TABLE 48.1 MODELS AT DIFFERENT LEVELS OF BODY COMPOSITION AND RELATED EQUATIONS | ||||||||||||||||||||||||||||||||||||||||||||||||||||||||||||||||||||||||||||||||||||||||||
---|---|---|---|---|---|---|---|---|---|---|---|---|---|---|---|---|---|---|---|---|---|---|---|---|---|---|---|---|---|---|---|---|---|---|---|---|---|---|---|---|---|---|---|---|---|---|---|---|---|---|---|---|---|---|---|---|---|---|---|---|---|---|---|---|---|---|---|---|---|---|---|---|---|---|---|---|---|---|---|---|---|---|---|---|---|---|---|---|---|---|
|
solids, extracellular fluid, and cells. The cellular mass can be divided further into two components, fat and BCM. BCM is the actively metabolizing component at the cellular level (11). The terms fat and lipid are often used interchangeably, although their meanings differ. In body composition assessment, lipid includes all of the biologic matter extracted with lipid solvents. These extracted lipids include triglycerides, phospholipids, and structural lipids that occur in small quantities in vivo (12). In contrast, fats refer to the specific family of lipids consisting of triglycerides (6). Based on reference man (13), approximately 90% of extractable lipids in healthy adults is triglyceride, although this proportion differs with dietary intake and illness (14). The remainder, approximately 10% of the total body lipid (nonfat lipid), are mainly composed of glycerophosphatides and sphingolipids.
current and UBW or IBW may be compared against clinical parameters to determine risk of morbidity and mortality. Body WT typically varies less than ±0.1 kg/day in healthy adults. WT loss of more than 0.5 kg/day indicates negative energy or negative water balance or both. A clinically significant rate of WT loss is considered to be 1% to 2% over 1 week, 5% over 1 month, 7.5% over 3 months, or 10% or greater over 6 months (21). Severity of WT loss may also be evaluated by the absolute WT reduction, which also has prognostic value. An absolute WT of 85% to 95% of UBW (or 80% to 90% of IBW) indicates mild malnutrition, 75% to 84% of UBW (or 70% to 79% of IBW) indicates moderate malnutrition, and 75% or less of UBW (or ≤69% of IBW) indicates severe malnutrition (21). Absolute WT reduction to less than 55% to 60% of IBW places an individual at the limits of starvation (22). In infirm individuals, a WT loss between 10% and 20% of pre-illness WT over 6 months has been associated with functional abnormalities (23), whereas a loss of more than 20% of pre-illness WT suggests significant protein-energy malnutrition (23). The minimum survivable body WT in humans is between 48% and 55% of IBW or a BMI of approximately 13 kg/m2.
TABLE 48.2 BODY MASS INDEX AND WAIST CIRCUMFERENCE CUTOFF POINTS AND RISK OF DISEASE | ||||||||||||||||||||||||||||||||||||||||||||||||
---|---|---|---|---|---|---|---|---|---|---|---|---|---|---|---|---|---|---|---|---|---|---|---|---|---|---|---|---|---|---|---|---|---|---|---|---|---|---|---|---|---|---|---|---|---|---|---|---|
|
TABLE 48.3 FAT FREE BODY COMPOSITION AND DENSITY IN CHILDREN AND YOUTH | |||||||||||||||||||||||||||||||||||||||||||||||||||||||||||||||||||||||||||||||||||||||||||||||||||||||||
---|---|---|---|---|---|---|---|---|---|---|---|---|---|---|---|---|---|---|---|---|---|---|---|---|---|---|---|---|---|---|---|---|---|---|---|---|---|---|---|---|---|---|---|---|---|---|---|---|---|---|---|---|---|---|---|---|---|---|---|---|---|---|---|---|---|---|---|---|---|---|---|---|---|---|---|---|---|---|---|---|---|---|---|---|---|---|---|---|---|---|---|---|---|---|---|---|---|---|---|---|---|---|---|---|---|
|
training (42). Sex and racial differences also exist, and even within a population, considerable interindividual variation (37) invalidates the assumption of FFM chemical constancy. Consequently, multicomponent models (three-component and four-component models; see Table 48.1), which require fewer assumptions because more components are measured, are more accurate than the two-component model. In children and in patients with edema, combining a measure of TBW along with BD significantly improves estimation of FFM; similarly, in elderly patients and in patients with significant bone loss, combining a measure of body mineral with BD gives a more accurate estimate of FFM. When a multicomponent model is not feasible, accuracy can be improved by using a population-specific equation that has been adjusted for the anticipated changes that occur with growth, maturation, and aging (Table 48.4).
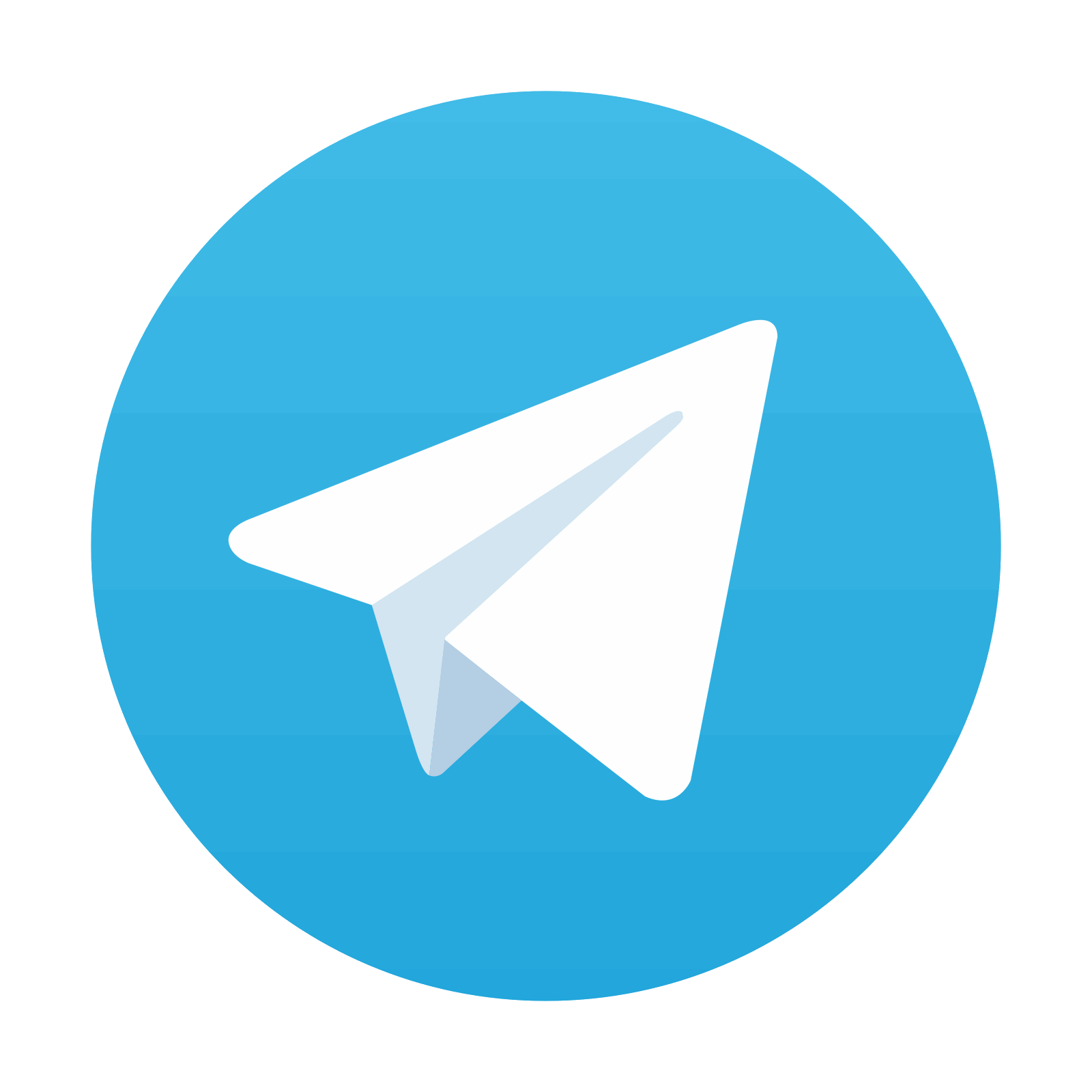
Stay updated, free articles. Join our Telegram channel

Full access? Get Clinical Tree
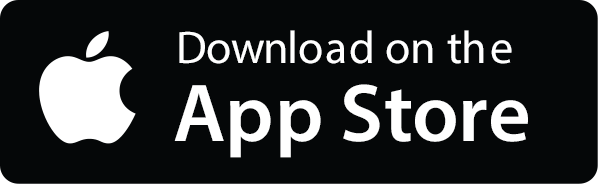
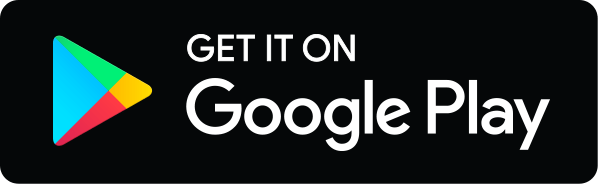