INTRODUCTION
The arthropod-borne viruses (arboviruses) and rodent-borne viruses represent ecologic groupings of viruses with complex transmission cycles involving arthropods or rodents. These viruses have diverse physical and chemical properties and are classified in several virus families.
Arboviruses and rodent-borne viruses are classified among the Arenaviridae, Bunyaviridae, Flaviviridae, Reoviridae, and Togaviridae families. The African hemorrhagic fever viruses are classified in the Filoviridae family (Table 38-1, Figure 38-1). A number of the diseases described here are considered emerging infectious diseases (see Chapter 29).
Taxonomic Classification | Important Arbovirus and Rodent-Borne Virus Members | Virus Properties |
---|---|---|
Arenaviridae | ||
Genus Arenavirus | New World: Chapare, Guanarito, Junin, Machupo, Sabia, and Whitewater Arroyo viruses. Old World: Lassa, Lujo, and lymphocytic choriomeningitis viruses. Rodent borne | Spherical, 50–300 nm in diameter (mean, 110–130 nm). Genome: double-segmented, negative-sense and ambisense, single-stranded RNA, 10–14 kb in overall size. Virion contains a transcriptase. Four major polypeptides. Envelope. Replication: cytoplasm. Assembly: incorporate ribosomes and bud from plasma membrane |
Bunyaviridae | ||
Genus Orthobunyavirus | ||
Anopheles A and B, Bunyamwera, California encephalitis, Guama, La Crosse, Oropouche, and Turlock viruses. Arthropod borne (mosquitoes) | Spherical, 80–120 nm in diameter. Genome: triple-segmented, negative-sense or ambisense, single-stranded RNA, 11–19 kb in total size. Virion contains a transcriptase. Four major polypeptides. Envelope. Replication: cytoplasm. Assembly: budding into the golgi | |
Genus Hantavirus | Hantaan virus (Korean hemorrhagic fever), Seoul virus (hemorrhagic fever with renal syndrome), Sin Nombre virus (hantavirus pulmonary syndrome). Rodent borne | |
Genus Nairovirus | Crimean-Congo hemorrhagic fever, Nairobi sheep disease, and Sakhalin viruses. Arthropod borne (ticks) | |
Genus Phlebovirus | Heartland virus, Lone Star virus, Rift Valley fever virus, sandfly (Phlebotomus) fever viruses, severe fever with thrombocytopenia syndrome virus (SFTSV,) and Uukuniemi viruses. Arthropod borne (mosquitoes, sandflies, ticks) | |
Filoviridae | ||
Genus Marburgvirus | Marburg viruses | Long filaments, 80 nm in diameter × varying length (>10,000 nm), although most average ~1000 nm. Genome: negative-sense, nonsegmented, single-stranded RNA, 19 kb in size. Seven polypeptides. Envelope. Replication: cytoplasm. Assembly: budding from plasma membrane |
Genus Ebolavirus | Ebola viruses | |
Flaviviridae | ||
Genus Flavivirus | Brazilian encephalitis (Rocio virus), dengue, Japanese B encephalitis, Kyasanur Forest disease, louping ill, Murray Valley encephalitis, Omsk hemorrhagic fever, Powassan virus, St. Louis encephalitis, West Nile fever, and yellow fever viruses. Arthropod borne (mosquitoes, ticks) | Spherical, 40–60 nm in diameter. Genome: positive-sense, single-stranded RNA, 11 kb in size. Genome RNA infectious. Envelope. Three structural polypeptides, two glycosylated. Replication: cytoplasm. Assembly: within endoplasmic reticulum. All viruses serologically related |
Reoviridae | ||
Genus Coltivirus | Colorado tick fever virus. Arthropod borne (ticks, mosquitoes) | Spherical, 60–80 nm in diameter. Genome: 10–12 segments of linear, double-stranded RNA, 16–27 kbp total size. No envelope. Ten to 12 structural polypeptides. Replication and assembly: cytoplasm (see Chapter 37) |
Genus Orbivirus | African horse sickness and bluetongue viruses. Arthropod borne (mosquitoes) | |
Togaviridae | ||
Genus Alphavirus | Chikungunya, Eastern, Western, and Venezuelan equine encephalitis viruses, Mayaro, O’Nyong-nyong, Ross River, Semliki Forest, and Sindbis viruses. Arthropod borne (mosquitoes) | Spherical, 70 nm in diameter, nucleocapsid has 42 capsomeres. Genome: positive-sense, single-stranded RNA, 11–12 kb in size. Envelope. Three or four major structural polypeptides, two glycosylated. Replication: cytoplasm. Assembly: budding through host cell membranes. All viruses serologically related |
FIGURE 38-1
Electron micrographs of typical arboviruses and rodent-borne viruses. A: An alphavirus, Semliki Forest virus (Togaviridae). B: A representative member of Bunyaviridae, Uukuniemi virus. C: An arenavirus, Tacaribe virus (Arenaviridae). D: Ebola virus (Filoviridae). (Courtesy of FA Murphy and EL Palmer.)
The arboviruses are transmitted by bloodsucking arthropods from one vertebrate host to another. The vector acquires a lifelong infection through the ingestion of blood from a viremic vertebrate. The viruses multiply in the tissues of the arthropod without evidence of disease or damage. Some arboviruses are maintained in nature by transovarian transmission in arthropods.
The major arbovirus diseases worldwide are yellow fever, dengue, Japanese B encephalitis, St. Louis encephalitis, western equine encephalitis, eastern equine encephalitis, tick-borne encephalitis, West Nile fever, and sandfly fever. In the United States the most important arboviral infections are La Crosse encephalitis, West Nile fever, St. Louis encephalitis, eastern equine encephalitis, and western equine encephalitis.
Rodent-borne viral diseases are maintained in nature by direct intraspecies or interspecies transmission from rodent to rodent without participation of arthropod vectors. Viral infection is usually persistent. Transmission occurs by contact with body fluids or excretions.
Major rodent-borne viral diseases include hantavirus infections, Lassa fever, and South American hemorrhagic fevers. In the United States, the most important rodent-borne diseases are hantavirus pulmonary syndrome (HPS) and Colorado tick fever. Also considered here are the African hemorrhagic fevers—Marburg and Ebola. Their reservoir hosts are unknown but are suspected to be rodents or bats.
HUMAN ARBOVIRUS INFECTIONS
There are several hundred arboviruses, of which about 100 are known human pathogens. Those infecting humans are all believed to be zoonotic, with humans the accidental hosts who play no important role in the maintenance or transmission cycle of the virus. Exceptions are urban yellow fever, chikungunya, and dengue. Some of the natural cycles are simple and involve infection of a nonhuman vertebrate host (mammal or bird) transmitted by a species of mosquito or tick (eg, jungle yellow fever, Colorado tick fever). Others, however, are more complex. For example, tick-borne encephalitis can occur after ingestion of raw milk from goats and cows infected by grazing in tick-infested pastures where a tick–rodent cycle is occurring.
Individual viruses were sometimes named after a disease (dengue, yellow fever) or after the geographic area where the virus was first isolated (St. Louis encephalitis, West Nile fever). Arboviruses are found in all temperate and tropical zones, but they are most prevalent in the tropics with its abundance of animals and arthropods.
Diseases produced by arboviruses may be divided into three clinical syndromes: (1) fevers of an undifferentiated type with or without a maculopapular rash and usually benign; (2) encephalitis (inflammation of the brain), often with a high case-fatality rate; and (3) hemorrhagic fevers, also frequently severe and fatal. These categories are somewhat arbitrary, and some arboviruses may be associated with more than one syndrome (eg, dengue).
The degree of viral multiplication and its predominant site of localization in tissues determine the clinical syndrome. Thus, individual arboviruses can produce a minor febrile illness in some patients and encephalitis or a hemorrhagic diathesis in others.
Arbovirus infections occur in distinct geographic distributions and vector patterns (Figure 38-2). Each continent tends to have its own arbovirus pattern, and names are usually suggestive, including Venezuelan equine encephalitis, Japanese B encephalitis, Murray Valley (Australia) encephalitis. Many encephalitides are alphavirus and flavivirus infections spread by mosquitoes, although the group of California encephalitis diseases is caused by bunyaviruses. On a given continent, there may be a shifting distribution depending on viral hosts and vectors in a given year.
FIGURE 38-2
Known distributions of flaviviruses causing human disease. A: Yellow fever virus. B: Dengue virus. C: St. Louis encephalitis virus. D: Japanese B encephalitis virus. E: Murray Valley encephalitis virus. F: Tick-borne encephalitis virus. G: West Nile virus. (Reproduced with permission from Monath TP, Tsai TF: Flaviviruses. In Richman DD, Whitley RJ, Hayden FG [editors]. Clinical Virology, 2nd ed. Washington DC: ASM Press, 2002. ©2002 American Society for Microbiology. No further reproduction or distribution is permitted without the prior written permission of American Society for Microbiology.)
Several arboviruses cause significant human infections in the United States (Table 38-2). The numbers of cases vary widely from year to year.
Diseasea | Exposure | Distribution | Major Vectors | Infection:Case Ratio (Age Incidence) | Sequelaeb | Mortality Rate (%) |
---|---|---|---|---|---|---|
Eastern equine encephalitis (Alphavirus) | Rural | Atlantic, southern coastal | Aedes, Culex | 10:1 (infants) 50:1 (middle-aged) 20:1 (elderly) | + | 30–70 |
Western equine encephalitis (Alphavirus) | Rural | Pacific, Mountain, Southwest | Culex tarsalis, Aedes | 50:1 (<5 years) 1000:1 (>15 years) | + | 3–7 |
Venezuelan equine encephalitis (Alphavirus) | Rural | South (also South and Central America) | Aedes, Psorophora, Culex | 25:1 (<15 years) 1000:1 (>15 years) | ± | Fatalities rare |
St. Louis encephalitis (Flavivirus) | Urban–rural | Widespread | Culex | 800:1 (<9 years) 400:1 (9–59 years) 85:1 (>60 years) | ± | 3–10 (<65 years) 30 (>65 years) |
West Nile fever (Flavivirus) | Urban–rural | Widespread | Culex, Aedes, Anopheles | 5:1 (fever) 150:1 (encephalitis) | ± | 3–15 |
California encephalitis (La Crosse) (Orthobunyavirus) | Rural | North central, Atlantic, South | Aedes triseriatus | Unknown ratio (most cases <20 years) | Rare | ~1 |
Hantavirus pulmonary syndrome (Hantavirus) | Rural | Southwest, West | Peromyscus maniculatusc | 15:1 | Rare | 30–40 |
Colorado tick fever (Coltivirus) | Rural | Pacific, Mountain | Dermacentor andersoni | Unknown ratio (all ages affected) | Rare | Fatalities rare |
In the Togaviridae family, the Alphavirus genus consists of about 30 viruses 70 nm in diameter that possess a single-stranded, positive-sense RNA genome (see Table 38-1). The envelope surrounding the particle contains two glycoproteins (see Figure 38-1). Alphaviruses often establish persistent infections in mosquitoes and are transmitted between vertebrates by mosquitoes or other blood-feeding arthropods. They have a worldwide distribution. All alphaviruses are antigenically related. The viruses are inactivated by acid pH, heat, lipid solvents, detergents, bleach, phenol, 70% alcohol, and formaldehyde. Most possess hemagglutinating ability. Rubella virus, classified in a separate genus in the Togaviridae family, has no arthropod vector and is not an arbovirus (see Chapter 40).
The Flaviviridae family consists of about 70 viruses 40–60 nm in diameter that have a single-stranded, positive-sense RNA genome. Initially, the flaviviruses were included in the togavirus family as “group B arboviruses” but were moved to a separate family because of differences in viral genome organization. The viral envelope contains two glycoproteins. Some flaviviruses are transmitted among vertebrates by mosquitoes and ticks, but others are transmitted among rodents or bats without any known insect vectors. Many have worldwide distribution. All flaviviruses are antigenically related. Flaviviruses are inactivated similarly to alphaviruses, and many also exhibit hemagglutinating ability. Hepatitis C virus, classified in a separate genus in the Flaviviridae family, has no arthropod vector and is not an arbovirus (see Chapter 35).
The alphavirus RNA genome is positive sense (Figure 38-3). Genomic length and subgenomic (26S) mRNAs are produced during transcription. The genomic-length transcript produces a precursor polyprotein encoding the nonstructural proteins (ie, replicase, transcriptase) needed for viral RNA replication. The subgenomic mRNA encodes structural proteins. The proteins are elaborated by posttranslational cleavage. Alphaviruses replicate in the cytoplasm and mature by budding nucleocapsids through the plasma membrane. Sequence data indicate that western equine encephalitis virus is a genetic recombinant of eastern equine encephalitis and Sindbis viruses.
FIGURE 38-3
Genomic organization of alphaviruses. The nonstructural proteins (nsP) are translated from the genomic RNA as a polyprotein that is processed into four nonstructural proteins by a viral protease present in nsP2. The structural proteins are translated from a subgenomic 26S mRNA as a polyprotein that is processed by a combination of viral and cellular proteases into a capsid protein (C), three envelope glycoproteins (E3, E2, and E1), and a membrane-associated protein named 6K. C, E2, and E1 are major components of virions and are shaded in the figure. (Reproduced with permission from Strauss JH, Strauss EG, Kuhn RJ: Budding of alphaviruses. Trends Microbiol 1995;3:346.)
The flavivirus RNA genome also is positive sense. A large precursor protein is produced from genome-length mRNAs during viral replication; it is cleaved by viral and host proteases to yield all the viral proteins, both structural and nonstructural. Flaviviruses replicate in the cytoplasm, and particle assembly occurs in intracellular vesicles (Figure 38-4). Proliferation of intracellular membranes is a characteristic of flavivirus-infected cells.
All alphaviruses are antigenically related. Because of common antigenic determinants, the viruses show cross-reactions in immunodiagnostic techniques. Hemagglutination-inhibition (HI), enzyme-linked immunosorbent assay (ELISA), and immunofluorescence tests define eight antigenic complexes or serogroups of alphaviruses, four of which are typified by Western equine encephalitis, Eastern equine encephalitis, Venezuelan equine encephalitis, and Semliki Forest virus. Identification of a specific virus can be accomplished using neutralization tests. Similarly, all flaviviruses share antigenic sites. At least eight antigenic complexes have been identified based on neutralization tests for alphaviruses and 10 serocomplexes for flaviviruses. The envelope (E) protein is the viral hemagglutinin and contains the group-, serocomplex-, and type-specific determinants. Sequence comparisons of the E glycoprotein gene show that viruses within a serocomplex share over 70% amino acid sequences but amino acid homology across serocomplexes is less than 50%.
In susceptible vertebrate hosts, primary viral multiplication occurs either in myeloid and lymphoid cells or in vascular endothelium. Multiplication in the central nervous system depends on the ability of the virus to pass the blood–brain barrier and to infect nerve cells. In natural infection of birds and mammals, an inapparent infection is usual. For several days there is viremia, and arthropod vectors acquire the virus by sucking blood during this period—the first step in its dissemination to other hosts.
The disease in experimental animals provides insights into human disease. Mice have been used to study the pathogenesis of encephalitis. After subcutaneous inoculation, virus replication occurs in local tissues and regional lymph nodes. Virus then enters the bloodstream and is disseminated. Depending on the specific agent, different tissues support further virus replication, including monocyte-macrophages, endothelial cells, lung, liver, and muscles. Virus crosses the blood–brain barrier by unknown mechanisms, perhaps involving olfactory neurons or cerebral vascular cells, and spreads. Widespread neuronal degeneration occurs in all arbovirus-induced encephalitides.
In the vast majority of infections, the virus is controlled before neuroinvasion occurs. Invasion depends on many factors, including the level of viremia, the genetic background of the host, the host innate and adaptive immune responses, and the virulence of the virus strain. Humans show an age-dependent susceptibility to central nervous system infections, with infants and elderly adults being most susceptible.
The equine encephalitides in horses are diphasic. In the first phase (minor illness), the virus multiplies in non-neural tissue and is present in the blood several days before the first signs of involvement of the central nervous system. In the second phase (major illness), the virus multiplies in the brain, cells are injured and destroyed, and encephalitis becomes clinically apparent. High concentrations of virus in brain tissue are necessary before the clinical disease becomes manifest.
Incubation periods of the encephalitides are between 4 and 21 days. Inapparent infections are common. Some infected persons develop mild flu-like illness, and others develop encephalitis. There is a sudden onset with severe headache, chills and fever, nausea and vomiting, generalized pains, and malaise. Within 24–48 hours, marked drowsiness develops, and the patient may become stuporous. Mental confusion, tremors, convulsions, and coma develop in severe cases. Fever lasts 4–10 days. The mortality rate in encephalitides varies (see Table 38-2). With Japanese B encephalitis, the mortality rate in older age groups may be as high as 80%. Sequelae may be mild to severe and include mental deterioration, personality changes, paralysis, aphasia, and cerebellar signs.
Virus isolation attempts require appropriate biosafety precautions to prevent laboratory infections. Virus occurs in the blood only early in the infection, usually before the onset of symptoms. Virus can also be found in cerebrospinal fluid and tissue specimens, depending on the agent. Alphaviruses and flaviviruses are usually able to grow in common cell lines, such as Vero, BHK, HeLa, and MRC-5. Mosquito cell lines are useful. Intracerebral inoculation of suckling mice or hamsters may also be used for virus isolation.
Antigen detection and polymerase chain reaction (PCR) assays are available for direct detection of viral RNA or proteins in clinical specimens for some arboviruses. The use of virus-specific monoclonal antibodies in immunofluorescence assays has facilitated rapid virus identification in clinical samples.
Neutralizing and hemagglutination-inhibiting antibodies are detectable within a few days after the onset of illness. The neutralizing and the hemagglutination-inhibiting antibodies endure for years. The HI test is the simplest diagnostic test, but it identifies the group rather than the specific causative virus. The most sensitive serologic assays detect virus-specific immunoglobulin M (IgM) in serum or cerebrospinal fluid by ELISA.
It is necessary to establish a fourfold or greater rise in specific antibodies during infection to confirm a diagnosis. The first sample of serum should be taken as soon after the onset as possible and the second sample 2–3 weeks later. The cross-reactivity within the alphavirus or flavivirus group must be considered in making the diagnosis. After a single infection by one member of the group, antibodies to other members may also appear. Serologic diagnosis becomes difficult when an epidemic caused by one member of the serologic group occurs in an area where another group member is endemic.
Immunity is believed to be permanent after a single infection. Both humoral antibody and cellular immune responses are thought to be important in protection and recovery from infection. In endemic areas, the population may build up immunity as a result of inapparent infections; the proportion of persons with antibodies to the local arthropod-borne virus increases with age.
Because of common antigens, the response to immunization or to infection with one of the viruses of a group may be modified by prior exposure to another member of the same group. This mechanism may be important in conferring protection on a community against an epidemic of another related agent (eg, no Japanese B encephalitis in areas endemic for West Nile fever).
In highly endemic areas, almost the entire human population may become infected with an arbovirus, and most infections are asymptomatic. High infection-to-case ratios exist among specified age groups for many arbovirus infections (see Table 38-2). Most cases occur in the summer months in the northern hemisphere when arthropods are most active.
Eastern equine encephalitis is the most severe of the arboviral encephalitides, with the highest case-fatality rate. Infections are rare and sporadic in the United States, averaging five confirmed cases per year. In the case of Western equine encephalitis, transmission occurs at a low level in the rural West, where birds and Culex tarsalis mosquitoes are involved in the maintenance cycle of the virus. Infections of humans average about 15 confirmed cases annually. However, there have been instances in the past (most recently in 1987) when humans and equines became infected at epidemic and epizootic levels. Outbreaks have affected wide areas of the western United States and Canada.
St. Louis encephalitis virus is the most important cause of epidemic encephalitis of humans in North America (see Figure 38-2), having caused about 10,000 cases and 1000 deaths since it was first recognized in 1933. Seroprevalence rates are generally low, and the incidence of St. Louis encephalitis varies each year in the United States. There are currently an average of 100 confirmed cases annually. Fewer than 1% of viral infections are clinically apparent. The presence of infected mosquitoes is required before human infections can occur, although socioeconomic and cultural factors (air conditioning, screens, mosquito control) affect the degree of exposure of the population to these virus-carrying vectors.
West Nile fever is caused by a member of the Japanese B encephalitis antigenic complex of flaviviruses. It occurs in Europe, the Middle East, Africa, the former Soviet Union, Southwest Asia, and, more recently, the United States. It appeared unexpectedly in the New York City area in 1999, resulting in seven deaths and extensive mortality in a range of domestic and exotic birds. Sequence analysis of virus isolates showed that it originated in the Middle East; it probably crossed the Atlantic in an infected bird, mosquito, or human traveler.
Within 3 years, West Nile virus had completed transcontinental movement across the United States and was established as a permanent presence in temperate North America. West Nile virus has been detected in all 48 contiguous states. It is now the leading cause of arboviral encephalitis in the United States. Other arboviruses that cause sporadic cases of neuroinvasive disease in the United States include La Crosse virus and Eastern and St. Louis encephalitis viruses. It is estimated that about 80% of West Nile infections are asymptomatic, with about 20% causing West Nile fever and less than 1% causing neuroinvasive disease (meningitis, encephalitis, or acute flaccid paralysis). Fatal encephalitis is more common in older people. A genetic deficiency resulting in a nonfunctional variant of chemokine receptor CCR5 has been identified as a risk factor for symptomatic West Nile infections. A West Nile virus epidemic in 2002 in the United States included the first documented cases of person-to-person transmission through organ transplantation, blood transfusion, in utero, and perhaps breastfeeding. Screening of blood donations for West Nile virus was implemented in the United States in 2003.
West Nile virus produces viremia and an acute, mild febrile disease with lymphadenopathy and rash. Transitory meningeal involvement may occur during the acute stage. Only one antigenic type of virus exists, and immunity is presumably permanent.
A West Nile vaccine for horses became available in 2003. There is no human vaccine. Prevention of West Nile virus disease depends on mosquito control and protection against mosquito bites.
Japanese B encephalitis is the leading cause of viral encephalitis in Asia (see Figure 38-2). About 50,000 cases occur annually in China, Japan, Korea, and the Indian subcontinent, with 10,000 deaths, mostly among children and elderly adults. The mortality rate can exceed 30%. A high percentage of survivors (up to 50%) are left with neurologic and psychiatric sequelae. Infections during the first and second trimesters of pregnancy have reportedly led to fetal death.
Seroprevalence studies indicate nearly universal exposure to Japanese B encephalitis virus by adulthood. The estimated ratio of asymptomatic to symptomatic infections is 300 to one. There is no treatment. Several effective Japanese encephalitis vaccines are available in Asia. An inactivated Vero cell culture-derived vaccine was licensed in the United States in 2009.
This mosquito-borne alphavirus is a member of the Semliki Forest antigenic complex. It reemerged in Kenya in 2004 after an absence of several decades and subsequently caused massive outbreaks of infection in India, southeastern Asia, and the Indian Ocean region. The virus caused an outbreak in Italy in 2007. Occasionally cases are reported in returning travelers in the United States. In 2013, chikungunya virus became established in the Caribbean region, and is likely to spread rapidly. Clinically, the infection resembles dengue fever, but is more likely to cause high fever, rash, and severe joint pain; asymptomatic infections are rare. There is no vaccine available.
This flavivirus is an important cause of encephalitis in Europe, Russia, and northern China. About 10,000–12,000 cases of tick-borne encephalitis are reported each year, with the most cases occurring in the Baltic states, Slovenia, and Russia. The disease occurs chiefly in the early summer, particularly in humans exposed to the ticks Ixodes persulcatus and Ixodes ricinus in forested areas during outdoor activities. Three subtypes of virus cause human disease: the European, Far-Eastern, and Siberian subtypes, with the Far-Eastern variant appearing to be the most virulent. Many species of animals can be infected by the virus; person-to-person transmission has not been reported.
There is no specific treatment for tick-borne encephalitis. Personal protective measures, such as wearing appropriate clothing, can help reduce the risk of exposure. Effective vaccines, produced in Austria, Germany, and Russia, are available that are based on the European and Far-Eastern strains of the virus.
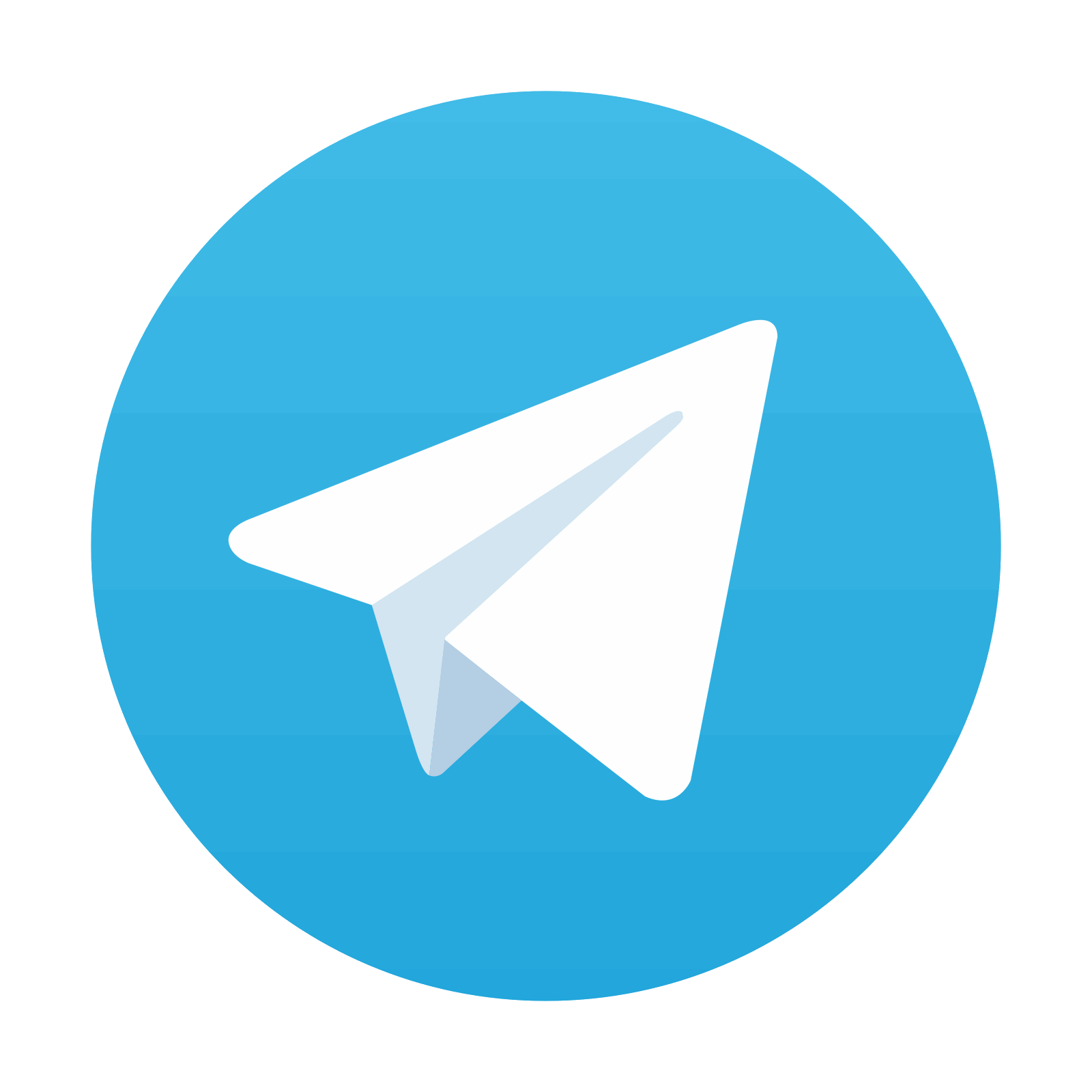
Stay updated, free articles. Join our Telegram channel

Full access? Get Clinical Tree
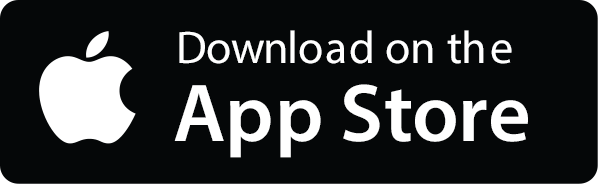
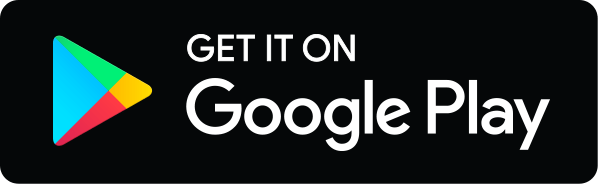