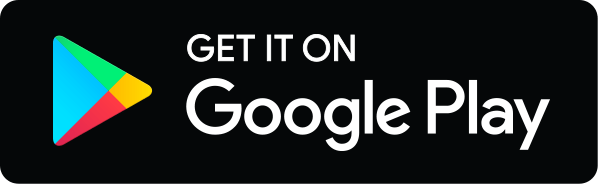