Figure 31.1. Age of Onset of Asthma. Most asthma begins in early childhood. This figure depicts the incidence of asthma among the general population in Rochester, Minnesota in the 1980s. Hatched bars = female; solid bars = male. SOURCE: Adapted from Yunginger JW, et al., Am Rev Respir Dis. 1992;146:888.
Historical features that point to a diagnosis of asthma include the intermittent nature of symptoms, characteristic triggers, and a favorable response to appropriate therapy. The following points bear emphasis. First, the exercise-induced bronchoconstriction of asthma characteristically occurs immediately after a short period (e.g., 5 min) of exercise and is characteristically worse if the air breathed during exercise is cold. Dyspnea on exertion, such as breathlessness climbing stairs or walking up an incline, should be distinguished from exercise-induced bronchoconstriction. Second, allergic triggers are unique to atopic asthma. For example, the patient who develops cough, wheezing, and chest tightness on exposure to cats almost surely has asthma. However, other triggers of asthmatic symptoms are nonspecific (e.g., exposure to smoke or strong fumes) and may be precipitants of symptoms in a variety of other respiratory diseases as well.
Third, for most patients with asthma, inhaled quick-acting bronchodilators bring rapid and effective relief of symptoms, if perhaps only temporarily, and typically a course of oral corticosteroids is dramatically successful in restoring normal breathing. Patients who report that they have tried these interventions in the past but found little benefit frequently will be found not to have asthma.
The characteristic diffuse, musical wheezing of asthma, prominent on exhalation, is familiar to most clinicians. It is worth remembering, however, that not all wheezing sounds are due to asthma. The single-toned, end-expiratory wheezing of COPD, repeated with little change at the end of each exhalation following deep breaths, can readily be distinguished from asthma. So, too, a unilateral or focal wheeze raises the possibility of localized endobronchial obstruction (e.g., by a bronchial neoplasm or aspirated foreign body) and is atypical of asthma. Likewise, the low-pitched wheezing of persistent airway mucus, often associated with palpable vibration of the chest wall (tactile fremitus) and referred to as rhonchi, may indicate bronchiectasis or aspiration. This physical finding is less likely to suggest a diagnosis of asthma.
If after history and physical examination the diagnosis of asthma remains in doubt, confirmation (or exclusion) of the diagnosis is established by pulmonary function testing. Asthma is defined in terms of variable airflow obstruction, observed either on measurements made at multiple points over time or in response to bronchodilator administration. Although peak flow measurements are useful for screening purposes (e.g., in assessing for workplace-induced symptoms of occupational asthma) and for monitoring established asthma, the best test for identifying and quantifying expiratory airflow obstruction is spirometry. A reduced 1-second forced expiratory volume (FEV1) to forced vital capacity (FVC) ratio (indicative of airflow obstruction) and a reduced FEV1 that increases by more than 12% following bronchodilator (indicative of reversible airflow obstruction) is typical of asthma. The larger the increase in FEV1 (e.g., >15–20% increase following bronchodilator) the more likely the diagnosis of asthma and less likely the diagnosis of other obstructive lung diseases. A patient whose FEV1 or peak expiratory flow (PEF) is consistently normal even when measurements are made during active respiratory symptoms probably does not have asthma.
In fully equipped pulmonary function testing laboratories, methacholine bronchoprovocation testing can be performed to evaluate for inducible bronchoconstriction in patients suspected of asthma whose lung function is repeatedly normal at the time of testing. Inhaled methacholine is administered in incremental doses, and spirometry is repeated after each dose. A fall in FEV1 in response to inhaled methacholine of at least 20% from baseline is found in nearly all patients with asthma; a fall in FEV1 of ≥20% in response to a low provocative concentration of methacholine (PC20 ≤8 mg/mL) is relatively specific for asthma and uncommon in other obstructive lung diseases. Most helpful, failure to demonstrate bronchial hyperresponsiveness (FEV1 does not fall to less the 80% of the baseline value even at the highest concentration of methacholine administered) excludes a diagnosis of asthma with at least 95% certainty (Figure 31.2).
To date, measurement of the concentration of nitric oxide (NO) in the exhaled breath has proved to be the most useful biomarker of asthmatic airway inflammation. Commercially available devices can accurately record the concentration of exhaled NO in parts per billion (ppb) during a sustained exhalation at a steady expiratory flow. Among patients not taking antiinflammatory medications (particularly inhaled steroids), values of >20 ppb argue against a diagnosis of asthma, whereas values <40 ppb are suggestive of asthma even in the absence of airflow obstruction at the time of the measurement.
Figure 31.2. Bronchial Hyperresponsiveness in Asthma. Lung function as the 1-second forced expiratory volume (FEV1) is expressed on the ordinate as the percentage of normal. Normal bronchial responsiveness is shown as the top line; the FEV1 did not fall by >20% from baseline in response to the highest dose of the provocative agent (such as methacholine). Three examples of bronchial hyperresponsiveness are shown, consistent with three patients with asthma. They differ in the degree of their bronchial hyperresponsiveness. The patient whose FEV1 falls to <20% below baseline in response to the smallest dose of provocative stimulus (line furthest to the left) has the most bronchial hyperresponsiveness, quantified by calculation of the provocative concentration of stimulus causing a 20% fall in FEV1 (PC20).
REDUCING ENVIRONMENTAL TRIGGERS OF ASTHMA
Some of the triggers of asthma, such as exercise, cause only transient bronchoconstriction. Others, however, cause both airway smooth muscle constriction and increased airway inflammation. Examples include allergen exposure (in the sensitized atopic patient), viral respiratory tract infections, and noxious chemicals such as ozone or cigarette smoke. The resulting increase in airway inflammation is associated with heightened bronchial responsiveness that, even after a single exposure, may last for days. Therefore, in the atopic patient allergen exposure can precipitate not only an asthmatic attack but also worsened asthma in general.
In support of this hypothesis is the observation from the Cooperative Inner-City Asthma Study that among children living in poverty in inner-city communities, those with allergic sensitivities (demonstrated by positive allergy skin tests) and intense allergic exposures in the home environment (quantified by measuring antigen levels in dust vacuumed from the children’s bedrooms) had more asthmatic symptoms and need for urgent asthma care than children without the combination of allergic sensitivity and high levels of allergen exposure. In this study, the most common offending allergen was cockroach antigen, but the principle confirmed by this study applies to all of the aeroallergens to which asthmatic patients may be sensitive.
Achieving good asthma control, therefore, begins with asking your patient about allergic (or noxious) exposures in the home or workplace, including cigarette smoking. The common allergens important in stimulating asthmatic inflammation are relatively few, leading to a short list of questions: Do you have a pet cat or dog or other furry animal (or bird)? Does your home have mold, particularly in a damp basement or bathroom? Do you have cockroaches, rats, or mice infesting your home? Does your asthma worsen when you dust or vacuum at home? Is there a large seasonal variation to your asthma, particularly with worsening in the spring (tree pollens), summer (grass pollens), or fall (weed pollens)?
With some patients you (or they) may suspect an allergic component but be uncertain based solely on their prior experiences. In this circumstance further testing is indicated and may include skin or blood testing for allergic sensitivities. The latter utilizes radioallergosorbent (RAST) testing to measure the amount of circulating immunoglobulin E (IgE) to specific allergens. For instance, you can order RAST testing for measurement of the circulating IgE to cat dander, dog dander, dust mite, cockroach, and common molds (e.g., Aspergillus and Alternaria). Positive test results combined with a consistent history point to a role for allergic exposure in worsening asthma control.
Although it is intuitively logical, until recently there has been little convincing evidence to demonstrate that reducing allergic exposures leads to improved asthma control in the allergic patient. However, in 2004 the Inner-City Asthma Study Group published the results of a randomized trial among impoverished households living in several inner-city communities. In one group, an intervention team helped families reduce allergen exposure in their homes; in the other group general encouragement to do so was offered, without equipment or educational reinforcement to help achieve those ends. Although symptoms lessened in both groups, presumably as the result of their participation in a formal research study, over the first year the intervention group had significantly greater improvement (fewer days with active asthma symptoms) than the control group. The interventions were conducted and reinforced over 1 year; of interest, the greater improvement in the intervention group was sustained for the entire 2 years of follow-up observations.
The interventions to reduce allergen exposures in these homes included the following: vacuum cleaners equipped with high-efficiency particulate air (HEPA) filters; stand-alone room HEPA filters for children with pets or dust mite allergy; cockroach and other pest extermination; and dust-impermeable covers for the children’s mattress and pillows. Cigarette smokers were encouraged to quit smoking or not to smoke cigarettes indoors. A reasonable supposition is that if environmental control measures can help improve asthma control in these inner-city environments among families with low socioeconomic means and limited educational resources, they will likely be at least equally effective among patients with greater opportunities for their successful implementation.
TREATING WITH APPROPRIATE MEDICATIONS
The medications used to treat asthma are categorized as quick relievers or controllers. In the former category are the inhaled beta-agonist bronchodilators with a quick onset of action; in the latter category are inhaled corticosteroids, leukotriene-modifying drugs, long-acting inhaled beta-agonist bronchodilators, and, most recently, anti-IgE monoclonal antibody. Theophylline and inhaled chromones (e.g., cromolyn and nedocromil) have little role in modern asthma management and are not discussed in any detail in this review.
It has been an adage of asthma care for many years that the medications used to treat asthma should be individually tailored according to the severity of each patient’s asthma. The Guidelines for the Diagnosis and Management of Asthma released by the Expert Panel 3 in 2007 confirms this recommendation but refocuses our thinking to consider the adequacy of asthma control in adjusting medications (i.e., consider the activity of recent asthmatic symptoms, level of lung function, and the risk of a future asthmatic attack while minimizing medication side effects). These guidelines recommend that treatment be escalated along a six-step sequence until good asthma control is achieved (Figure 31.3).
Figure 31.3. Step-Care Approach to Achieving Asthma Control. This chart displays a step-care approach to achieving asthma control in children >12 years old and in adults. SOURCE: Expert Panel 3 of the National Asthma Education and Prevention Program, www.nhlbi.nih.gov/guidelines/asthma
Step 1 is the recommended treatment for patients with intermittent asthma (symptoms necessitating quick-relief bronchodilator no more often than two days per week; nocturnal awakenings due to asthma no more often than two days per month; lung function within the normal range; and no more than one attack of asthma within the past year requiring a course of oral steroids). The previously used term “mild intermittent asthma” has been modified to “intermittent asthma” to emphasize the point that even patients with intermittent asthma can suffer severe and life-endangering asthmatic attacks.
The treatment recommended at Step 1 of asthma care is an inhaled quick-acting beta-agonist bronchodilator used as needed for relief of symptoms. It is also helpful to remind (or inform) patients that they can take their quick-acting bronchodilator 10–15 minutes prior to physical exertion to prevent exercise-induced bronchoconstriction. The quick-acting beta agonists of short duration of action (4–6 hours) are albuterol, pirbuterol, and levalbuterol. All of these short-acting beta-agonist (SABA) bronchodilators have similar activity and side-effects profiles. Pirbuterol (Maxair) comes in a breath-actuated metered-dose inhaler, where medication is released at the mouthpiece only in response to inspiratory flow detected at the beginning of an inhalation. Its manufacture stopped in 2013. Levalbuterol contains a single (dextrorotatory) stereoisomer from the racemic mixture that is albuterol; at half the dose (45 µg/puff), it has the same activity as albuterol.
Traditional metered-dose inhalers utilized chlorofluorocarbons (CFCs) as their propellant. Beginning in January 2009, use of CFC propellants has been gradually phased out. The newer metered-dose inhalers contain the propellant hydrofluoroalkane (HFA). No generic albuterol-HFA metered-dose inhaler is currently available; brand name albuterol-HFA inhalers are ProAir, Proventil, and Ventolin. The force of the medication plume as it exits the inhaler is slower with the HFA propellant compared to the traditional CFC-driven devices (Figure 31.4). The medication consequently causes a different sensation in the pharynx; it feels less forceful and less cold. Nonetheless, patients can be reassured that the medication effects are identical regardless of the propellant used for its delivery.
Figure 31.4. Albuterol Plumes with Different Propellants. This image provides a visual display of the difference in medication plume between albuterol delivered via a metered-dose inhaler with hydrofluoroalkane (HFA) propellant and one with traditional chlorofluorocarbon (CFC) propellant.
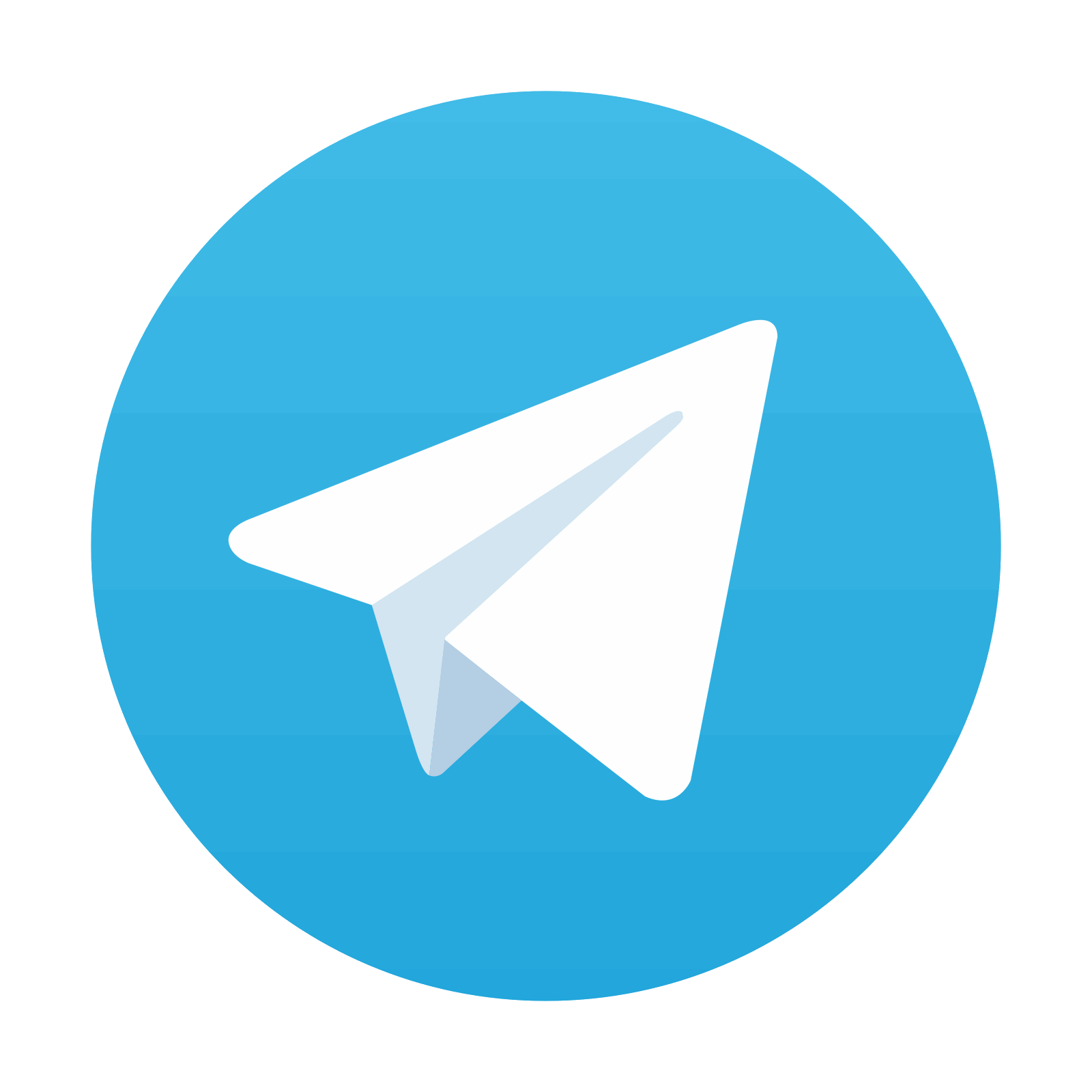
Stay updated, free articles. Join our Telegram channel

Full access? Get Clinical Tree
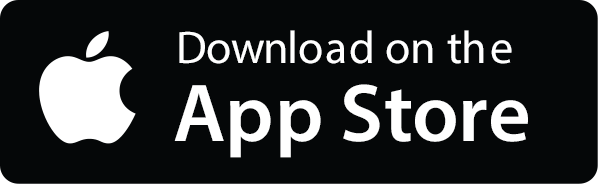
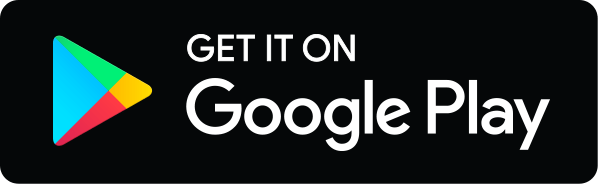