High-Yield Terms to Learn
Cell cycle-nonspecific (CCNS) drug An anticancer agent that acts on tumor stem cells when they are traversing the cell cycle and when they are in the resting phase Cell cycle-specific (CCS) drug An anticancer agent that acts selectively on tumor stem cells when they are traversing the cell cycle and not when they are in the G0 phase
Growth fraction The proportion of cells in a tumor population that are actively dividing Myelosuppressant A drug that suppresses the formation of mature blood cells such as erythrocytes, leukocytes, and platelets. This effect is also known as “bone marrow suppression” Oncogene A mutant form of a normal gene that is found in naturally occurring tumors and which, when expressed in noncancerous cells, causes them to behave like cancer cells
Cancer Cell Cycle Kinetics
Cell Cycle Kinetics
Cancer cell population kinetics and the cancer cell cycle are important determinants of the actions and clinical uses of anticancer drugs. Some anticancer drugs exert their actions on cells undergoing cycling (cell cycle-specific [CCS] drugs), and others (cell cycle-nonspecific [CCNS] drugs) kill tumor cells in both cycling and resting phases of the cell cycle (although cycling cell are more sensitive). CCS drugs are usually most effective when cells are in a specific phase of the cell cycle (Figure 54-1). Both types of drugs are particularly effective when a large proportion of the tumor cells are proliferating (ie, when the growth fraction is high).
FIGURE 54-1
Phases of the cell cycle that are susceptible to the actions of cell cycle-specific (CCS) drugs. All dividing cells—normal and neoplastic—must traverse these cell cycle phases before and during cell division. Tumor cells are usually most responsive to specific drugs (or drug groups) in the phases indicated. Cell cycle-nonspecific (CCNS) drugs act on tumor cells while they are actively cycling and while they are in the resting phase (G0).
(Reproduced and modified, with permission, from Katzung BG, editor: Basic & Clinical Pharmacology, 11th ed. McGraw-Hill, 2009: Fig. 54-2.)
The Log-Kill Hypothesis
Cytotoxic drugs act with first-order kinetics in a murine model of leukemia. In this model system, in which all the cells are actively progressing through the cell cycle, a given dose kills a constant proportion of a cell population rather than a constant number of cells. The log-kill hypothesis proposes that the magnitude of tumor cell kill by anticancer drugs is a logarithmic function. For example, a 3-log-kill dose of an effective drug reduces a cancer cell population of 1012 cells to 109 (a total kill of 999 x 109 cells); the same dose would reduce a starting population of 106 cells to 103 cells (a kill of 999 x 103 cells). In both cases, the dose reduces the numbers of cells by 3 orders of magnitude, or “3 logs.” A key principle that stems from this finding and that is applicable to hematologic malignancies is an inverse relationship between tumor cell number and curability (Figure 54-2). Mathematical modeling data suggest that most human solid tumors do not grow in such an exponential manner and rather that the growth fraction of the tumor decreases with time owing to blood supply limitations and other factors. In drug-sensitive solid tumors, the response to chemotherapy depends on where the tumor is in its growth curve.
FIGURE 54-2
Relationship, based on the log-kill hypothesis, of tumor cell number to 3 approaches to drug treatment and to no treatment (dashed line). In the protocol diagrammed at the top, infrequent treatment (indicated by arrows) prolongs survival but with recurrence of symptoms between treatments and eventual death. With the regimen diagrammed in the middle section that is more intensive and begun earlier, cure results after many cycles of therapy. In the treatment diagrammed near the bottom of the graph, early surgery removes much of the tumor burden, and intensive adjuvant chemotherapy has been used long enough to produce a cure.
(Reproduced with permission, from Katzung BG, editor: Basic & Clinical Pharmacology, 11th ed. McGraw-Hill, 2009: Fig. 54-1.)
Resistance to Anticancer Drugs
Drug resistance is a major problem in cancer chemotherapy. Mechanisms of resistance include the following:
Increased DNA Repair
An increased rate of DNA repair in tumor cells can be responsible for resistance and is particularly important for alkylating agents and cisplatin.
Formation of Trapping Agents
Some tumor cells increase their production of thiol trapping agents (eg, glutathione), which interact with anticancer drugs that form reactive electrophilic species. This mechanism of resistance is seen with the alkylating agent bleomycin, cisplatin, and the anthracyclines.
Changes in Target Enzymes
Changes in the drug sensitivity of a target enzyme, dihydrofolate reductase, and increased synthesis of the enzyme are mechanisms of resistance of tumor cells to methotrexate.
Decreased Activation of Prodrugs
Resistance to the purine antimetabolites (mercaptopurine, thioguanine) and the pyrimidine antimetabolites (cytarabine, fluorouracil) can result from a decrease in the activity of the tumor cell enzymes needed to convert these prodrugs to their cytotoxic metabolites.
Inactivation of Anticancer Drugs
Increased activity of enzymes capable of inactivating anticancer drugs is a mechanism of tumor cell resistance to most of the purine and pyrimidine antimetabolites.
Decreased Drug Accumulation
This form of multidrug resistance involves the increased expression of a normal gene (MDR1) for a cell surface glycoprotein (P-glycoprotein). This transport molecule is involved in the accelerated efflux of many anticancer drugs in resistant cells.
Strategies in Cancer Chemotherapy
Cancer Treatment Modalities
Chemotherapy is used in three main clinical settings:
Primary Induction Chemotherapy
Drug therapy is administered as the primary treatment for many hematologic cancers and for advanced solid tumors for which no alternative treatment exists. Although primary induction can be curative in a small number of patients who present with advanced metastatic disease (eg, lymphoma, acute myelogenous leukemia, germ cell cancer, choriocarcinoma, and several childhood cancers), in many cases the goals of therapy are palliation of cancer symptoms, improved quality of life, and increased time to tumor progression.
Neoadjuvant Chemotherapy
The use of chemotherapy in patients who present with localized cancer for which alternative local therapy, such as surgery, exist is known as neoadjuvant chemotherapy. The goal is to render the local therapy more effective.
Adjuvant Chemotherapy
In the treatment of many solid tumors, chemotherapy serves as an important adjuvant to local treatment procedures such as surgery or radiation. The goal is to reduce the risk of local and systemic recurrence and to improve disease-free and overall survival.
Principles of Combination Therapy
Chemotherapy with combinations of anticancer drugs usually increases log-kill markedly, and in some cases synergistic effects are achieved. Combinations are often cytotoxic to a heterogeneous population of cancer cells and may prevent development of resistant clones. Drug combinations using CCS and CCNS drugs may be cytotoxic to both dividing and resting cancer cells. The following principles are important for selecting appropriate drugs to use in combination chemotherapy:
(1) Each drug should be active when used alone against the particular cancer.
(2) The drugs should have different mechanisms of action.
(3) Cross-resistance between drugs should be minimal.
(4) The drugs should have different toxic effects (Table 54-1).
TABLE 54-1 Selected examples of cancer chemotherapy.
Diagnosis Examples of Commonly-Used Anticancer Drugs Acute lymphocytic leukemia in children Prednisone, vincristine, and asparaginase or an anthracycline, plus intrathecal methotrexate Acute myelogenous leukemia in adults Cytarabine and idarubicin or daunorubicin Breast carcinoma Cytotoxic agents, hormonal therapy with tamoxifen or an aromatase inhibitor (eg, anastrozole), trastuzumab Chronic myelogenous leukemia Imatinib, newer tyrosine kinase inhibitors, interferon Colon carcinoma Fluorouracil plus leucovorin plus oxaliplatin Hodgkin’s lymphoma ABVD regimen: doxorubicin (Adriamycin), bleomycin, vincristine, dacarbazine, and prednisone Non-Hodgkin’s lymphoma CHOP regimen (cyclophosphamide, doxorubicin, vincristine, and prednisone) plus rituximab Ovarian carcinoma Paclitaxel and carboplatin Pancreatic carcinoma Gemcitabine and erlotinib Prostate carcinoma GnRH agonist (eg, leuprolide) or antagonist (eg, abarelix) and androgen receptor antagonist (eg, flutamide) Lung carcinoma Carboplatin, paclitaxel, and bevacizumab Testicular carcinoma PEB regimen: cisplatin (Platinol), etoposide, and bleomycin
GnRH, gonadotropin-releasing hormone.
Rescue Therapy
Toxic effects of anticancer drugs can sometimes be alleviated by rescue strategy. For example, high doses of methotrexate may be given for 36-48 h to cells of the gastrointestinal tract and bone marrow and terminated before severe toxicity occurs. Leucovorin, a form of tetrahydrofolate that is accumulated more readily by normal than by neoplastic cells, is then administered. This results in rescue of the normal cells because leucovorin bypasses the dihydrofolate reductase step in folic acid synthesis.
Mercaptoethanesulfonate ( mesna ) “traps” acrolein released from cyclophosphamide and thus reduces the incidence of hemorrhagic cystitis. Dexrazoxane inhibits free radical formation and affords protection against the cardiac toxicity of anthracyclines (eg, doxorubicin).
Alkylating Agents
The alkylating agents include nitrogen mustards ( chlorambucil, cyclophosphamide, mechlorethamine ), nitrosoureas ( carmustine, lomustine ), and alkyl sulfonates ( busulfan ). Other drugs that act in part as alkylating agents include cisplatin, dacarbazine, and procarbazine.
The alkylating agents are CCNS drugs. They form reactive molecular species that alkylate nucleophilic groups on DNA bases, particularly the N-7 position of guanine. This leads to cross-linking of bases, abnormal base-pairing, and DNA strand breakage. Tumor cell resistance to the drugs occurs through increased DNA repair, decreased drug permeability, and the production of trapping agents such as thiols.
Cyclophosphamide
Pharmacokinetics
Hepatic cytochrome P450-mediated biotransformation of cyclophosphamide is needed for antitumor activity. One of the breakdown products is acrolein.
Clinical Use
Uses of cyclophosphamide include leukemia, non-Hodgkin’s lymphoma, breast and ovarian cancers, and neuroblastoma.
Toxicity
Gastrointestinal distress, myelosuppression, and alopecia are expected adverse effects of cyclophosphamide. Hemorrhagic cystitis resulting from the formation of acrolein may be decreased by vigorous hydration and by use of mercaptoethanesulfonate ( mesna ). Cyclophosphamide may also cause cardiac dysfunction, pulmonary toxicity, and a syndrome of inappropriate antidiuretic hormone (ADH) secretion.
Mechlorethamine
Mechanism and Pharmacokinetics
Mechlorethamine spontaneously converts in the body to a reactive cytotoxic product.
Clinical Use
Mechlorethamine is best known for use in regimens for Hodgkin’s and non-Hodgkin’s lymphoma.
Toxicity
Gastrointestinal distress, myelosuppression, alopecia, and sterility are common. Mechlorethamine has marked vesicant actions.
Platinum Analogs (Cisplatin, Carboplatin, Oxaliplatin)
Pharmacokinetics
Cisplatin is used intravenously; the drug distributes to most tissues and is cleared in unchanged form by the kidney.
Clinical Use
Cisplatin is commonly used as a component of regimens for testicular carcinoma and for cancers of the bladder, lung, and ovary. Carboplatin has similar uses. Oxaliplatin is used in advanced colon cancer.
Toxicity
Cisplatin causes gastrointestinal distress and mild hematotoxicity and is neurotoxic (peripheral neuritis and acoustic nerve damage) and nephrotoxic. Renal damage may be reduced by the use of mannitol with forced hydration. Carboplatin is less nephrotoxic than cisplatin and is less likely to cause tinnitus and hearing loss, but it has greater myelosuppressant actions. Oxaliplatin causes dose-limiting neurotoxicity.
Procarbazine
Mechanisms
Procarbazine is a reactive agent that forms hydrogen peroxide, which generates free radicals that cause DNA strand scission.
Pharmacokinetics
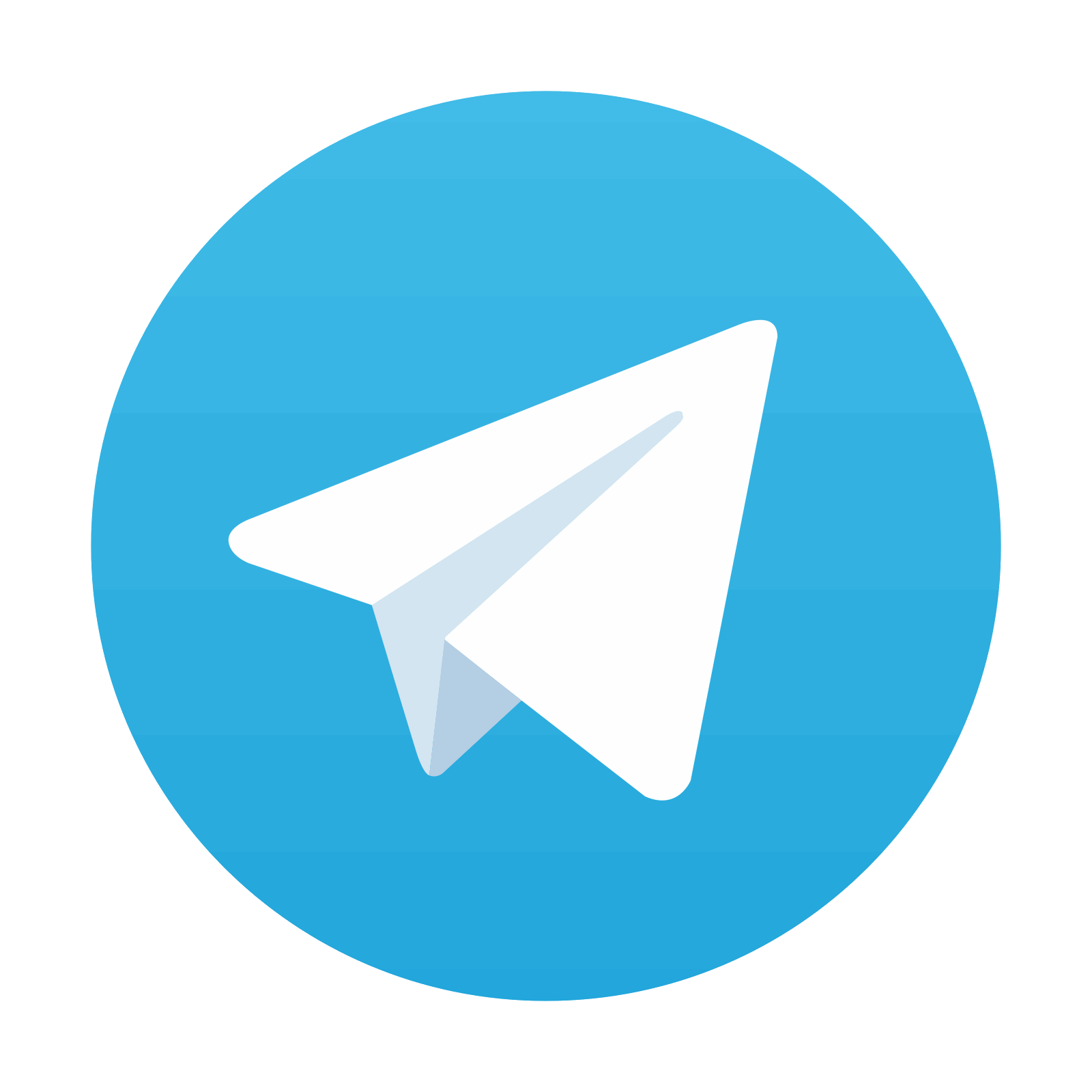
Stay updated, free articles. Join our Telegram channel

Full access? Get Clinical Tree
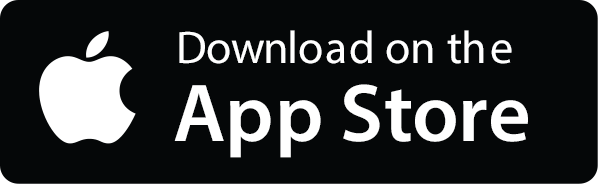
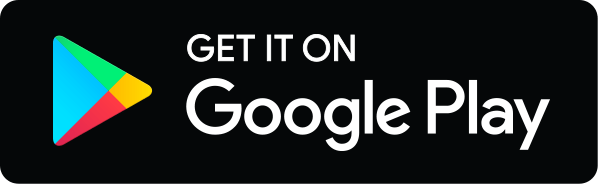