Fig. 10.1
Schematic diagram of biosensor
Biosensor consists of a biological entity such as an enzyme, antibody or nucleic acid that interacts with an analyte and produces a signal that is measured electronically. Each biosensor, therefore, has a biological component that acts as the sensor and an electronic component to transduce and detect the signal. A variety of substances including nucleic acids, proteins (particularly antibodies and enzymes), lectins (plant proteins that bind sugar moieties) and complex materials (organelles, tissue slices, microorganisms) can be used as the biological components. In each case it is the specificity of the biological components for an analyte (or group of related analytes) that makes the biomolecules attractive as sensing component. For example, a single strand of DNA can be used as a biomolecular sensor that will hybridize only to its complementary strands under appropriate conditions. The signal, which can be electrical, optical, or thermal, is converted by means of a suitable transducer into a measurable electrical parameter such as current or voltage. Biosensor probes are attaining increasing sophistication because of the fusion of two technologies: microelectronics and biotechnology. Biosensors provide a useful means for measuring a wide spectrum of analytes (e.g., gases, ions, and organic compounds, or even bacteria) and are suitable for studies of complex microbial environments.
10.2 Overview of Biosensor Technology
The important components of biosensor technology and their interaction in the form of flow chart are represented in Fig. 10.2.
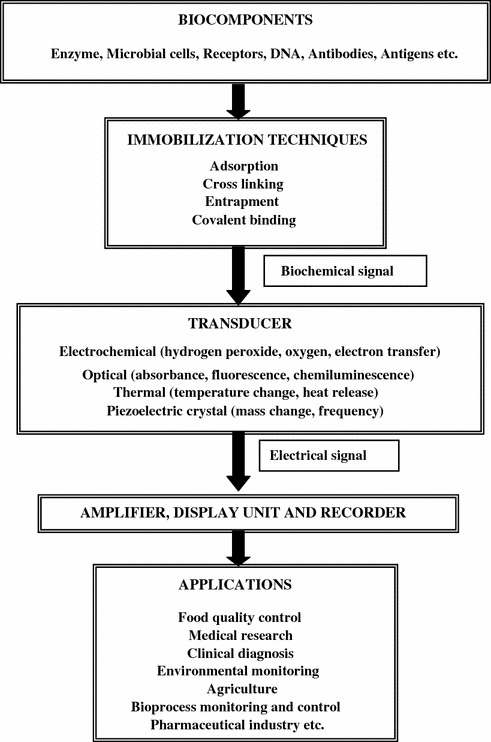
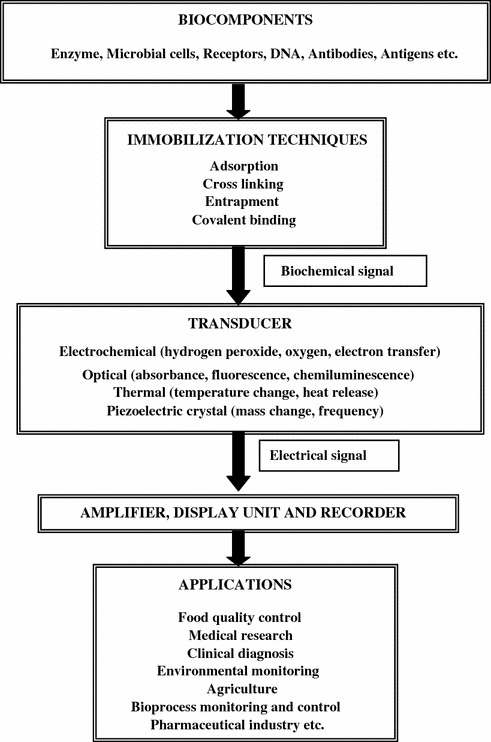
Fig. 10.2
An overview of biosensor technology
10.3 Biological Recognition Elements
The bioactive component could be an enzyme, an antibody, a cell (bacterial and fungal), a tissue slice, a receptor, nucleic acid, or an organelle. It is used to recognize and interact specifically with the analyte. Generally, there are two categories of biosensors, catalytic and affinity:
1.
Catalytic biosensors: In this type of biosensors, biocatalysts such as enzyme, microbiological cells, animal and plant cells, organelles and tissues are used to recognize, bind, and chemically convert a molecule identified by the transducer. These biorecognition elements can be used to analyze substrate, inhibitor, activator, co-factor, and enzyme activity.
2.
Affinity biosensors: In this category the biological element (receptor) binds specifically to the analyte leading to a complex. The transducer reveals the complex and generates an electronic signal. Receptor molecule such as antibodies, nucleic acids, lectins, and hormone receptors are used to bind molecules irreversibly and non-catalytically (Table 10.1).
Table 10.1
Affinity biosensor
Device name | Bio-recognition element | Analyte |
---|---|---|
Immunoassay | Antibody | Antigen |
– | Receptor | Hormone |
– | Enzyme | Inhibitor |
– | Membrane transport protein | Substrate analogue |
Ion selective electrode | Ionophore | Ions |
– | Lectin | Saccharide glycoprotein |
– | Apoenzyme | Prosthetic group |
10.3.1 Enzymes
Enzymes were the first biological components used in biosensors (Clark 1956) and remain by far the most commonly employed elements. Enzymes are the large protein molecules that act as biological catalysts, i.e., they speed up chemical reactions at moderate temperature usually acting on a unique substrate or group of substrates. It is this remarkable specificity that makes enzymatic methods attractive in sensing devices. Enzymes are normally the preferred choice in catalytic-type biosensor. However, in many cases the use of enzymes is currently impractical or impossible. The enzyme may be unstable, may require soluble cofactors, may be difficult to purify, may not involve a readily detectable species, or the analyte may not be substrate for a specific enzyme.
10.3.2 Antibodies
In principle, an antibody-based assay can quantify analyte on the basis of binding reactions between antibody and antigen. It is done from a total of the antibody-antigen complex after 20 min assay operation. To shorten the time for the assay, a new format was developed. The binding reactions between antibody and antigen can be monitored as a time-dependent change of fluorescence signal. The signal change was proportional as a reaction ratio of antibody to the analyte. Thus, it was possible to monitor the analyte concentration in the sample from the signal. As a result of the monitoring, the analytical time was dramatically shortened to less than 2 min.
10.3.3 Receptors
An active biological receptor can be immobilized and stabilized in a polymeric film to construct receptor-based biosensor for determining an analyte of interest in a sample. The receptor-based biosensors include a polymeric film having a biological receptor capable of binding an analyte of interest immobilized therein according to the method of the present invention and an electrical means for determining the presence and quantity of the analyte. In particular, acetylcholine and opiate receptors have been immobilized in a polymeric film made by combining the receptor, a material capable of polymerization (e.g., bovine serum albumin, gelatin) and a polymerizing agent (e.g., glutaraldehyde).
10.3.4 Fragments of Nucleic Acids
An automated optical biosensor system was reported based on fluorescence excitation and detection in the evanescent field of a quartz fiber used to detect 16-mer oligonucleotides in DNA hybridization assays (Abel et al. 1996). For all hybridization experiments a biotinylated capture probe was immobilized using aminosilanized fibers.
10.3.5 Microorganisms
Microorganisms have a number of advantages as biological sensing materials in the fabrication of biosensors. They are present ubiquitously and are able to metabolize a wide range of chemical compounds. Microbes have a great capacity to adapt to adverse conditions and to develop the ability to degrade new molecules with time. They are also amenable for genetic modifications through mutation or recombinant DNA technology and serve as an economical source of intracellular enzymes. A microbial biosensor consists of a transducer in conjunction with immobilized viable or non-viable microbial cells (Riedel 1998). Non-viable cells obtained after permeabilization or whole cells containing periplasmic enzymes have mostly been used as an economical substitute for enzymes. Viable cells make use of the respiratory and metabolic functions of the cell, the analyte to be monitored being either a substrate or an inhibitor of these processes.
Microorganism-based biosensors tend to use one of three primary mechanisms. For the first mechanism, the pollutant is a respiratory substrate. Biosensors that detect biodegradable organic compounds measured as biological oxygen demand (BOD) are the most widely reported of the microorganism-based biosensors using this mechanism. Several of these devices are commercially available from vendors including: Nisshin Electric Co. Ltd., Tokyo; Autoteam, GmbH, Berlin; Prufgeratewrk, Medingen GmbH, Dresden; and Dr. Lange, GmbH, Berlin. The use of these devices has been incorporated into industrial standard methods in Japan.
Another mechanism used for microorganism-based biosensors involves the inhibition of respiration by the analyte of interest. Microbial respiration and its inhibition by various environmental pollutants have been measured both optically and electrochemically. Inherent advantages of these techniques apply primarily to the use of microorganisms as compared to isolated enzymes. These biosensors are relatively inexpensive to construct and can operate over a wide range of pH and temperature. General limitations involve the long assay times including the initial response and return to baseline. These characteristics are primarily determined by the cellular diffusion characteristics that can be modified by using genetically engineered microorganisms. The broad specificity of these biosensors to environmental toxins may be an advantage or disadvantage depending on the intended application. In this respect, these devices might be most applicable for general toxicity screening or in situations where the toxic compounds are well defined, or where there is a desire to measure total toxicity through a common mode of action.
Biosensors have also been developed using genetically engineered microorganisms (GEMs) that recognize and report the presence of specific environmental pollutants. The microorganisms used in these biosensors are typically produced with a constructed plasmid in which genes that code for luciferase or β-galactosidase are placed under the control of a promoter that recognizes the analyte of interest. Because the organism’s biological recognition system is linked to the reporting system, the presence of the analyte results in the synthesis of inducible enzymes which then catalyze reactions resulting in the production of detectable products. With respect to environmental applications, the primary disadvantage for this type of biosensor is the limited number of GEMs which have been constructed to respond to specific environmental pollutants. Nevertheless, reported advances include the development of GEMs involving a variety of bioremediation pathways and mechanisms. GEMs that could report both the metabolic condition of the relevant microorganisms as well as the rates of pollutant breakdown could be very useful (Burlage and Kuo 1994).
10.3.6 Lectin
Lectins are proteins which are highly specific for their sugar moieties. They play a role in biological recognition phenomena involving cells and proteins. Specific mono- and oligosaccharides bind to lectins and provide their attachment to cell membranes. Microbial surface bear many of the sugar residues capable of interacting with lectins. The ability of lectins to react with microbial glycoconjugates renders them to be employed as probes and sorbents for whole cells, mutants, and numerous cellular constituents and metabolites. Lectins are attractive reagents for the clinical diagnostic laboratory because of their diverse specificity, commercial availability, a wide range of molecular weights, and their stability in standard buffers. A lectin-based biosensor is reported for electrochemical assay of glycan expression on living cancer cells which may facilitate the medical diagnosis and treatment in early process of cancer (Zhang et al. 2010).
10.3.7 Membrane Transport Protein
A membrane transport protein is a membrane protein involved in the movement of ions, small molecules, or macromolecules, such as another protein across a biological membrane. Transport proteins are integral membrane proteins; that is they exist within and span the membrane across which they transport substances. The proteins may assist in the movement of substances by facilitated diffusion or active transport. These mechanisms of action are known as carrier-mediated transport. A prototype lactose sensor is reported which consists of a quartz slide covered by a lipid membrane containing the protein lactose permease from Escherichia coli. This protein is a lactose/H+ co-transporter, hence lactose in the external medium initiates lactose/H+ co-transport across the lipid membrane. This leads to a rise in proton concentration in the small volume between the lipid membrane and the quartz surface which can be detected by a pH-sensitive fluorescence dye (Kiefer et al. 1991).
10.4 Transducers
The transducing element of a biosensor is used to convert the biological recognition step into an electrical signal that can be coupled to a microprocessor for control and display. Transducers are mainly divided into four major categories as summarized in Table 10.2.
Table 10.2
Summary of transducer devices
Type | Device | Parameter measured |
---|---|---|
Electronic | Amperometric | Change in current |
Potentiometric | Change in potential | |
Impedance | Change in impedance | |
Thermal | Thermistor | Change in temperature |
Mechanical | Piezo-electric | Change in mass vibrations |
Acoustical | Acoustical | Change in amplitude, phase, or frequency of acoustic wave |
Optical (Colorimetric and Photometric) | Photo-conductive | Change in intensity, colour or emission |
Photo-emissive | Fluorescence or luminescence activation, quenching, and polarization | |
Photo-diodes | ||
Photo-transistors | ||
Fluorescence or Chemiluminiscence |
10.4.1 Electrochemical Transducers
The most common transducer for the construction of a biosensor is electrochemical transducer. The electrochemical device uses a chemical change to measure the input parameter, whereas the output is a varying electrical signal proportional to the measured chemical change. On the basis of output electrical signal the electrochemical transducers may be classified as:
10.4.1.1 Amperometric Biosensors
These biosensors are based upon the resulting steady state limiting current between two electrodes under constant voltage. Most of the glucose biosensors are based upon this principle. It can be either directly or indirectly mediated using suitable redox mediator like ferrocene dye. The electrode potential E will control the concentration of the two redox forms according to Nernst equation:
![$$ {\mathbf{E}} = {\mathbf{E}}^{{\mathbf{0}}} + \, {\mathbf{RT}}/{\mathbf{nF}}\;{\mathbf{ln}}\;\left\{ {\left[ {{\mathbf{ox}}} \right]/\left[ {{\mathbf{red}}} \right]} \right\} $$](/wp-content/uploads/2016/10/A315564_1_En_10_Chapter_Equa.gif)
![$$ {\mathbf{E}} = {\mathbf{E}}^{{\mathbf{0}}} + \, {\mathbf{RT}}/{\mathbf{nF}}\;{\mathbf{ln}}\;\left\{ {\left[ {{\mathbf{ox}}} \right]/\left[ {{\mathbf{red}}} \right]} \right\} $$](/wp-content/uploads/2016/10/A315564_1_En_10_Chapter_Equa.gif)
E 0
is electrode potential at standard conditions
R
is universal gas constant
T
is absolute temperature
F
is Faraday constant
n
is electrons transferred in the reaction
[ox]/[red]
is ratio of oxidized to reduced molecules
10.4.1.2 Potentiometric Biosensors
These biosensors are based upon non-faradic electrodes process with no net current flow. They are based on the rise in electrode potential due to the accumulation of the ionic species produced from the particular biochemical reactions and is measured with a reference electrode. This electrode potential is proportional to analyte concentration in log scale and is also governed by Nernst equation. These sensors have accordingly glass electrode, ion selective electrode, or field effect transistor (FET) as the transducer. Many biosensors for β-lactam antibiotic are based upon this principle.
10.4.1.3 Impedance Biosensors
Impedance biosensors may be used to measure the electrical impedance of an interface in AC steady state with constant DC bias conditions. Mostly this is accomplished by imposing a small sinusoidal voltage at a particular frequency and measuring the resulting current; the process can be repeated at different frequencies. The current–voltage ratio gives the impedance and this technique is known as electrochemical impedance spectroscopy (EIS). The impedance of the electrode-solution interface changes when the target analyte is captured by the probe. EIS can be utilized over a wide frequency range or at a single frequency to study a variety of electrochemical phenomena. Impedance measurement does not require special reagents or labels for operation.
10.4.2 Optical Transducers
Optical sensors, originally developed for oxygen, carbon dioxide, and pH, have been extended to the construction of fluorescent and luminescent opto-electrodes. The selective biocomponent is immobilized at one end of optical fiber, with both the excitation and detection components located at the other end. NAD(P)+/NAD(P)H- dependent dehydrogenases are obvious candidates for these biosensors, because NAD(P)H is known to absorb light strongly at 340 nm and fluorescence at 460 nm. Several NAD(P)+/NAD(P)H-dependent dehydrogenase enzymes exist for a number of food and beverage-related compounds, but the requirement for expensive and unstable cofactors is the major drawback of this technique. Hydrogen peroxide fiber-optic sensor based on absorbance or chemiluminescence has also been developed for various analytes using oxidases. Optical transducers can also take advantages of affinity interactions between antibodies and antigens to screen for microbial contaminants (Mehrvar et al. 2000).
10.4.3 Thermal Transducers
This device is regarded as a small microcalorimeter with immobilized biocomponents, packed in a small column in proximity to the heat sensing transducer, commonly a thermistor. Most enzyme or microorganism catalyzed reactions are accompanied by considerable heat evolution (20–100 kJ/mol), hence this device has a broader applicability than other transducers. The commercial enzyme thermistor claims to detect temperature change in the range 0.0001–0.05 °C. The sensitivity of this technique is equivalent to the substrate concentration of 10−5 M. Thermal transducers have also been applied for the detection of antibody-antigen interactions. This technique is known as the thermometric enzyme linked immunosorbent assay, however its use is sophisticated and expensive.
10.4.4 Piezoelectric Transducer (Quartz Microbalance)
A quartz crystal can oscillate when partially or completely immersed in a liquid, and if the physiochemical properties of the liquid (such as viscosity, density, and conductivity) are defined, a change in the weight of the quartz crystal can be detected by measuring its vibration frequency. Biologically active materials such as antibodies, enzymes, and antigens have been used as active coatings. For the past two decades, intensive research has been directed to developing piezoelectric sensors for a variety of applications. However, non-specific binding and poor sensitivity are the two major drawbacks of piezoelectric transduction (Sochaczewski et al. 1990).
10.5 Immobilization Techniques
An efficient and effective biosensor requires intimate contact between its component parts, normally achieved by restricting the biomolecule, cell, or organelles to a thin layer on or near the transducer surface. The processes employed in achieving this restriction are collectively known as immobilization techniques, defined according to the recommendation of standardization of nomenclature in enzyme technology as:
1.
Entrapment with a membrane, gel, or microcapsule .
2.
Physical or chemical adsorption.
3.
Cross-linking between molecules.
4.
Covalent bonding to soluble or insoluble supports.
The localization of biological components at the transducer confers several advantages:
1.
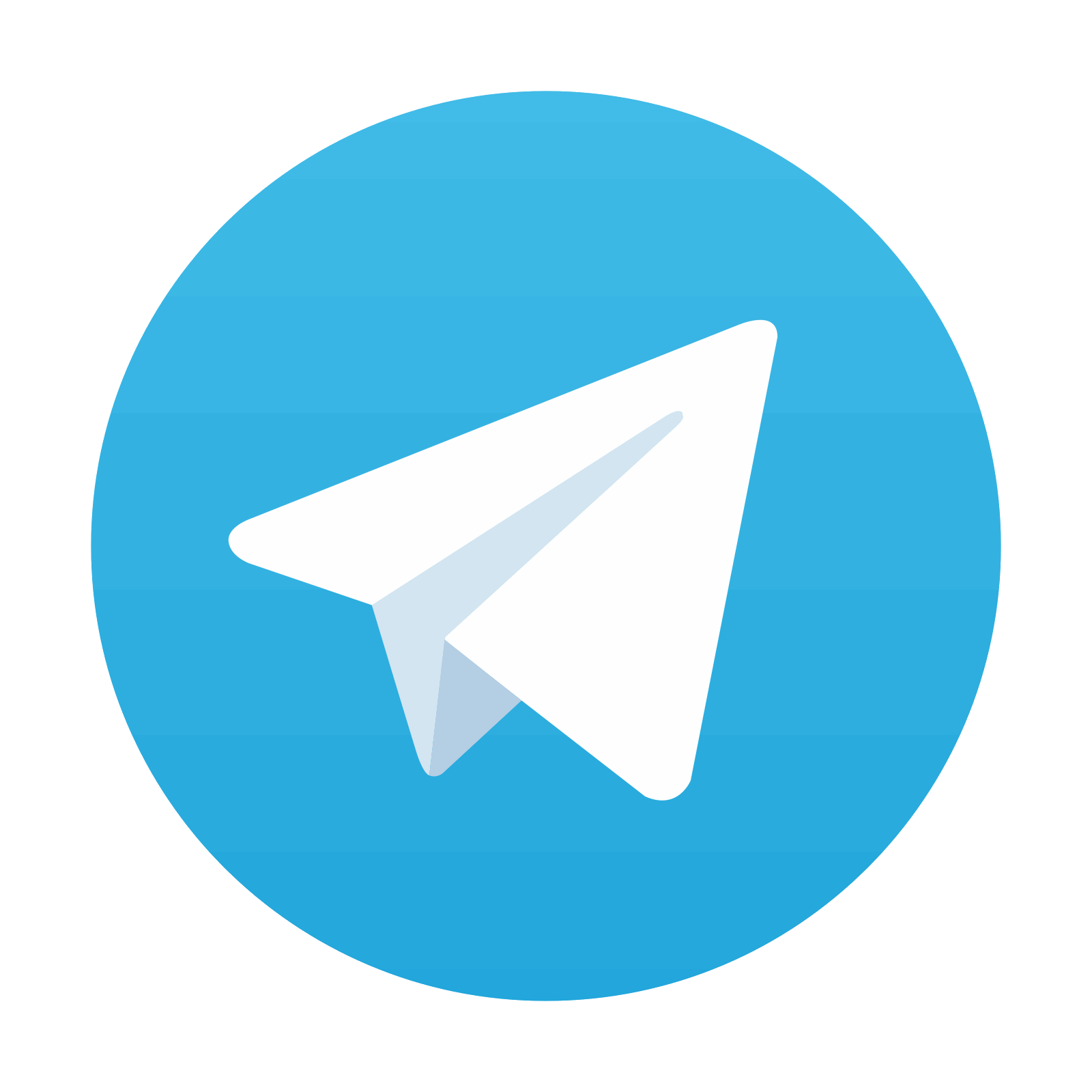
Enables recovery of the biocomponent without dilution or contamination.
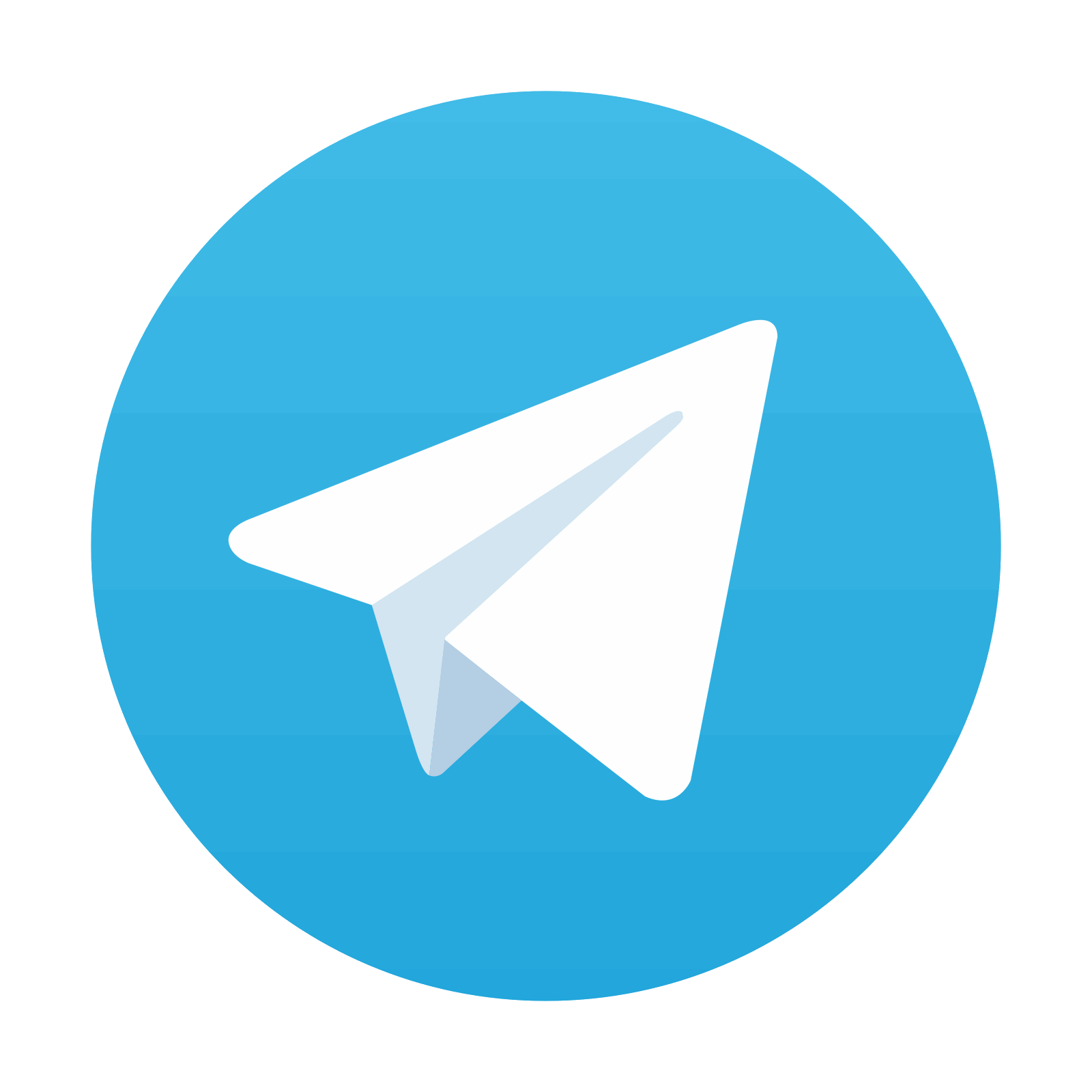
Stay updated, free articles. Join our Telegram channel

Full access? Get Clinical Tree
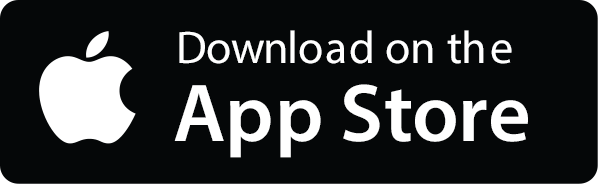
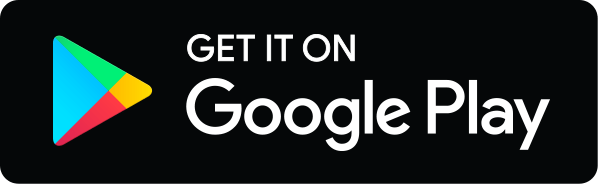
