Approach to Patients with Chronic Kidney Disease, Stages 1-4
Ajay Singh
Chronic kidney disease (CKD) can be defined in a variety of ways. The US Preventive Health Service defines it as decreased kidney function, with size-adjusted estimated glomerular filtration rate (eGFR/1.73 m2) <60 mL/min, or as kidney damage that persists for at least 3 months.
The management of a patient with CKD involves the following considerations: screening, etiologic diagnosis, and staging of the CKD severity; identifying and managing patients at high risk of progression; management of complications of CKD; and preparing the patient for transplantation or renal replacement therapy.
I. SCREENING, DIAGNOSIS, AND STAGING. Screening should include monitoring for the presence of proteinuria and measurement of kidney function. Screening should focus on patients with CKD risk factors. These include diabetes mellitus, hypertension, cardiovascular disease, history of smoking, obesity, age >60 years, indigenous racial origin, and a family history of CKD.
A. Urinary protein measurement. The US Preventive Health Service recommends urinary protein measurement as a screening test in all high-risk individuals. The American Diabetes Association (ADA) recommends that an evaluation for microalbuminuria be performed in all type 2 diabetic patients at the time of diagnosis and in all type 1 diabetic patients 5 years after initial evaluation. Screening can be done by urine dipstick, but a more reliable method is an early morning measurement of the albumin-to-creatinine ratio in a spot urine sample. The dipstick used should be able to detect both albumin and evidence of blood or white cells. If the dipstick test suggests either blood or white cell activity, then a microscopic analysis of the urinary sediment should be performed. Table 1.1 lists several limitations of urine dipstick evaluation. One problem with urine dipstick tests is that they measure concentration only, and can give falsely negative results in a dilute urine. The urine albumin-to-creatinine ratio (UACR) overcomes this problem by looking at the ratio of albumin to creatinine, as both will be affected by dilution, and the effects of dilution will
tend to cancel out. In terms of milligrams albumin per gram or millimole of creatinine, normoalbuminuria is defined as <30 mg/g (<3 mg/mmol); microalbuminuria as 30-300 mg/g (3-30 mg/mmol); and macroalbuminuria as >300 mg/g (>30 mg/mmol). These cutoffs correspond only roughly to albuminuria measured in terms of milligrams per day (e.g., 30 and 300 mg per day), and they assume that 1 g of creatinine is being excreted per day. In fact, the average amount of creatinine excreted per day is actually higher, and as discussed elsewhere in this chapter, creatinine excretion is greater in men than in women and in young people versus older people. However, fine-tuning these “cutoff” UACR ratios is not of great clinical importance, as the risk of increased urine albumin excretion is continuous, and risk is increased even when the albumin excretion is <30 mg per day. The UACR can be done at any time, but a morning test may increase sensitivity and will tend to exclude the relatively benign condition of orthostatic proteinuria, where, proteinuria is present during the day, but ceases while the subject is sleeping supine. A positive UACR test should be repeated at least twice over 3 months to exclude acute kidney injury and to confirm a positive test.
tend to cancel out. In terms of milligrams albumin per gram or millimole of creatinine, normoalbuminuria is defined as <30 mg/g (<3 mg/mmol); microalbuminuria as 30-300 mg/g (3-30 mg/mmol); and macroalbuminuria as >300 mg/g (>30 mg/mmol). These cutoffs correspond only roughly to albuminuria measured in terms of milligrams per day (e.g., 30 and 300 mg per day), and they assume that 1 g of creatinine is being excreted per day. In fact, the average amount of creatinine excreted per day is actually higher, and as discussed elsewhere in this chapter, creatinine excretion is greater in men than in women and in young people versus older people. However, fine-tuning these “cutoff” UACR ratios is not of great clinical importance, as the risk of increased urine albumin excretion is continuous, and risk is increased even when the albumin excretion is <30 mg per day. The UACR can be done at any time, but a morning test may increase sensitivity and will tend to exclude the relatively benign condition of orthostatic proteinuria, where, proteinuria is present during the day, but ceases while the subject is sleeping supine. A positive UACR test should be repeated at least twice over 3 months to exclude acute kidney injury and to confirm a positive test.
TABLE 1.1 Limitations of Urine Dipstick | |||||||||||
---|---|---|---|---|---|---|---|---|---|---|---|
|
B. Measurement of kidney function
1. Glomerular filtration rate (GFR). The GFR, usually expressed in terms of milliliters per minute, is the volume of serum cleared by the kidneys per unit of time. The GFR depends on body size and age, and so an isolated value of GFR needs to be evaluated in context. One usually normalizes GFR to body surface area, specifically, per 1.73 m2. In healthy subjects, GFR/1.73 m2 is similar in men and women, but GFR/1.73 m2 declines with age, averaging about 115 mL/min in young adults, 100 mL/min in the middle-aged, and then dwindling to 90, 80, and 70 mL/min as patient age increases to 60, 70, and 80 years, respectively.
2. Serum creatinine. Creatinine is produced at a relatively constant rate from creatine in muscle and is excreted by the kidneys by both glomerular filtration and tubular secretion. Normal creatinine concentrations range from 0.6 to 1.0 mg/dL (53-88 mcmol/L) in women and from 0.8 to 1.3 mg/dL (70-115 mcmol/L) in men. Measurement of the serum creatinine concentration is one way to obtain a rough estimate of the level of renal function, because as renal function falls, creatinine will continue to be produced, and the serum levels will rise. The relationship between serum creatinine and renal function is nonlinear: a doubling of the serum creatinine will reflect a decline in GFR of approximately 50%. Doubling of serum creatinine from an initially low value can result in a serum creatinine still in the “normal range” despite a substantial loss of kidney function. Serum creatinine levels are influenced by muscle mass, recent dietary intake, especially of cooked meat, and concomitant drug therapy (e.g., treatment with cimetidine, a drug that blocks tubular secretion of creatinine and which will slightly increase the serum creatinine without any effect on GFR). In patients with cirrhosis and ascites, estimation of renal function from serum creatinine is particularly difficult. There can be a very low creatinine production rate due to extremely low muscle mass (low creatinine production rate), plus it often is difficult to determine ascites-free body weight for normalization. In such patients, serum creatinine levels in the 0.5-1.0-mg/dL range (44-88 mcmol/L), nominally “normal,” may reflect moderately to markedly impaired levels of renal function. Even in patients without cachexia, the serum creatinine level must always be interpreted in the context of a patient’s muscle mass. For example, a serum creatinine of 1.3 mg/dL (115 mcmol/L) can represent a creatinine clearance of 94 mL/min in a young 80-kg male, or a creatinine clearance of only 28 mL/min in an elderly, 50-kg female (Macgregor and Methven, 2011).
Until recently the serum creatinine was measured by a variety of methods, some of which, due to interfering substances in the blood, deviated substantially from “true” values of creatinine concentration as determined by isotope dilution mass spectrometry (IDMS). In the United States and many other countries, laboratories are now normalizing their measurement methods to IDMS, and the normalized values tend to be lower than those obtained using other methods.
3. Creatinine clearance by timed urine collection. A timed (usually 24 hours) urine collection of creatinine excretion can be used to calculate the creatinine clearance (CCr), which is defined as the volume of serum cleared of creatinine per minute. Normal CCr is approximately 95 ± 20 mL/min in average-size adult women and 125 ± 25 mL/min in average-size adult men. Patients are instructed to urinate
into the toilet on arising, and to mark this time as the start of the collection period. Next they are to pass all of their urine into a container during the ensuing day and night. The following morning, the patients are to urinate into the container one last time, and to note this time as the end of the collection period. By dividing the amount of creatinine in the collected urine by the number of minutes in the collection period (start time to finish time), the laboratory can calculate the per minute rate of creatinine excretion. A sample of blood must be drawn at some point during the urine collection period in which the serum creatinine level is measured. To calculate creatinine clearance, one simply divides the per minute creatinine excretion rate by the serum value. This gives the volume per minute of serum that had to have been “cleared” of creatinine by the kidneys. For example, if the per minute creatinine excretion rate is 1.0 mg/min, and the serum creatinine level is 1 mg/dL, or 0.01 mg/mL, then 1.0/0.01 = 100 mL/min of serum were on average being cleared of creatinine by the kidneys during the collection period. Despite the technical challenge of collecting urine properly, timed urine collections are a very useful means of estimating kidney function in cachectic patients, including those with cirrhosis and ascites, as well as markedly obese patients. The completeness of the urine collection for creatinine can be estimated by comparing the amount of creatinine recovered per day based on the expected daily creatinine excretion rate for a given patient based on sex and body weight. Thus, one expects daily creatinine excretion to be about 15-20 mg/kg lean body weight in women and 20-25 mg/kg lean body weight in men. A more exact estimate of daily creatinine excretion rate can be obtained from the use of an equation incorporating body weight, gender, age, and race, such as that developed by Ix (2011), and which is detailed as a nomogram in Appendix A. A creatinine excretion rate that is significantly less than expected usually indicates an incomplete urine collection.
into the toilet on arising, and to mark this time as the start of the collection period. Next they are to pass all of their urine into a container during the ensuing day and night. The following morning, the patients are to urinate into the container one last time, and to note this time as the end of the collection period. By dividing the amount of creatinine in the collected urine by the number of minutes in the collection period (start time to finish time), the laboratory can calculate the per minute rate of creatinine excretion. A sample of blood must be drawn at some point during the urine collection period in which the serum creatinine level is measured. To calculate creatinine clearance, one simply divides the per minute creatinine excretion rate by the serum value. This gives the volume per minute of serum that had to have been “cleared” of creatinine by the kidneys. For example, if the per minute creatinine excretion rate is 1.0 mg/min, and the serum creatinine level is 1 mg/dL, or 0.01 mg/mL, then 1.0/0.01 = 100 mL/min of serum were on average being cleared of creatinine by the kidneys during the collection period. Despite the technical challenge of collecting urine properly, timed urine collections are a very useful means of estimating kidney function in cachectic patients, including those with cirrhosis and ascites, as well as markedly obese patients. The completeness of the urine collection for creatinine can be estimated by comparing the amount of creatinine recovered per day based on the expected daily creatinine excretion rate for a given patient based on sex and body weight. Thus, one expects daily creatinine excretion to be about 15-20 mg/kg lean body weight in women and 20-25 mg/kg lean body weight in men. A more exact estimate of daily creatinine excretion rate can be obtained from the use of an equation incorporating body weight, gender, age, and race, such as that developed by Ix (2011), and which is detailed as a nomogram in Appendix A. A creatinine excretion rate that is significantly less than expected usually indicates an incomplete urine collection.
Because creatinine is cleared by the renal tubules in addition to being filtered at the glomerulus, the creatinine clearance is greater than GFR. When GFR/1.73 m2 is very low (e.g., less than 10-15 mL/min), the proportion of creatinine excretion due to tubular secretion is high. To get a more reliable estimate of GFR when GFR is low, one can measure the amounts of both creatinine and urea in the timed urine sample, and measure the serum urea level as well as the creatinine level during the collection period. The per minute clearance of urea is calculated in the same way as for creatinine. Urea is filtered at the glomerulus, but then some urea is reabsorbed by the renal tubules, so with urea, the situation is opposite to that with creatinine; due to tubular reabsorption, the urea clearance will be less than
the GFR, whereas the creatinine clearance will be greater than the GFR. Averaging the urea and creatinine clearances has been shown to give a good estimate of GFR in patients with GFR less than 10-15 mL/min.
the GFR, whereas the creatinine clearance will be greater than the GFR. Averaging the urea and creatinine clearances has been shown to give a good estimate of GFR in patients with GFR less than 10-15 mL/min.
4. Estimated creatinine clearance. To avoid the inaccuracies and inconvenience of timed urine collections, creatinine clearance (CCr) can be estimated by using equations that estimate the per minute creatinine excretion rate based on age, body size, gender, and in some equations, race. One equation that has been used for this is the Cockcroft-Gault equation:
Estimated CCr = (140 – Age) × (0.85 if female) × (W in kg)/(72 × SCr in mg/dL)
or
Estimated CCr = (140 – Age) × (0.85 if female) × (W in kg)/(0.814 × SCr in mcmol/L)
where W is body weight. This equation provides a quick and reasonably accurate estimate of renal function at the bedside. The more recently developed Ix equation (Ix, 2011), described in Appendix A, also can be used. The Ix equation was developed and validated in a much larger sample of individuals, including blacks, and was based on modern, IDMS-calibrated laboratory measures of creatinine. Neither equation is very accurate in markedly obese or cachectic patients. Some have suggested that the accuracy of the Cockcroft-Gault equation can be increased by using actual body weight for cachectic patients, ideal body weight for normal weight patients, and adjusted body weight for markedly obese patients (Brown, 2013). See Appendix B for more details.
5. Estimated GFR
a. Modification of Diet in Renal Disease (MDRD) equation. This equation was derived from the MDRD trial and reports eGFR normalized per 1.73 m2 of body surface area. For laboratories using the new IDMS-standardized serum creatinine values, the version of the MDRD equation that should be used is what follows:
eGFR/1.73 m2 = 175 × [SCr] – 1.154 × [Age] – 0.203 × [0.742 if patient is female] × [1.210 if patient is black].
The “175” term in this equation replaces the “186” term in the original published equation to account for the slightly lower values of IDMS-standardized creatinine assays compared to assays used in the MDRD study. When serum creatinine is measured in SI units (mcmol/L), one needs to divide the serum creatinine value by 88.5 to convert to mg/dL prior to inserting into the equation.
The MDRD GFR equation differs from the Cockcroft-Gault or Ix estimates of the creatinine clearance in several ways. First, it was developed from data that measured GFR by iothalamate, a substance which is not secreted by the renal tubules, and so it predicts GFR rather than creatinine clearance. All else being equal, the MDRD equation will give a lower value for renal function (GFR) than creatinine clearance, which includes the tubular secretion component of renal function. Secondly, the MDRD equation is normalized to body size and is expressed as eGFR/1.73 m2 of body surface area. Creatinine clearance, whether obtained from a timed urine sample or from the Ix or Cockcroft-Gault equation, is raw renal creatinine clearance that has not been adjusted for body size.
b. The CKD-EPI GFR equation. This is similar to the MDRD equation, but this newer equation was validated in a larger group of subjects, particularly those with only mild degrees of renal impairment. The CKD-EPI equation is listed in Appendix A. The differences between the two equations are usually not of clinical importance, as they occur primarily in patients with GFR levels greater than 60, where the impact of knowing the precise level of renal function is not particularly large.
c. Cystatin C equations. An alternative method of estimating GFR is based on equations that use the serum cystatin C level. Cystatin C is a 13-kDa protein produced by all cells that is filtered by the glomerulus and not reabsorbed. The production rate of cystatin C is not related to muscle mass or dietary meat intake, and cystatin C-based estimates of GFR correlate better with CKD-related outcomes than creatinine-based equations in some studies. Some of the newest efforts to predict GFR combine both serum creatinine and cystatin C levels (Levey, 2014). Laboratory methods of measuring cystatin C are not commonly standardized (this is in progress, similar to IDMS standardization of creatinine), and for the moment, cystatin C equations are not in wide use.
6. Problems with estimated clearances in acute kidney injury. The estimating equations based on either creatinine or cystatin are based on steady-state assumptions. If one were to surgically remove both kidneys, the serum creatinine or cystatin C levels would begin to rise, but this would take place over a number of days as opposed to immediately. For this reason, none of the renal function estimating equations described above are useful in situations where the level of kidney function is rapidly changing. The timed urine collection method can be used to measure creatinine clearance, but then serum creatinine levels need to be measured at both the beginning and end of the collection period, and the per minute excretion rate should be divided by the time-averaged serum value in the calculations.
C. Ultrasound and serum electrolytes. In patients found to have CKD, one should image the kidneys, commonly by ultrasound, to look for structural abnormalities and possible obstruction and measure serum electrolytes (Na, K, Cl, HCO3) to screen for metabolic acidosis and electrolyte disorders, the presence of which may give clues to an underlying renal disease.
D. Looking for an etiologic diagnosis. Identifying the underlying cause of CKD is important. The CKD may be reversible, for example in a patient with bilateral renovascular disease or chronic bladder neck obstruction from prostatic hypertrophy. The cause of CKD may provide insights into the tempo of disease progression. Since some etiologies of kidney disease are very likely to recur in a future kidney allograft, identifying the underlying cause of CKD at the outset may help in later management decisions.
E. Staging. The National Kidney Foundation’s (NKF) Kidney Disease Outcome Quality Initiative’s (KDOQI) staging of CKD has been widely adopted. It stages CKD from stage 1 (mildest) to stage 5 (most severe) based on the level of eGFR normalized to body surface area. The two mildest stages—stages 1 and 2, in which the eGFR/1.73 m2 is still above 60 mL/min—require evidence for kidney damage apart from reduced GFR. Kidney damage can be manifest as pathologic changes on kidney biopsy; abnormalities in the composition of the blood or urine (proteinuria or changes in the urine sediment examination), or abnormalities in imaging tests. The more severe stages of CKD—stages 3, 4, and 5—are present by definition when the GFR is below 60, 30, and 15, respectively. Some elderly patients with eGFR/1.73 m2 in the range of 45-60 mL/min may not have obvious kidney damage, nor an increased risk of an accelerated decline in renal function or mortality. A subsequent staging system developed by KDIGO (Kidney Disease: Improving Global Outcomes) partially takes this into account by subdividing stage 3 CKD into two levels: 3a, with eGFR/1.73 m2 in the range of 45-59 mL/min; and 3b, with GFR levels between 30 and 44 mL/min. Also, the newer staging system adds in the degree of proteinuria as measured by the UACR. One newer staging system is shown in Table 1.2, where a low risk of CKD progression and complications is indicated by “green,” and progressively increased risk is indicated by “yellow,” “orange,” and “red.”
II. SLOWING PROGRESSION OF CKD AND OF CARDIOVASCULAR DISEASE. In CKD patients, risk factors for progression of renal disease are very similar to those associated with increased cardiovascular risk. One purpose of identifying CKD patients early on is to attempt to correct and/or mitigate such risk factors, in the hopes of both maintaining GFR and minimizing cardiovascular risk. The main risk factors include smoking, high blood pressure, hyperglycemia in diabetic patients (and perhaps in nondiabetic persons as well), elevated blood lipid levels, anemia, and elevated serum
phosphorus levels. Urinary protein excretion and even microalbuminuria markedly increase both the risk of progression and cardiovascular complications. Levels of inflammatory mediators, notably C-reactive protein (CRP), are increased in CKD and are associated with increased atherosclerotic risk.
phosphorus levels. Urinary protein excretion and even microalbuminuria markedly increase both the risk of progression and cardiovascular complications. Levels of inflammatory mediators, notably C-reactive protein (CRP), are increased in CKD and are associated with increased atherosclerotic risk.
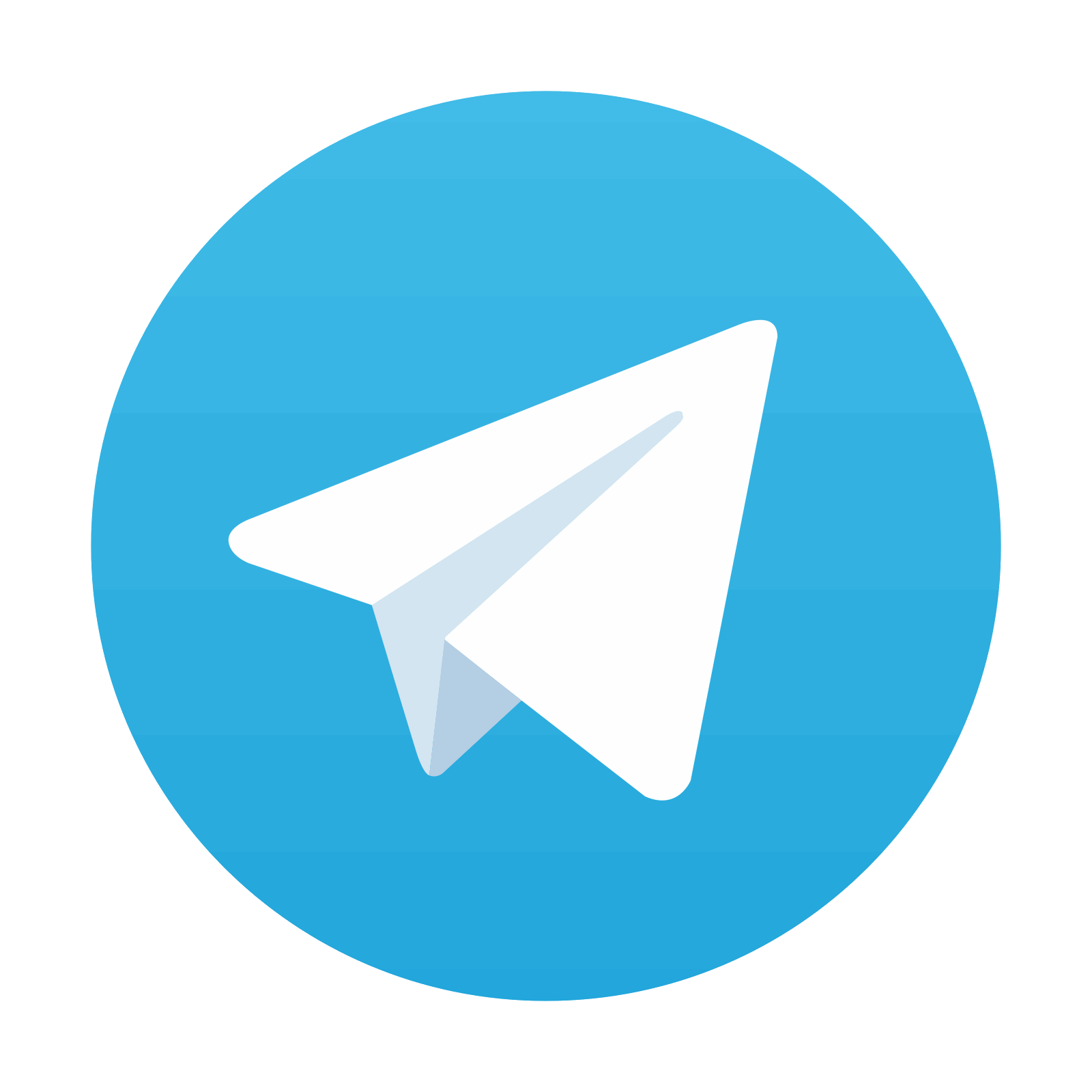
Stay updated, free articles. Join our Telegram channel

Full access? Get Clinical Tree
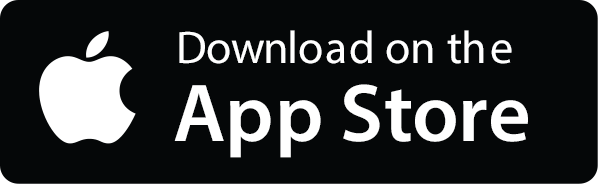
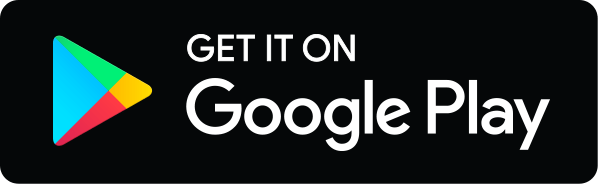