12
Biologic Oxidation
Kathleen M. Botham, PhD, DSc & Peter A. Mayes, PhD, DSc
OBJECTIVES
After studying this chapter, you should be able to:
Understand the meaning of redox potential and explain how it can be used to predict the direction of flow of electrons in biologic systems.
Identify the four classes of enzymes (oxidoreductases) involved in oxidation and reduction reactions.
Describe the action of oxidases and provide examples of where they play an important role in metabolism.
Indicate the two main functions of dehydrogenases and explain the importance of NAD- and riboflavin-linked dehydrogenases in metabolic pathways such as glycolysis, the citric acid cycle, and the respiratory chain.
Identify the two types of enzymes classified as hydroperoxidases; indicate the reactions they catalyze and explain why they are important.
Give the two steps of reactions catalyzed by oxygenases and identify the two subgroups of this class of enzymes.
Appreciate the role of cytochrome P450 in drug detoxification and steroid synthesis.
Describe the reaction catalyzed by superoxide dismutase and explain how it protects tissues from oxygen toxicity.
BIOMEDICAL IMPORTANCE
Chemically, oxidation is defined as the removal of electrons and reduction as the gain of electrons. Thus, oxidation is always accompanied by reduction of an electron acceptor. This principle of oxidation-reduction applies equally to biochemical systems and is an important concept underlying understanding of the nature of biologic oxidation. Note that many biologic oxidations can take place without the participation of molecular oxygen, eg, dehydrogenations. The life of higher animals is absolutely dependent upon a supply of oxygen for respiration, the process by which cells derive energy in the form of ATP from the controlled reaction of hydrogen with oxygen to form water. In addition, molecular oxygen is incorporated into a variety of substrates by enzymes designated as oxygenases; many drugs, pollutants, and chemical carcinogens (xenobiotics) are metabolized by enzymes of this class, known as the cytochrome P450 system. Administration of oxygen can be lifesaving in the treatment of patients with respiratory or circulatory failure.
FREE ENERGY CHANGES CAN BE EXPRESSED IN TERMS OF REDOX POTENTIAL
In reactions involving oxidation and reduction, the free energy change is proportionate to the tendency of reactants to donate or accept electrons. Thus, in addition to expressing free energy change in terms of ΔG0’ (Chapter 11), it is possible, in an analogous manner, to express it numerically as an oxidation-reduction or redox potential (E’0). The redox potential of a system (E0) is usually compared with the potential of the hydrogen electrode (0.0 V at pH 0.0). However, for biologic systems, the redox potential (E’0) is normally expressed at pH 7.0, at which pH the electrode potential of the hydrogen electrode is -0.42 V. The redox potentials of some redox systems of special interest in mammalian biochemistry are shown in Table 12-1. The relative positions of redox systems in the table allow prediction of the direction of flow of electrons from one redox couple to another.
TABLE 12–1 Some Redox Potentials of Special Interest in Mammalian Oxidation Systems
Enzymes involved in oxidation and reduction are called oxidoreductases and are classified into four groups: oxidases, dehydrogenases, hydroperoxidases, and oxygenases.
OXIDASES USE OXYGEN AS A HYDROGEN ACCEPTOR
Oxidases catalyze the removal of hydrogen from a substrate using oxygen as a hydrogen acceptor.* They form water or hydrogen peroxide as a reaction product (Figure 12–1).
FIGURE 12–1 Oxidation of a metabolite catalyzed by an oxidase (A) forming H2O and (B) forming H2O2.
Some Oxidases Contain Copper
Cytochrome oxidase is a hemoprotein widely distributed in many tissues, having the typical heme prosthetic group present in myoglobin, hemoglobin, and other cytochromes (Chapter 6). It is the terminal component of the chain of respiratory carriers found in mitochondria (Chapter 13) and transfers electrons resulting from the oxidation of substrate molecules by dehydrogenases to their final acceptor, oxygen. The action of the enzyme is blocked by carbon monoxide, cyanide, and hydrogen sulfide, and this causes poisoning by preventing cellular respiration. It has also been termed “cytochrome a3.” However, it is now known that the heme a3 is combined with another heme, heme a, in a single protein to form the cytochrome oxidase enzyme complex, and so it is more correctly termed cytochrome aa3. It contains two molecules of heme, each having one Fe atom that oscillates between Fe3+ and Fe2+ during oxidation and reduction. Furthermore, two atoms of Cu are present, each associated with a heme unit.
Other Oxidases Are Flavoproteins
Flavoprotein enzymes contain flavin mononucleotide (FMN) or flavin adenine dinucleotide (FAD) as prosthetic groups. FMN and FAD are formed in the body from the vitamin riboflavin (Chapter 44). FMN and FAD are usually tightly—but not covalently—bound to their respective apoenzyme proteins. Metalloflavoproteins contain one or more metals as essential cofactors. Examples of flavoprotein enzymes include L-amino acid oxidase, an FMN-linked enzyme found in kidney with general specificity for the oxidative deamination of the naturally occurring L-amino acids; xanthine oxidase, which contains molybdenum and plays an important role in the conversion of purine bases to uric acid (Chapter 33), and is of particular significance in uricotelic animals (Chapter 28); and aldehyde dehydrogenase, an FAD-linked enzyme present in mammalian livers, which contains molybdenum and non-heme iron and acts upon aldehydes and N-heterocyclic substrates. The mechanisms of oxidation and reduction of these enzymes are complex. Evidence suggests a two-step reaction as shown in Figure 12–2.
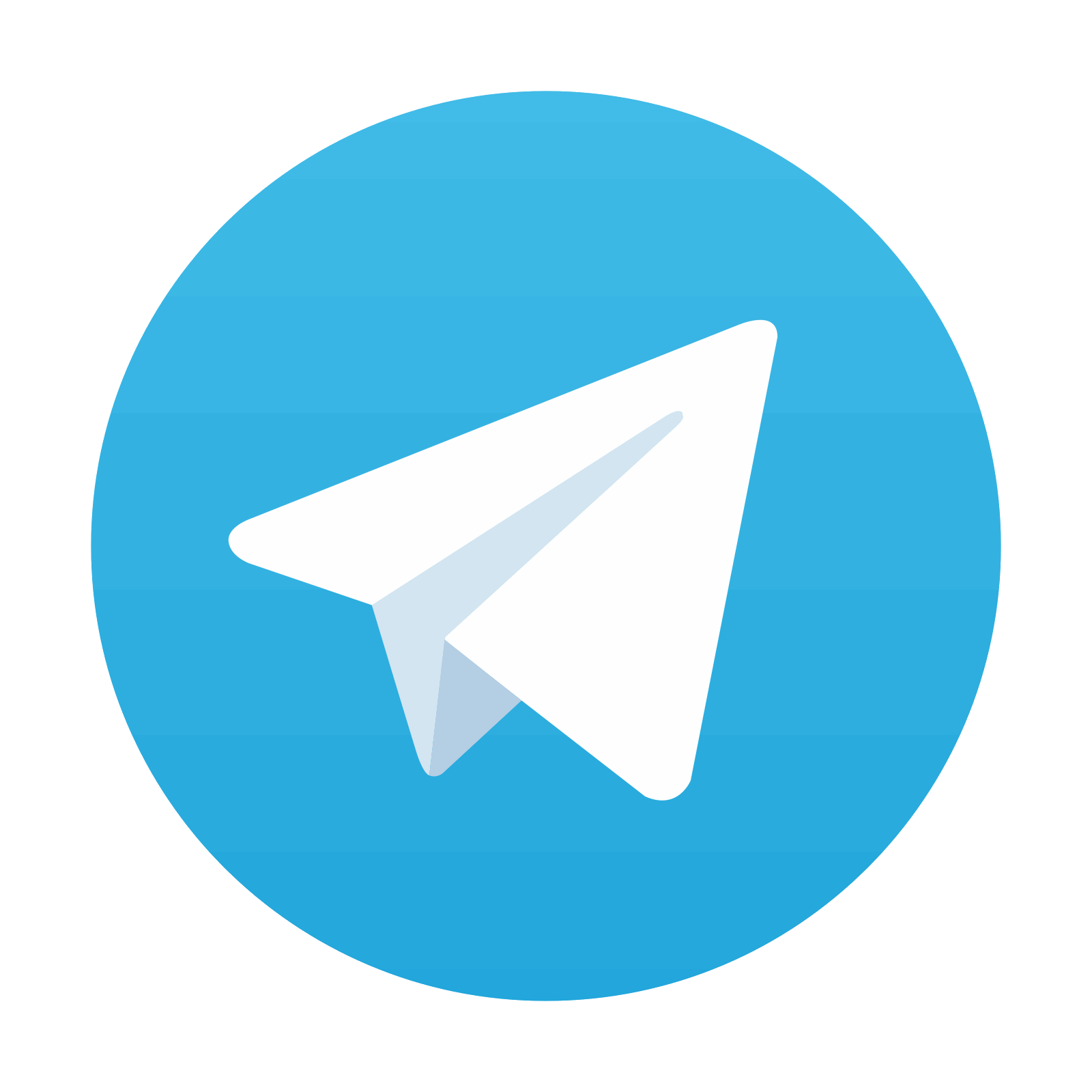
Stay updated, free articles. Join our Telegram channel

Full access? Get Clinical Tree
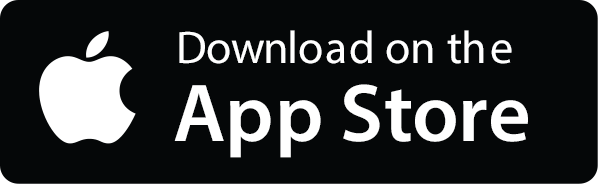
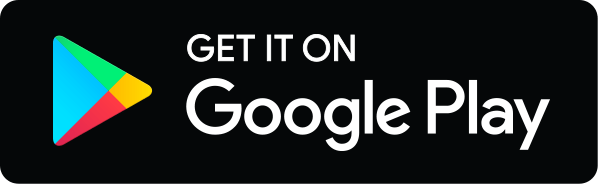