16 Bone
Normal bone
Bone collagen
The collagen found in bone differs from other collagen in the body in that it becomes mineralized and is laid down in bands or lamellae roughly parallel to one another. The collagen fibers within each lamella tend to lie next each other but at an angle to the fibers in adjacent lamellae. A cement of proteoglycan ground substance outlining these fibers is seen in sections only at the cement lines. The organization of collagen lamellae is responsible for the distinctive micro-anatomical patterns of bone which are easily seen with polarized light microscopy (Fig. 16.1).
The simplest pattern occurs on the periosteal and endosteal surfaces of compact bone as circumferential lamellae, and in trabecular or non-Haversian bone where lamellae are roughly parallel to the surface. Cortical bone is composed of Haversian systems or osteons in which concentric lamellae surround channels (Volkmann’s canals) containing one or more blood vessels. These tubular structures run longitudinally in the bone and are packed closely together with irregular interstices filled by the remnants of older osteons (Fig. 16.2). Cement lines outline the boundaries of osteons, and some trabecular and circumferential lamellae.
Bone cells
There are three types of bone cell other than the marrow cells belonging to the hemopoietic system.
Osteocytes
In one respect osteoblasts do represent immature cells, as some are trapped in the osteoid matrix they lay down, and become mature osteocytes residing in tiny spaces or lacunae within the bone. Lacunae are connected to each other and to the vascular spaces by canaliculi – tiny channels into which osteocyte processes project for the purpose of passing fluids and dissolved substances necessary for cell metabolism (Fig. 16.3).
Osteoclasts
These are the cells responsible for bone resorption or erosion. Osteoclasts are large multinucleated (giant) cells whose cytoplasm contains numerous mitochondria and alkaline phosphatase. They occur in small clusters or singly on bone surfaces undergoing resorption and are often seen in the depressions (Howship’s lacunae) they are actively creating by erosion. These surfaces have an irregular outline and lack osteoid (Fig. 16.4). The direction of resorption is random, with no relationship to lamellar structure. Osteoclasts respond to altered mechanical stresses on the skeleton and to growth, and their activity contributes to remodeling. They also respond to hormones that can either stimulate or inhibit their activity. When resorption halts, the process of bone formation resumes (osteoid, mineralization, etc.). Cement lines will occur at the junction between old and newly formed bone.
Techniques for analyzing bone
• Decalcified bone for frozen, paraffin, and transmission electron microscopy (TEM).
• Mineralized bone for frozen, plastic (microtomed or sawn/ground sections), transmission or scanning EM samples.
Biopsies
Biopsies are used for diagnosis of several diseases such as cancers, hemopoietic disorders, and infections. These specimens are usually small enough to treat much like soft tissue, except that a bone biopsy usually needs decalcification. This is particularly neccesary if it contains a piece of the cortical bone in order to produce paraffin sections. A bone marrow biopsy is usually removed with a Jamshidi needle for diagnosis of metabolic bone disease. Metabolic bone diseases are diagnosed using trabecular bone (Byers & Smith 1967) taken from the iliac crest which is an accessible bone site representative of skeletal bone as a whole.
Sections that are requisite to assess the relationship between mineralized and unmineralized bone (osteoid) are best processed and embedded into a plastic, such as methyl methacrylate (MMA), glycolmethacrylate or a epon-like plastic. The preferred method used for metabolic bone disease research and diagnosis is MMA plastic. Plastic is not a common embedding medium in clinical laboratories but is useful in research and clinical laboratories that are connected with research. It is also possible to produce frozen sections from an undecalcified bone biopsy. There are silver stains that demonstrate bone and osteoid in a decalcified, paraffin-embedded bone section (Tripp & McKay 1972) but many researchers choose the MMA-embedded section.
Fine-detail specimen radiography
Radiographs of bone slabs, blocks, or fragments are useful for four main purposes:
1. To examine the nature and extent of a lesion.
2. To provide a diagram of a lesion prior to block selection for processing.
3. To check progress of decalcification, i.e. decalcification endpoint test.
4. To confirm the presence of foreign materials, e.g. prosthetic devices, metal or glass fragments implanted by trauma.
An example of manual exposure requirements is as follows:
• bone slab 3–5 mm thick on polyethylene sheet (moisture barrier)
• Kodak X-OMAT 2, Ready Pak (Kodak Ltd)
• film-to-source distance (FTSD), 50 cm (lowest shelf level)
Decalcification
Decalcifying agents
As noted previously there are two major types of decalcifying agent, i.e. acids and chelating agents, although Gray (1954) lists over 50 different mixtures. Many of these mixtures were developed for special purposes with one used as a fixing and dehydrating agent. Other mixtures contain reagents, e.g. buffer salts, chromic acid, formalin, or ethanol, intended to counteract the undesirable swelling effects that acids have on tissues. Many popular mixtures used today are from the original formulae developed many years ago (Evans & Krajian 1930; Kristensen 1948; Clayden 1952). For most practical purposes, today’s laboratories seem to prefer simpler solutions for routine work. Provided the bone is totally fixed and treated with a decalcifier suitable for removal of the amount of mineral present, the simple mixtures work as well or better than more complex mixtures.
Acid decalcifiers
Acid decalcifiers can be divided into two groups: strong (inorganic) and weak (organic) acids. As Brain (1966) suggested, many laboratories keep an acid from each group available for either rapid diagnostic or slower, routine work.
Proprietary decalcifiers
The components in proprietary decalcifying solutions are often trade secrets. Manufacturers provide Material Safety Data Sheets (MSDS) that frequently indicate the type and concentration of acid. Their product data sheets usually indicate if a solution is rapid or slow, and give decalcification instructions and warnings against prolonged use. Rapid proprietary solutions usually contain hydrochloric acid (HCl), whereas slow proprietary mixtures contain buffered formic acid or formalin/formic acid. A study (Callis & Sterchi 1998) found that dilution of a proprietary HCl solution was not deleterious for effective decalcification or staining, and this is an option if a strong mixture is considered too concentrated. Chelating reagents such as EDTA mixtures are also available pre-mixed. Although proprietary mixtures have no obvious advantages over solutions prepared in laboratories, their use is increasingly popular in busy laboratories because they are reliable, time and cost-effective while addressing some safety issues by eliminating handling and storage of concentrated acids.
Strong inorganic acids, e.g. nitric, hydrochloric
Strong acids are used for needle and small biopsy specimens to permit rapid diagnosis within 24 hours or less. They can be used for large or heavily mineralized cortical bone specimens with decalcification progress carefully monitored by a decalcification endpoint test (Callis & Sterchi 1998). The following is a list of strong acid decalcifying solutions. The formulae and preparations are available in Bancroft & Gamble, sixth ed. (2008).
Weak, organic acids, e.g. formic, acetic, picric
Of these, formic is the only weak acid used extensively as a primary decalcifier. Acetic and picric acids cause tissue swelling and are not used alone as decalcifiers but are found as components in Carnoy’s, Bouin’s, and Zenker’s fixatives. These fixatives will act as incidental, although weak, decalcifiers and could be used in urgent cases with only minimal calcification. Formic acid solutions can be aqueous (5–10%), buffered or combined with formalin. The formalin–10% formic acid mixture simultaneously fixes and decalcifies, and is recommended for very small bone pieces or needle biopsies. However, it is still advisable to have complete fixation before any acid decalcifier is used. The salts, sodium formate (Kristensen 1948) or sodium citrate (Evans & Krajian 1930), are added to formic acid solutions making ‘acidic’ buffers. Buffering is used to counteract the injurious effects of the acid. However, in addition to low 4–5% formic acid concentration, increased time is needed for complete decalcification. Formic acid is gentler and slower than HCl or nitric acids, and is suitable for most routine surgical specimens, particularly when immunohistochemical staining is needed. Formic acid can still damage tissue, antigens, and enzyme staining, and should be endpoint tested. Decalcification is usually complete in 1–10 days, depending on the size, type of bone, and acid concentration. Dense cortical or large bones have been effectively decalcified with 15% aqueous formic acid and a 4% hydrochloric acid–4% formic acid mixture (Callis & Sterchi 1998). The following is a list of weak acid decalcifying solutions. The formulae and preparations are available in Bancroft & Gamble, sixth ed. (2008).
Chelating agents
The chelating agent generally used for decalcification is ethylenediaminetetraacetic acid (EDTA). Although EDTA is nominally ‘acidic’, it does not act like inorganic or organic acids but binds metallic ions, notably calcium and magnesium. EDTA will not bind to calcium below pH 3 and is faster at pH 7–7.4; even though pH 8 and above gives optimal binding, the higher pH may damage alkali-sensitive protein linkages (Callis & Sterchi 1998). EDTA binds to ionized calcium on the outside of the apatite crystal and as this layer becomes depleted more calcium ions reform from within; the crystal becomes progressively smaller during decalcification. This is a very slow process that does not damage tissues or their stainability. When time permits, EDTA is an excellent bone decalcifier for enzyme staining, and electron microscopy. Enzymes require specific pH conditions in order to maintain activity, and EDTA solutions can be adjusted to a specific pH for enzyme staining. EDTA does inactivate alkaline phosphatase but activity can be restored by addition of magnesium chloride.
EDTA and EDTA disodium salt (10%) or EDTA tetrasodium salt (14%) are approaching saturation and can be simple aqueous or buffered solutions at a neutral pH of 7–7.4, or added to formalin. EDTA tetrasodium solution is alkaline, and the pH should be adjusted to 7.4 using concentrated acetic acid. The time required to totally decalcify dense cortical bone may be 6–8 weeks or longer, although small bone spicules may be decalcified in less than a week. Formulae and preparations are available in Bancroft & Gamble, sixth ed. (2008).
Factors influencing the rate of decalcification
Concentration of decalcifying agent
Generally, more concentrated acid solutions decalcify bone more rapidly but are more harmful to the tissue. This is particularly true of aqueous acid solutions, as various additives, e.g. alcohol or buffers, which protect tissues, may slow down the decalcification rate. Remembering that 1 N and 1 M solutions of HCl, nitric, or formic acid are equivalent, Brain (1966) found that 4 M formic acid decalcified twice as fast as a 1 M solution without harming tissue staining, and felt it was advantageous to use the concentrated formic acid mixture. With combination fixative-acid decalcifying solutions, the decalcification rate cannot exceed the fixation rate or the acid will damage or macerate the tissue before fixation is complete. Consequently, decalcifying mixtures should be compromises that balance the desirable effects (e.g. speed) with the undesirable effects (e.g. maceration, impaired staining).
Temperature
The optimal temperature for acid decalcification has not been determined, although Smith (1962) suggested 25°C as the standard temperature, but in practice a room temperature (RT) range of 18–30°C is acceptable. Conversely, lower temperature decreases reaction rates and Wallington (1972) suggested that tissues not completely decalcified at the end of a working week could be left in acid at 4°C over a weekend. This practice may result in ‘over-decalcification’ of tissues, even with formic acid. A better recommendation is to interrupt decalcification by briefly rinsing acid off bone, immersing it in NBF, removing from fixative, rinsing off the fixative, and resuming decalcification on the next working day. Microwave, sonication, and electrolytic methods produce heat, and must be carefully monitored to prevent excessive temperatures that damage tissue (Callis & Sterchi 1998).
Increased temperature also accelerates EDTA decalcification without the risk of maceration. However, it may not be acceptable for preservation of heat-sensitive antigens, enzymes, or electron microscopy work. Brain (1966) saw no objection to decalcifying with EDTA at 60°C if the bone was well fixed.
Agitation
The effect of agitation on decalcification remains controversial even though it is generally accepted that mechanical agitation influences fluid exchange within as well as around tissues with other reagents. Therefore, it would be a logical assumption that agitation speeds up decalcification, and studies have been done which attempt to confirm this theory. Russell (1963) used a tissue processor motor rotating at one revolution per minute and reported the decalcification period was reduced from 5 days to 1 day. Others, including Clayden (1952), Brain (1966), and Drury and Wallington (1980), repeated or performed similar experiments and failed to find any time reduction. The sonication method vigorously agitates both specimen and fluid, and one study noted cellular debris found on the floor of a container after sonication could possibly be important tissue shaken from the specimen (Callis & Sterchi 1998). Gentle fluid agitation is achieved by low-speed rotation, rocking, stirring, or bubbling air into the solution. Even though findings from various studies are unresolved, agitation is a matter of preference and not harmful as long as tissue components remain intact.
Completion of decalcification
Decalcification endpoint test
There are several methods for testing the completion of decalcification, with two considered to be the most reliable. These are specimen radiography, using an X-ray unit and the chemical method to test acids and EDTA solutions. Another method first used to test nitric acid is a weight loss, weight gain procedure that provides relatively good, quick results with all acids and EDTA (Mawhinney et al. 1984; Sanderson et al. 1995). Although still used, ‘physical’ tests are considered inaccurate and damaging to tissues. Probing, ‘needling’, slicing, bending, or squeezing tissue can create artifacts, e.g. needle tracks, disrupt soft tumor from bone, or cause false-positive microfractures of fine trabeculae, a potential misdiagnosis. The ‘bubble’ test is subjective and dependent on worker interpretation.
Methods for chemical testing of acid decalcifying fluids detect the presence of calcium released from bone. When no calcium is found or the result is negative, decalcification is said to be complete and may entail using one extra change of decalcifier after actual completion. EDTA can be chemically endpoint tested by acidifying the used solution; this forces EDTA to release calcium for precipitation by ammonium oxalate (Rosen 1981).
Calcium oxalate test (Clayden 1952)
Method
Radiography. This is the most sensitive test for detecting calcium in bone or tissue calcification. The method is the same as specimen radiography using a FAXITRON with a manual exposure setting of approximately 1 minute, 30 kV, and Kodak X-OMAT X-ray film on the bottom shelf. It is possible to expose several specimens at the same time. The method is to rinse acid from the sample, carefully place identified bones on waterproof polyethylene sheet on top of the X-ray film, expose according to directions, and leave bones in place until the film is developed and examined for calcifications. Bones with irregular shapes and variable thickness can occasionally mislead workers on interpretation of results. This problem is resolved by comparing the test radiograph to the pre-decalcification specimen radiograph and correlate suspected calcified areas with specimen variations. Areas of mineralization are easily identified, although tiny calcifications are best viewed using a hand-held magnifier. Metal dust particles from saw blades are radio-opaque, sharply delineated fragments that never change in size. These are unaffected by decalcification, appearing as gray specks on the bone surface and can be easily removed. Spicules of metal, metallic paint, or glass forced deep into tissue by a traumatic injury are also sharply delineated but cannot be removed without damaging the tissue. Radiography only indicates the presence of deeper foreign objects, and care must be taken during microtomy to not damage the knife (Fig. 16.5).
Treatment following decalcification
Acids can be removed from tissues or neutralized chemically after decalcification is complete. Chemical neutralization is accomplished by immersing decalcified bone into either saturated lithium carbonate solution or 5–10% aqueous sodium bicarbonate solution for several hours. Many laboratories simply rinse the specimens with running tap water for a period of time. Culling (1974) recommended washing in two changes of 70% alcohol for 12–18 hours before continuing with dehydration in processing, a way to avoid contamination of dehydration solvents even though the dehydration process would remove the acid along with the water.
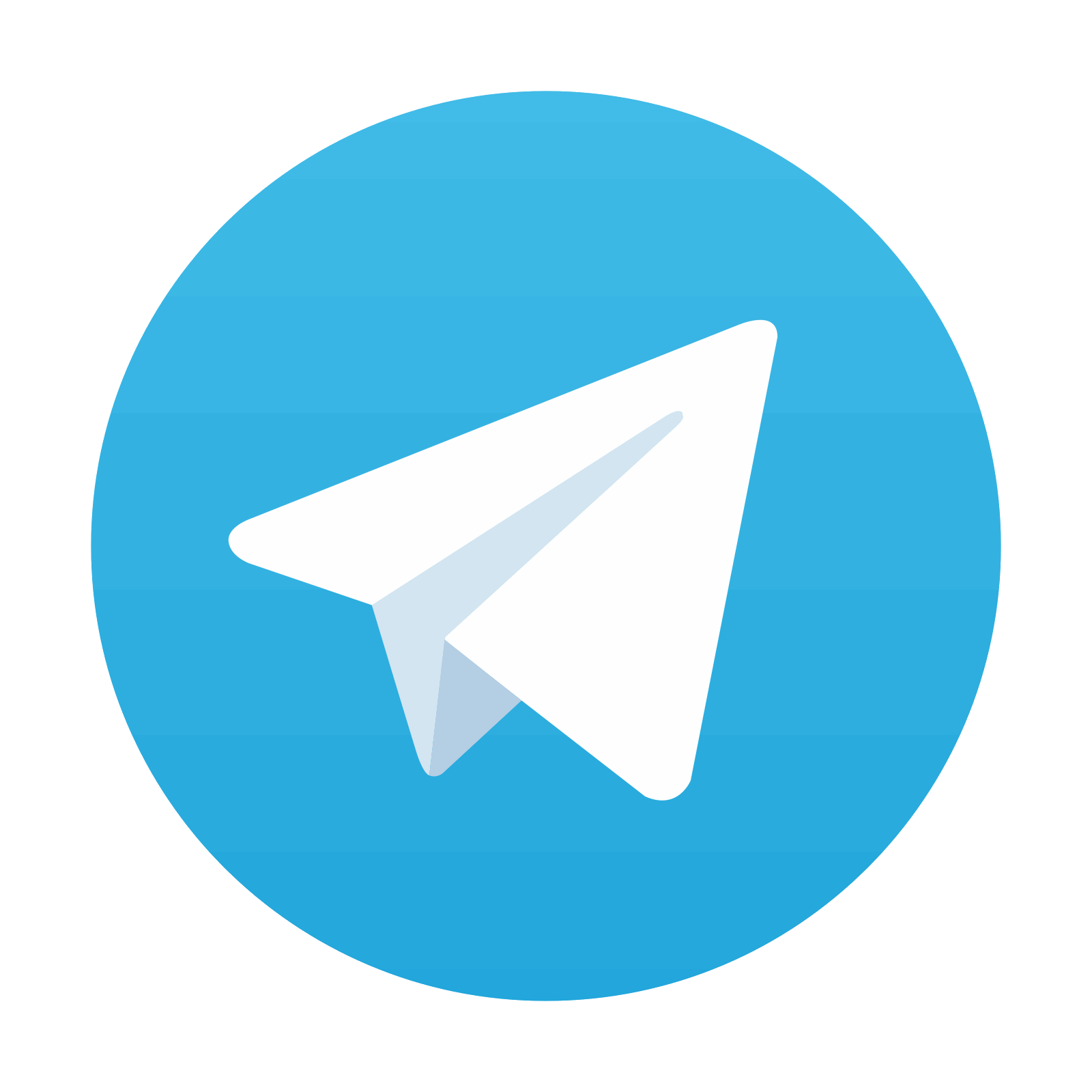
Stay updated, free articles. Join our Telegram channel

Full access? Get Clinical Tree
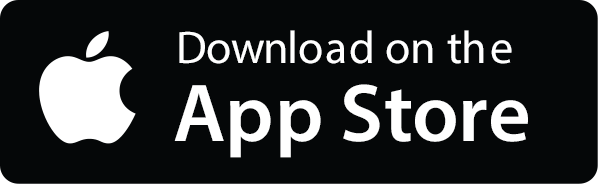
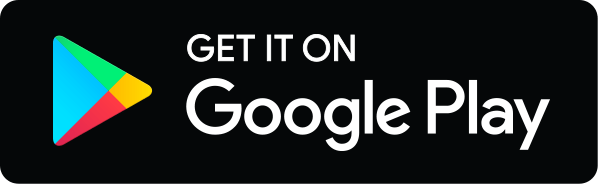